Abstract
Salmon slurry containing C. sporogenes spores was subjected to high pressure (HP) treatments (700–900 MPa; 80–100°C, and 0–24 min). Destruction rates (D value) and pressure/temperature sensitivity parameters (ZP and ZT ) were evaluated. Thermal treatment D values were an order of magnitude higher than those under HP. Higher pressures and temperatures accelerated the spore destruction rates. ZP values were 14.5, 17.3 and 15.5°C at 700, 800 and 900 MPa respectively, while ZT values (at constant temperature) were 440, 540, 550 MPa at 80, 90, and 100°C, respectively. The z value under thermal treatment was 8.8°C. The spores were relatively more sensitive to temperature than to pressure.
INTRODUCTION
High-pressure processing as a novel non-thermal food processing technique has been widely applied for pasteurization of foods to extend food shelf life and reserve high qualities, such as natural color, flavor, and nutrients.[Citation1–6 However, microbial spores and some enzymes can survive the pasteurization. Therefore, high pressure treated foods should be kept at low temperature to prevent spoilage. Seafood products are the table favorites for their delicacy due to their specific flavor, texture, and rich of nutrients. They are low in acid, highly perishable and usually spoil faster than other muscle foods. Currently, high pressure processing is recognized as a value added pasteurization process for seafood processing. HP treated seafood, such as oyster, mussel and lobster, are currently available in the global food markets.[Citation7] However, this technique has not been used for low acid food sterilization because the more resistant bacterial spores are not adequately inactivated by HP applications at room or refrigerated temperatures.[Citation3,Citation8]
Although the potential for the use of HP treatment as an alternative method to thermal process is possible, commercial potential high pressure sterilization technology has not yet been fully realized because of the lack of data on high pressure destruction kinetics of microbial spores. Sterile shelf-stable seafood products are currently produced by thermal processing under conditions designed for low acid canned foods (LACF) respecting a minimum 12 log cycle reduction of the most heat resistant pathogen Clostridium botulinum.[Citation9] Recent studies demonstrate that high pressure combined with high temperature (HPHT) can inactivate many pressure resistant spores and enzymes.[Citation10–19 Adiabatic temperature change associated with pressure processing make them ideal for achieving rapid heating to processing temperatures and subsequent rapid cooling. These HPHT techniques would benefit the product in terms of retaining higher quality in the product. Such advantages have been demonstrated in some studies.[Citation20–22
Majority of HP studies on fish and seafood products have been carried out at lower pressures with the objective of controlling vegetative pathogens and spoilage bacteria with subsequent storage at refrigerated conditions or for accomplishing other objectives like improvement in sensory properties, assisting in other processes such as freezing and thawing.[Citation23–30 There have been some studies dealing with high-pressure inactivation of bacterial spores at elevated temperatures; however, detailed data on destruction kinetics gathered at near isobaric and isothermal conditions is still limited.[Citation21] Recently, Koutchma et al.[Citation13] carried out inactivation kinetics of C. sporogenes (PA3679) spores in buffer with engineering improvement by using a internal heater to maintain pressure chamber temperatures. Shao et al.[Citation18] used a specially designed insulated chamber for carrying out near isothermal isobaric spore inactivation kinetics under high pressure processing conditions at elevated temperatures. Ahn et al.[Citation16] observed pressure-assisted thermal processing (PATP) at 700 MPa and 121°C for 1 min inactivated up to 7–8 log reduction for C. sporogenes spores. Ahn et al.[Citation17] reported that spore clumps formed during the PATP may lead to an increase in pressure-thermal resistance, and multiple-pulsed pressurization can be more effective in inactivating bacterial spores. Zhu et al.[Citation19] have documented the high pressure destruction kinetics of C. sporogenes spores in ground beef and indicated they could serve as possible surrogates for C. botulinum. Published information suggests that destruction kinetics of C. sporogenes likely depends on the nature of the food matrix in which they are present.
Seafood represents an important group of highly perishable low acid foods with typically high nutritive values. For the application of high pressure sterilization as a value added alternative processing technique, data relevant to destruction kinetics of bacterial spores is very important and necessary. The objective of this study was to evaluate the pressure destruction kinetics of C. sporogenes 7955 spore suspended in salmon slurry at high pressure high temperature processing conditions.
MATERIALS AND METHODS
Culture Preparation
Freeze-dried cultures of C. sporogenes (ATCC-7955) was obtained from the American Type Culture Collection (ATCC, Rockville, MD) and stored at –40°C. This culture was hydrated in 10 ml Reinforced Clostridial Medium (RCM) broth (Oxoid, Basingstoke, Hampshire, UK) at 37°C for 24 h in anaerobic condition, and then stored at 4°C. To prepare the inoculation, 0.1 ml of the cultured broth was transferred to 50 ml of fresh RCM broth and incubated at 37°C for 24 h in anaerobic condition. Two such transfers were made in succession to obtain the culture with viable counts of 108 CFU/ml, approximately.
An aliquote (0.2 ml) of C. sporogenes culture, prepared as detailed above, was transferred and spread on Campdem Sporulating Agar plate (CSA) and incubated at 37°C for seven days in an anaerobic condition for growing the bacterial spores. The CSA medium formula was 5 g tryptone (Oxoid, Basingstoke, Hampshire UK), 5 g bacterial peptone (BD, Co., Spark, MD), 1 g Lab Lemco meat extract (Oxoid, Basingstoke, Hampshire UK), 2 g yeast extract (BD, Co., Spark, MD), 0.056 g calcium chloride (BDH, Inc., Toronto, ON), 0.062 g MnSO4.H2O (Fisher Scientific, Fair Lawn, NJ), 1 g glucose (Fisher Scientific, Fairlawn, NJ) and 15 g bacterial agar (Fisher Scientific, Fairlawn, NJ) in 1 liter distilled water. Spores were collected by flooding the agar surface with 10 ml sterile distilled water and scraping the colonies with a sterile glass spreader. After harvest, the spores were washed three times and centrifuged at 4000 × g for 15 min each at 4°C, and suspended in sterile distilled water to give approximately 109 CFU/ml and stored at 4°C until use.
Salmon Sample Preparation
Fresh Atlantic salmon was obtained locally (La Mer, Montreal, QC) with skin and bone removed. The salmon fillet (68.6% moisture and pH 6.32) was cut into small cubes (1×1×1 cm), about 100 g sample weighed in to sterile stomacher plastic bags, sealed, quickly frozen and kept in a freezer (at −40°C) until use. Prior to preparation, the salmon fillet pieces was thawed overnight at 4°C, mixed with 100 g pre-adjusted spore suspension and then blended for 3 min in a stomacher (Bagmixer 400, St Nom, France) to get the 50% salmon slurry with initial spore counts of about 106CFU/g. Test sample bags were prepared by placing approximately 12 g spore slurry into each sterile plastic pouches (Nasco, Toronto, ON). Each sample was squeezed flat into a thin plate shape (60-mm length, 40-mm width, and 3-mm thickness) and heat sealed (National instrument Co., Baltimore, MD) after removing most of the trapped air. All sample bags were kept at 4°C refrigerator until use within 12 h. All the operations above were carried out in a Class 100 Forma laminar airflow workstation (Thermo Electron Co., Mariette, Ohio).
High Pressure Equipment
A vertical iso-static press (Model ACIP9000/1.7/8.5VB, ACB Corp., Nantes, France) with a pressure vessel (8.5 cm diameter and 30 cm high, 1.7 L capacity and 900 MPa pressure limit) was used for giving the HP treatment. A high capacity water bath (FP45, Julabo Labortechnik GMBH, Germany) was used to circulate temperature controlled hot water around the pressure vessel shell in order to regulate the shell temperature. The equipment was rated for operating at a maximum pressure of 900 MPa and vessel shell temperature of 80°C, although the internal fluid temperature could go much higher as a result of compression heating during pressurization. A data-logger (Agilent 34970A, formerly HP, Agilent Technologies Canada Inc., Mississauga, ON) connected K-type thermocouple (OMEGA Engineering, Stamford, CT) which went though high pressure chamber cover, was used to collect online pressure and temperatures data every 1 s. The pressurization medium was pure glycerin G33-20 (Fisher Scientific, Whitby, ON, Canada) to meet the requirement of its pump.
High Pressure Treatment
A thick wall POM (polyoxymethylene, Johnston Industrial Plastic Ltd., Montreal, Canada) plastic cylinder (4.0-cm inside diameter, 8.0-cm outside diameter, and 22-cm height) was used as the insulating chamber for holding the sample during the treatment to provide temperature stability (). Full details of this chamber and its performance are given in Shao et al.[Citation18] The insulator was assembled in pressure vessel for delaying heating transfer from sample tubes to pressure vessel during high pressure processing. This design allowed pressure equivalence between inside and outside of insulator. This insulator had a plug with threads installed on the top for thermocouple insertion and a plastic moveable stopper at the bottom. The insulator was filled with distilled water. Two samples bags were kept inside with thermocouple attached to one of them and this insulator assembly was positioned inside the pressure vessel for delaying heat transfer from sample bags to pressure vessel during high pressure processing.
Two K-type thermocouples (OMEGA Engineering, Stamford, CT) attached to a data-logger were installed through the vessel plug: one installed in the sample pouch for recording sample reference temperature and the second thermal couple was installed outside insulator for monitoring pressure vessel temperature. A typical pressure treatment consisted of pressurization, specified pressure holding time and depressurization. The equipment was operated at a maximum speed 15.4 ± 0.1 MPa/s and up to a maximum pressure of 900 MPa. Pressurization come-up time ranged from 45 to 60 s (depending on the target pressure level), and depressurization time was <15 s. The pulse mode was similar to the above except that there was no holding time. Several pressure levels (700–900 MPa) and treatment temperatures (80–100°C) were selected for kinetic study. After pressure treatment, treated samples were kept in an ice water bath prior to bacterial enumeration. Each experiment was conducted in duplicate and the results were averaged for each sample. Test samples subjected to a 10 min heat treatment at 80°C (heat shock) were used as control.
Our previous study on HP treatment of milk[Citation18] demonstrated that the above insulated chamber provided good temperature stability in test samples to be treated for microbial destruction kinetics at high temperature and high pressure conditions. Regression models were established to compute the processing temperature as a function of initial sample temperature (Ti) (20°C ≤ Ti ≤ 80°C) and pressure (P) (MPa).[Citation18] The conditions employed in this study are detailed in .
Table 1 Experimental setup and temperature control for the kinetic tests
Prior to the HP treatment, insulator and test samples were preheated in a temperature controlled water bath (SL26, Julabo, Labortechnik GMBH, Germany) to stabilize their temperature at the pre-selected level (initial temperature). Then the sample bags were placed in the insulator and the set up was closed by a plug and pushing up the bottom piston to eliminate the entrapped air from inside the chamber. The insulator was quickly then assembled in HP chamber and the test started.
Thermal Treatment
A glycerin bath (SL26, Julabo, Labortechnik GMBH, Germany) was used to treat samples at 90, 95 and 100 ± 0.1°C. A temperature reference sample bag (with a K-type thermocouple tip at the bag center) was placed in the glycerin bath along with samples. A datalogger (Agilent 34970A, formerly HP, Agilent Technologies Canada Inc., Mississauga, ON) was used to gather sample and bath temperatures. When the reference temperature reached the desired set-point temperature (bath temperature), the timer was started to measure the holding time. The come-up time was 5 to 6 min, which depended on process temperature, and was excluded from the kinetic studies such that no corrections were necessary. Since the extent of destruction during the come-up time was very small (less than 0.25 log reductions), and the treatment times were relatively long, this exclusion would not affect the computed kinetic parameters. Treated samples were taken out at different points of time for microbiological enumeration and kept in ice water bath.
Enumeration
After HP treatment, the sample bags were aseptically opened and 10 g sample transfered to a stomacher bag and mixed with 90 g sterile distilled water. Serial dilutions were made with 0.1% peptone water. Spores of C. sporogenes were enumerated in Modified PA3679 agar (MP3679A) plates, which has 10 g tryptone (Oxiod, Basingstoke, England, UK); 2 g yeast extract (BD, Co. Spark, MD, USA); 2 g K2HPO4 (Acros Organics, NJ, USA); 2 g soluble starch (Sigma, St. Louis, MO); and 15 g bacterial agar (Fisher Scientific, Fairlawn, NJ) in 1 liter distilled water, by using a pour-plate technique. These MP3679A plates were incubated at anaerobic 37°C condition for five days before counting.
Kinetic Data Analysis
Small variations in the initial concentrations were unavoidable since tests were carried out over several days. Hence, all counts were normalized by multiplying the survival fraction under each condition with the nominal initial count (106 CFU/ml). This will not affect the rate parameters but helps to better compare the different survivor curves on the same plot. The pressure inactivation kinetics of C. sporogenes spores at constant temperature and pressure was analyzed using a first-order kinetic model:
The pressure resistance was analyzed by pressure death time (PDT) model. In PDT model, the pressure resistance at a constant temperature is obtained by plotting the logarithm of D value against the pressure (P). From the regression of 1og (D) vs. P, the pressure resistance z-value (ZT) was determined as the negative reciprocal of the slope as shown below:
Temperature Correction
The insulator was used to delay heat transfer and keep sample temperature relatively stable during the pressure holding period, but there was always a small and steady drop in the sample temperature due to the temperature imbalance between the hot sample/pressure medium and the relatively cooler pressure chamber vessel during pressure holding period. Therefore, it was necessary to correct the kinetic data and eliminate the influence of minor sample temperature fluctuations. Corrections were based on the first order kinetic analysis theory and the concepts used in thermal process calculations.[Citation31] This concept has been detailed in previous studies.[Citation32] An effective time teT equation was established as the accumulation of temperature and time integration:
Table 2 Decimal reduction time of C. sporogenes 7955 spores in salmon slurry subjected to thermal treatment
RESULTS AND DISCUSSION
Thermal Destruction Kinetics
shows the survivor curves for C. sporogenes 7955 spores suspended in salmon slurry under thermal processing conditions (90–100°C) and selected holding times. summarizes the associated D and z values of thermal destruction kinetics. The thermal destruction characteristics C. sporogenes 7955 spores suspended in salmon meat slurry were similar to those observed previously for C. sporogenes ATCC 11437 and ATCC 7955 in milk. They were well described by the first order log linear trends (R2 > 0.98). Higher temperatures resulted in lower D values. The associated D values were 104, 19.9, 7.5 min at 90, 95, 100°C, respectively. The D values in salmon slurry were lower than those found for the same strain in milk (156, 57.5, 12.1 min found at 90, 95, 100°C), but higher than observed for C. sporogenes 11437 spores in milk (65.8, 26.3, 6.0 min at 90, 95, 100°C, respectively).[Citation36] Zhu et al.[Citation19] found relatively larger D-values of 103.8, 41.9, and 16.2 min at 90, 95, and 100°C, respectively, with a z-value of 12.4°C when the spores were suspended in ground beef. Hence, the strain dependent and media dependent variations become obvious. The corresponding z value was 8.8°C (), compared to 9.0°C found for the same in milk.[Citation36] Again there were differences, but much smaller. Overall, for thermal destruction, C. sporogenes 7955 spores in milk offered the most resistance.
Sample Temperature during HP Treatment
illustrates the temperature profile for a test run at 700 MPa at 80°C for a 24 min treatment. Before treatment, the water initial temperature and chamber medium temperature were set at 52.5°C and 61.5°C. During pressurization, water, sample and glycerine temperatures increased due to the adiabatic heating. When the pressure reached desired process pressure 700 MPa, the sample reached a temperature 79.8°C and chamber medium at 93.7°C. Due to the higher temperature of the medium in pressure chamber outside the insulator, the product internal temperature inside the insulator slowly rose to a maximum of 80.8°C at the sixth min and then dropped gradually to 78.6°C before depressurization. Temperature verification experiments demonstrated that the large volume insulator temperature change had the same trend as those in the smaller volume insulator.[Citation18] The selected initial conditions, process temperatures achieved and their stability measured as standard deviation during the test runs are shown in indicating fairly stable test conditions.
High Pressure Destruction Kinetics
show C. sporogenes 7955 spores survival curves at 700, 800, and 900 MPa, respectively, at 80, 90, and 100°C as a function of pressure holding time. The pressure destruction curves showed a small shift from the initial value as a result of the pressure pulse which was then followed by a first order rate destruction during the pressure hold time. summarizes the pressure pulse effect on C. sporogenes 7955 spores. High pressure (700–900 MPa) combined with 100°C caused 0.67–0.1.35 log-reductions while effect was negligible at 80°C. Compared with previous studies, C. sporogenes 7955 spores in salmon were more sensitive to pulse pressure than in buffer, milk or meat.[Citation13,Citation19,Citation36] The extent of pressure pulse destruction is relatively small, but not insignificant, as compared to other studies with vegetative bacterial cells.[Citation37]
Figure 5 Uncorrected (a) and temperature corrected (b) survivors of C. sporogenes 11437 spores in salmon meat slurry pressure treated at 700 MPa and different temperatures: (♦) 80°C, (■)90°C, (▲) 100°C.
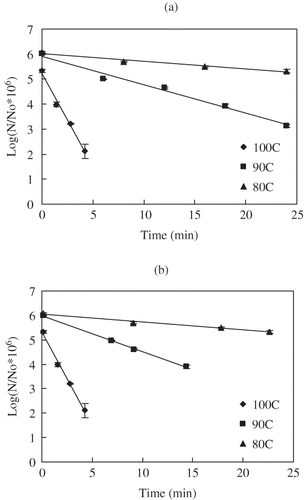
Figure 6 Uncorrected (a) and temperature corrected (b) survivors of C. sporogenes 11437 spores in salmon meat slurry pressure treated at 800 MPa and different temperatures: (♦) 80°C, (■)90°C, (▲) 100°C.
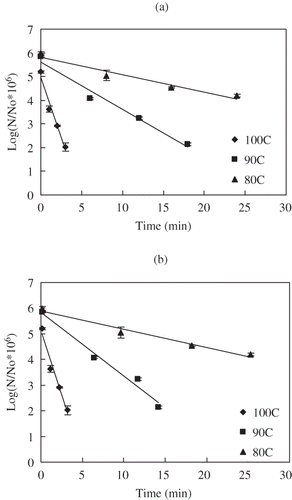
Figure 7 Uncorrected (a) and temperature corrected (b) survivors of C. sporogenes 11437 spores in salmon meat slurry pressure treated at 900 MPa and different temperatures: (♦) 80°C, (■)90°C, (▲) 100°C.
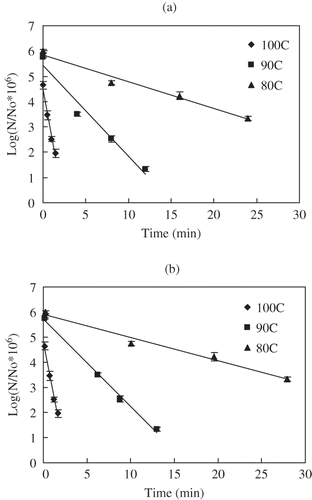
During pressure holding time, the destruction trend was well fitted to the first order model. Computed D values from the regression analysis are summarized in . Higher pressures and higher temperatures resulted in a decrease in D value meaning acceleration in destruction of bacterial spores. Before corrections were applied (), D values at 700, 800, 900 MPa were 32.8, 13.6, 9.4 min and 1.3, 1.0, 0.6 min for HP treatment at 80 and 100°C, respectively. These values were slightly lower than for the same strain in milk medium (34.8, 17.2, 12.5 min and 2.7, 1.8, 1.3 min for HP treatment at 80, 100°C respectively). After corrections, the resulting D values at 700, 800, 900 Pa were 31.2, 14.0, 10.9 min and 1.3, 1.0, 0.6 min at 80, 100°C, respectively (, , and ; ). The D values of C. sporogenes 7955 spores in buffer for 700, 800 MPa were 4.9, 4.5 min and 2.83, 2.3 min at 91, 100°C, respectively.[Citation13] Rovere et al.[Citation38] reported inactivation kinetics of C. sporogenes 7955 in meat broth, which were 6.8, 5.3 min for 700, 800 MPa at 93°C and 3.5, 3.2 min for 600, 700 MPa at 100°C, respectively. Similar results were observed C. sporogenes PA 3679 spores in beef.[Citation19] Compared with C. sporogenes 7955 spores in different media, the D values C. sporogenes 7955 strain in salmon appear to be relatively lower.
Table 3 Effect of pressurization come-up time (CUT) and depressurization come-down time (CDT) on C. sporogenes 7955 spores reduction
Table 4 Decimal reduction time (D values) of C. sporogenes 7955 spores in salmon meat slurry with HP and thermal treatment
Comparing HPHT processing with thermal treatments at the same temperature conditions (90, 100°C), it can again be recognized that HPHT was more efficient with considerably lower D values for C. sporogenes 7955 spores ( and ). The thermal processing D value at 90°C was 15 times higher than the HPHT D value at 700 MPa, 25 times at 800 MPa and 75 times at 900 MPa. Likewise at 100°C, the relative D values were 6 times at 700 MPa, 8 times at 800 MPa and 12 times at 900 MPa. The differences were noticeably getting narrower as the process temperature increased. These results indicate that high pressure combined with mild heat can inactive bacterial spores and effectively shorten the processing times, especially at lower processing temperatures.
Pressure and Thermal Dependency Study
ZT and ZP of C. sporogenes 7955 spores suspended in salmon meat were analyzed from the respective D value curves either as a function of pressure or temperature. Thermal sensitivity (at constant pressure) of D values was described by the ZP value (). The ZP were 14.5, 17.3, 15.5°C for 700, 800, 900 MPa, respectively (), compared to 16.5, 20.3, 18.9°C, for 700, 800, 900 MPa, respectively, in milk.[Citation36] By comparison, ZP values of C. sporogenes 7955 spores in milk and salmon meat were relatively similar. Higher ZP value meant lower thermal sensitivity. Comparing these ZP values with thermal treatment z value (8.8°C), one can find that the bacterial spores had higher thermal resistance under pressure, even though they had lower D values.
Table 5 High pressure ZP value of C. sporogenes 7955 spores in salmon meat slurry subjected to HP and thermal treatments
Figure 8 Uncorrected (a) and corrected (b) D value curves of C. sporogenes 11437 spores in salmon meat slurry subjected to HP and thermal treatments at different temperatures: (♦) 80°C, (■)90°C, (▲) 100°C as a function of pressure.
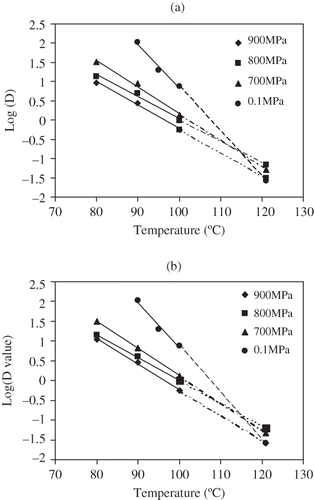
Similarly, the pressure resistance (ZT) value was obtained from the linear relationship at each constant temperature (). Corrected ZT values C. sporogenes 7955 in salmon meat slurry were 440, 540, 550 MPa at 80, 90, 100°C, respectively (), which indicated pressure resistance increased with process temperature. These ZT values of C. sporogenes 7955 spore in fish meat were similar to those in milk.
Table 6 High pressure ZT value of C. sporogenes 7955 spores in salmon meat slurry subjected to HP and thermal treatments
D values at Higher Temperature
Using the ZP and ZT, D values were computed for an extrapolated temperature of 121°C to compare the thermal and pressure D values at commercial processing temperatures. also illustrates the D value curves against temperature and shows the computed D values at specific temperatures (105, 110, 115, 121°C). It can be found that extrapolated HP D values of C. sporogenes 7955 were almost same as the thermal D values at around 121°C. Again, because of the larger ZP values associated with pressure, HPHT D values changed relatively slowly with temperature. On the other hand, the thermal D values, which were several folds higher at lower temperatures, converge rapidly because of their lower z values. It meant that at the temperature close to 121°C, high pressure treatment may not accelerate Clostridial spore destruction. Margosch et al.[Citation14] observed that a pressure-mediated protection happened on B. amyloliquefaciens TMW 2.479 spores at 120°C and 800 to 1200 MPa. Ahn et al.[Citation16,Citation17] reported that at 700 MPa at 121°C, B. amyloliquefaciens ATCC 49763 spores hand a lower spore count reduction than in a thermal treatment at 121°C.
Table 7 Predicted decimal reduction time (D values) of C. sporogenes 7955 spore in HP and thermal treated salmon meat slurry at higher temperatures
This seemingly contradictory behavior can be explained by the differences in pressure and temperature effects. Higher temperatures are associated with a higher kinetic energy and would shift the reaction kinetics to the farther side of the activation energy accomplishing a more rapid destruction. Pressure effect is based on activation volume and reactions resulting in a volume decrease are accelerated by pressure. Higher pressures therefore favor better destruction rates. Therefore, it is logical to expect an increase in pressure and temperature to enhance the destruction rates. However, higher temperatures result in a volume expansion as opposed to higher pressures, which result in a volume decrease, hence these two are generally opposing. The pressure effect therefore can be expected to be lower at higher temperatures. At lower temperatures, pressure becomes the dominating factor while at higher temperatures, temperature effect dominates over the pressure effect. This explains the progressive decrease in the pressure acceleration of destruction rates at higher temperatures. Further research is, however, necessary to verify the destruction patterns at temperatures in the 115–130°C range where the reversal of the pressure temperature role may occur.
CONCLUSIONS
Higher pressures and high temperatures resulted in a faster destruction of C. sporogenes 7955(PA3679) spores in salmon slurry (smaller D value). High pressure combined with elevated temperature was able to destroy C. sporogenes 7955(PA3679) spores in salmon meat and had a greater efficiency than thermal processing. C. sporogenes 7955(PA3679) spores in milk were more pressure resistant than C. sporogenes 7955(PA3679) spores in salmon slurry or meat slurry suggesting that the pressure resistance depends on the medium. Media in which the kinetic tests are carried out plays a significant role, and for process establishment the media representing the product should be employed. High pressure processing at moderate temperatures (80–100°C) significantly accelerated the destruction rates of the bacterial spore; however level of acceleration rapidly decreased as the process temperatures were elevated. At temperatures of 120°C or higher, the acceleration effect may totally become non-existing. However, high-pressure processing would still be attractive as a powerful means of quick heating and cooling because of the adiabatic heating during pressurization and cooling during depressurization and would provide a quality advantage for commercial sterilization of low acid foods.
ACKNOWLEDGMENTS
The authors gratefully acknowledge the financial support by the Strategic Grants Program of the Natural Sciences and Engineering Research Council of Canada (NSERC, Ottawa, ON, Canada). The authors would like to thank Dr. Fadia Naim and Dr. Michèle Marcotte of Food Research and Development Centre, Agriculture and Agri-Food Canada (CRDA, St. Hyacinthe, QC, Canada) for sharing the strain of C. sporogenes 7955 and preparation methods.
REFERENCES
- Cheftel , J.C. 1991 . Applications des hautes pressions en technoloie alimentaire . Actualites des Industries Alimentaire et Agro-Alimmentaire , 108 : 141 – 153 .
- He , H.A. , Adams , R.M. , Farkas , D.F. and Morrissey , M.T. 2001 . The use of high hydrostatic pressure to shuck oysters and extend shelf-life . Journal of Shellfish Research , 20 : 1299 – 1300 .
- Knorr , D.. 1995 . “ Hydrostatic pressure treatment of food: microbiology ” . In New Methods for Food Preservation , Edited by: Gould , G.W. 159 – 175 . London : Blackie Academic and Professional .
- Zhu , S. , Ramaswamy , H.S. and Simpson , B.K. 2004 . Effect of high-pressure versus conventional thawing on color, drip loss and texture of Atlantic salmon frozen by different methods . Lebensm.-Wiss. U.-Technol. , 37 ( 3 ) : 291 – 299 .
- Ahmed , J. , Ramaswamy , H.S. , Alli , I. and Raghavan , G.S.V. 2007 . Protein denaturation, rheology, and gelation characteristics of radio-frequency heated egg white dispersions . International Journal of Food Properties , 10 ( 1 ) : 145 – 161 .
- Ahmed , J. , Ayad , A. , Ramaswamy , H.S. , Alli , I. and Shao , Y. 2007 . Dynamic viscoelastic behavior of high pressure treated soybean protein isolate dispersions . International Journal of Food Properties , 10 : 397 – 411 .
- Hugas , M. , Garriga , M. and Monfort , J.M. 2002 . New mild technologies in meat processing: high pressure as a model technology . Meat Science , 62 ( 3 ) : 359 – 371 .
- Maggi , A. , Gola , S. , Rovere , P. and Miglioli , L. 1996 . Dall'Aglio, G . Lonneborg, N.G. Effects of combined high pressure–temperature treatments on Clostridium sporogenes spores in liquid media. Industria Conserve , 71 : 8 – 14 .
- Stumbo , C.R. 1973 . Thermobacteriology in food processing , 329 New York, NY : Academic Press .
- Mills , G. , Earnshaw , R. and Patterson , M.F. 1998 . Effects of high hydrostatic pressure on Clostridium botulinum spores . Letters in Applied Microbiology , 26 : 227 – 230 .
- Reddy , N.R. , Solomon , H.M. , Tetzloff , R.C. and Rhodehamel , E.J. 2003 . Inactivation of Clostridium botulinum type A spores by high pressure processing at elevated temperatures . Journal of Food Protection , 66 ( 8 ) : 1402 – 1407 .
- Reddy , N.R. , Tetzloff , R.C. , Solomon , H.M. and Larkin , J.W . 2006 . Inactivation of Clostridium botulinum nonproteolytic type B spores by high pressure processing at moderate to elevated high temperature . Innovative Food Science and Emerging Technologies , 7 : 169 – 175 .
- Koutchma , T. , Guo , B. , Patazca , E. and Parisi , B. 2005 . High pressure-high temperature sterilization: from kinetic to analysis to process verification . Journal of Food Process Engineering , 28 : 610 – 629 .
- Margosh , D. , Ehrmann , M.A. , Buckow , R. , Heinz , V. , Vogel , R.F. and Ganzle , M.G. 2006 . High-pressure-mediated survival of Clostridium botulinum and Bacillus amyloliquefaciens endospores at high temperature . Applied and Environmental Microbiology , 72 ( 5 ) : 3476 – 3481 .
- Scurrah , K.J. , Robertson , R.E. , Craven , H.M. , Pearce , L.E. and Szabo , E.A. 2006 . Inactivation of Bacillus spores in reconstituted skim milk by combined high pressure and heat treatment . Journal of Applied Microbiology , 101 : 172 – 180 .
- Ahn , J. , Balasubramaniam , V.M. and Yousef , A.E. 2007 . Inactivation kinetics of selected aerobic and anaerobic bacterial spores by pressure-assisted thermal processing . International Journal of Food Microbiology , 113 : 321 – 329 .
- Ahn , J. and Balasubramaniam , V. E. 2007b . Effects of inoculum level and pressure pulse on the inactivation of Clostridium sporogenes spores by pressure-assisted thermal processing . Journal of Microbiology and Biotechnology , 17 ( 4 ) : 616 – 623 .
- Shao , Y. , Zhu , S. , Ramaswamy , H.S. and Marcotte , M. 2010 . Compression heating and temperature control for high pressure destruction of bacterial spores: an experimental method for kinetics evaluation . Food and Bioprocess Technologies , 3 ( 1 ) : 71 – 78 .
- Zhu , S. , Naim , F. , Marcotte , M. , Ramaswamy , H.S. and Shao , Y. 2008 . High-pressure destruction kinetics of Clostridium sporogenes spores in ground beef at elevated temperatures . International Journal of Food Microbiology , 126 ( 1–2 ) : 86 – 92 .
- Hoogland , H. , de Heij , W.B.C. and van Schepdael , L. 2001 . High pressure sterilization: novel technology, new products, new opportunities . New Food , 4 ( 1 ) : 21 – 26 .
- de Heij , W.B.C. , van Schepdael , L.J.M.M. , Moezelaar , R. , Hoogland , H. , Matser , A.M. and van den Berg , R.W. High-pressure sterilization: Maximizing the benefits of adiabatic heating. Food Technology . 2003 , 57 ( 3 ) 37 – 41 .
- Matser , A.M. , Krebbers , B. , van den Berg , R.W. and Bartels , P.V. 2004 . Advantages of high pressure sterilization on quality of food products . Trends Food Science Technology , 15 ( 2 ) : 79 – 85 .
- Sareevoravitkul , R. , Simpson , B.K. and Ramaswamy , H.S. 1996 . Effects of crude a2-macroglobulin on properties of bluefish (Pomatomus saltatrix) gels prepared by high hydrostatic pressure and heat . J. Food Biochemistry , 20 ( 1 ) : 49 – 63 .
- Sareevoravitkul , R. , Ramaswamy , H.S. and Simpson , B.K. 1996 . Comparative properties of bluefish (Pomatomus saltatrix) gels formulated by high hydrostatic pressure and heat . Journal of Aquatic Food Product Technology , 5 : 65 – 79 .
- Ashie , I.N.A. , Simpson , B.K. and Ramaswamy , H.S. 1996 . Changes in texture and microstrusture of pressure-treated fish muscle during chilled storage . J. Muscle Foods , 8 ( 1 ) : 13 – 32 .
- Ashie , I.N.A. , Simpson , B.K. and Ramaswamy , H.S. 1996 . Control of endogenous enzyme activity in fish muscle by inhibitors and hydrostatic pressure using RSM . Journal of Food Science , 61 ( 2 ) : 350 – 356 .
- Rouille , J. , Lebail , A. Ramaswamy , H.S. 2002 . High pressure thawing of fish and shellfish . J. Food Eng. , 53 ( 1 ) : 83 – 88 .
- Sequeira-Munoz , A. , Chevalier , D. , LeBail , A. , Ramaswamy , H.S. and Simpson , B.K. 2006 . Physicochemical changes induced in carp (Cyprinus carpio) fillets by high pressure processing at low temperature . Innovative Food Science & Emerging Technologies , 7 ( 1–2 ) : 13 – 18 .
- Sequeira-Munoz , A. , Chevalier , D. , Simpson , B.K. , Le Bail , A. and Ramaswamy , H.S. 2005 . Effect of pressure-shift freezing versus air-blast freezing of carp Cyprinus carpio fillets: A storage study . Journal of Food Biochemistry , 29 ( 5 ) : 504 – 516 .
- Ramaswamy , H.S. , Zaman , S.U. and Smith , J.P. 2008 . High pressure destruction kinetics of Escherichia coli (O157: H7) and Listeria monocytogenes . Journal of Food Engineering , 87 ( 1 ) : 99 – 106 .
- Patashnik , M. 1953 . A simplified procedure for thermal process evaluation . Food Technology , 7 ( 1 ) : 1 – 6 .
- Patazca , E. , Koutchma , T. and Ramaswamy , H.S. 2006 . Inactivation Kinetics of Geobacillus stearothermophilus Spores in Water Using High-pressure Processing at Elevated Temperatures . Journal of Food Science , 71 ( 3 ) : M110 – 116 .
- Tajchakavit , S. and Ramaswamy , H.S. 1997 . Thermal vs. microwave inactivation kinetics of pectin methylesterase in orange juice under batch mode heating conditions . Lebensmittel-Wissenschaft and Technologies , 30 : 85 – 93 .
- Tajchakavit , S. , Ramaswamy , H.S. and Fustier , P. 1998 . Enhanced destruction of spoilage microorganisms in apple juice during continuous flow microwave heating . Food Rsearch International , 31 ( 10 ) : 713 – 722 .
- Awuah , G.B. , Ramaswamy , H.S. , Simpson , B.K. and Smith , J.P. 1993 . Thermal inactivation kinetics of trypsin at aseptic processing temperatures . Journal of Food Process Engineering , 16 : 315 – 328 .
- Shao , Y. and Ramaswamy , H.S. High pressure destruction kinetics of C. sporogenes 7955 spores in milk at elevated temperatures . IFT Conference Abstracts . June 25 , Orlando, FL.
- Ramaswamy , H.S. , Riahi , E. and Idziak , E. 2003 . High-pressure destruction kinetics of E-coli(29055) in apple juice . J. Food Sci. , 68 ( 5 ) : 1750 – 1756 .
- Rovere , P. , Carpi , G. , Dall'Aglio , G. , Gola , S. , Maggi , A. , Miglioli , L. and Scaramuzza , N. 1996 . High-pressure heat treatments: Evaluation of the sterilizing effect and of thermal damage . Industria Conserve , 71 : 473 – 483 .