Abstract
The paper investigates the impact of osmotic dehydration on the mechanical properties (i.e., structural changes and shrinkage) of freeze-dried strawberries. In the research, Senga Sengana strawberries were osmotically dehydrated in sucrose, glucose solution, and starch syrup and subjected to freeze-drying. The osmotic dehydration process strengthened the fruit structure by increasing their cell wall thickness. Osmotic pre-treatment limited the shrinkage of the strawberries by about 50%, while the compression force required for 25% deformation of the dried material was almost 2–963 times greater in comparison to the fruit not subjected to osmotic dehydration, dependent on the type of osmotic solution used.
INTRODUCTION
A common way of preserving food is decreasing its water content through drying.[Citation1] During the convective-drying process the amount of free space in plant tissue decreases, which leads to the disintegration of cellular walls and formation of larger structures under the shrinkage stress.[Citation2] Continuous improvement of the convective-drying process aimed at obtaining high-quality dried apples[Citation3] and blueberries[Citation4] has led to the development of freeze-drying technology. Considering the fact that customers are always looking for new products of improved quality, freeze-drying will definitely meet their expectations.[Citation5]
Freeze-dried fruit are characterized by a fragile and open structure which makes them susceptible to chemical changes, as well as mechanical damage during production, packaging, transport and storage. Therefore, the need arises to keep the adverse factors at bay.[Citation1] One possible solution is to use osmotic dehydration as a pre-treatment method. Osmotic dehydration is a process defined as a partial dehydration by immersion of fruit in sugar or sugar solution for a definite period of time.[Citation6]
Texture is the most important feature of processed food,[Citation7] and also the main determinant in consumer's quality assessment.[Citation8] In plant tissues, the texture mostly depends on the characteristics and shape of cellular walls.[Citation9] The structure and functional properties of osmotically dehydrated products (e.g., texture, colour) depend mainly on the changes in composition resulting from impregnation, as well as from the influence of the dehydration process on cellular walls, membranes, and cell contents.[Citation10]
The influence of some sugars on changes in the structure of osmotically dehydrated plant material can be determined on the basis of texture analysis, particularly tissue damage and volume change.[Citation11] For instance, fruit saturated with a glucose solution retain cellular wall shapes similar to fresh fruit, while those osmotically dehydrated in a sucrose solution have their cellular walls more damaged.[Citation12] Osmotic dehydration also causes deformation and rugosity of the cellular surface, which influences the shape and volume of intercellular spaces. As a consequence, the structure of osmotically dehydrated apple tissue is less regular than that of fresh fruit.[Citation13]
Also, the mechanical properties of food products depend on structural changes rendered by technological processes. In foods subjected to osmotical dehydration followed by convection drying, the changes are caused by the presence of osmotic substances, e.g., sugars. These changes, however, have not been a common subject of study,[Citation14] and the results obtained from the analyses made so far are to a large degree contradictory. Lewicki and Łukaszuk[Citation14] affirmed that subjecting the apple tissue to osmotic dehydration before convective drying significantly influenced the tissue's rheological properties: in particular, it enriched the tissue in sugar, and considering that the material was treated with a concentrated, sticky solution, it also made the tissue more vulnerable to deformation in comparison with fruit convectively dried to the same water content without preprocessing. The concentration of sugar solution in the surface layer of osmotically dehydrated apples prevented the formation of a rigid coating during the convective drying, and ensured uniform brittleness. Surface hardening still took place with osmotical dehydration and convection-drying to low water content. The osmotic dehydration of onion before convective drying caused a decrease in compression work by almost 50%, but had no influence on the relaxation time.[Citation15]
Monsalve-Gonzalez, Barbosa-Canovas and Cavalieri[Citation16] showed that osmotic pre-treatment influenced the surface structure of osmotically dehydrated fruit only to a depth of 1 mm, so, in principle, the structure was similar to that of apples not subjected to osmotic dehydration. Jakubczyk, Sitkiewicz, and Lewicki[Citation17] recommended osmotic dehydration for apples, because it decreased their hardness and influenced the relaxation rate. However, the compression test to 25% of initial dry material height for osmotically dehydrated freeze-dried strawberries required a significant increase in compression force in comparison to fruit not subjected to osmotic pre-treatment. At the same time, the type of osmotic solution used in the test proved to be irrelevant.[Citation1]
The aim of this work was to investigate the influence of osmotic dehydration on the structure of freeze-dried strawberries, taking into consideration changes in the fruit's mechanical properties. Various conditions of osmotic dehydration were taken into account. An attempt was made at defining pre-treatment conditions for freeze-drying of strawberries with the purpose of influencing the structural changes in the freeze-dried fruit. During the process, frozen strawberries were osmotically dehydrated to equal water content, then frozen again and eventually freeze-dried.
MATERIALS AND METHODS
Strawberries of Senga Sengana variety, frozen, deprived of leaf stalks, and about 25–30 mm in diameter was used in this study. They had been stored in plastic pouches of 500 g each at the temperature of −18°C for 12–18 months. The frozen strawberries were osmotically dehydrated in solutions with water activity equal to 0.9 [sucrose 61.5 g/100 g solution, glucose 49.2 g/100 g solution, starch syrup (glucose equivalent DE 30–35) 67.2 g/100 g solution] in a water bath (ELPAN-357) at the temperature of 30°C for 3 h under atmospheric pressure. The ratio of material to solution was 1:4 w/w.[Citation19,Citation20] Additionally, the system was shaken with the frequency of 100 Hz and the amplitude of 10 Hz. After the specified period of time the strawberries were separated from the osmotic solution with a sieve, and rinsed twice in water.
Osmotically dehydrated strawberries were frozen in a National Lab GmbH (ProfiMaster Personal Freezers PMU series) freezer at the temperature of −70°C for 2 h. Both osmotically dehydrated and unprocessed frozen strawberries were then dried for 24 h in an ALPHA1–4 LDC-1m freeze-dryer (Christ, Germany) using contact heating under the pressure of 63 Pa, safety pressure 103 Pa, the dryer shelves temperature being 30°C. During the process of drying, the fruit temperature was being monitored by a thermocouple which indicated that the temperature inside osmotically dehydrated strawberries rose from −30 to 25°C. Subsequently, the fruit were put into jars and stored in a dark place at the temperature of 25 ± 3°C until the time of planned examination.
Moreover, to show the influence of the drying method on fruit structure, both osmotically dehydrated in sucrose solution and unprocessed (i.e., not osmotically pre-treated) convection-dried fruit were produced. The convection drying was conducted at the temperature of 60°C for 3 h with the air velocity of 1.8 m/s.
Shrinkage of freeze-dried strawberries was measured with a volumetric method (using toluene displacement). Initially, two freeze-dried strawberries, weighed on an analytical balance, were placed in a graduated cylinder. Then, from a burette of the same volume as the cylinder toluene was being poured onto the fruit until the liquid's lower meniscus reached a value equal to the burette volume. The volume of strawberries was calculated as the difference between the nominal cylinder volume and the volume of the toluene residue in the burette. This measurement was repeated five times.[Citation21]
The determination of mechanical properties of freeze-dried strawberries involved compression force measurement using a TA-XT2i Texture Analyser at the temperature of 20 ± 2°C. The measurement was repeated four times. The fruit were compressed at a constant rate of 20 mm/min, until 25% deformation of the initial height of the freeze-dried fruit was achieved. As a result, maximal compression force and compression work were determined.
Structural changes were examined using an FEI Quanta 200 scanning electron microscope. Freeze-dried strawberries were additionally vacuum-dried for 2 hours at the temperature of 50°C, and subsequently cut along their axes with a razor blade. Next, pieces of about 3 mm thick were cut out from the lower part of the dried fruit. Structural changes were scrutinised under 50× and 100× magnification. Using the Multi Scan computer software and relying on the images taken at 50× magnification, average area (mm2), perimeter (mm) and Feret diameter (mm) for individual spaces formed by cells in convection-dried and freeze-dried strawberries were calculated.
The microstructure of freeze-dried strawberries was examined by birefringent interferometry, using a Biolar PI microscope.[Citation22] Again, freeze-dried strawberries were vacuum-dried for 2 h at the temperature of 50°C, and cut along their axes with a razor blade. This time, samples ca 0.01 mm. thick were cut out transversely to the surfaces of halved freeze-dried fruit. Images were taken under ×600 and ×1200 magnification. Moreover, images of crystal forms on the surfaces of freeze-dried strawberries stored for one year were made. Pictures in the jpeg format were obtained with a Casio QV-2900UX digital camera.
The statistical analysis Statgrafics Plus, version 3.0. (Microsoft), Excel 2000 (Microsoft), Table Curve 2D v. 3 (Jadel), and Statistica. 5.0 (StatSoft) software was used in this study. For the obtained averaged results standard deviations (SD) were calculated.[Citation23]
RESULTS AND DISCUSSION
Shrinkage of Freeze-Dried Strawberries
To estimate the influence of osmotic dehydration together with the type of osmotic solution on shrinkage of freeze-dried strawberries as compared to frozen fruit, the average value of shrinkage was employed (Sav) (). According to Krokida and Maroulis,[Citation24] volume reduction practically fails to occur in freeze-dried products, because of limited shrinkage and minimal structural deformation in comparison to raw material. Also, Ratti[Citation25] describes shrinkage of freeze-dried strawberries as very insignificant, ranging from 5–15%, stating for comparison that in the case of convective drying shrinkage equals 80%. According to Aguilera,[Citation26] as well as Szarycz et al.[Citation27] during the freeze-drying process only minimal shrinkage takes place, unless the temperature of structural collapse (Tg = 45°C) is exceeded. Shishehgarha, Makhlouf and Ratti[Citation28] proved that when heating-shelves temperature in a freeze dryer exceeded 50°C, structural collapse occurred in more than 20% of the analysed strawberries, which could be regarded as product homogeneity loss. Moreover, such dried material exhibited significant volume changes and detrimental changes in appearance (e.g., colour distortion).
Figure 1 Influence of osmotic dehydration and the type of osmotic solution on average shrinkage (Sav) of osmotically dehydrated freeze-dried strawberries.
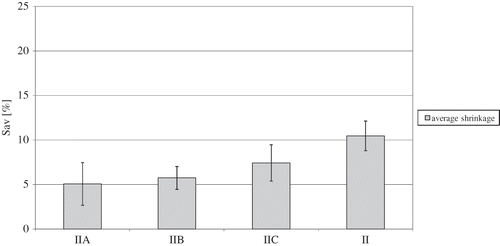
When comparing shrinkage rates of freeze-dried strawberries with osmotic dehydration to those of freeze-dried strawberries with no subjection to osmotic dehydration, it can be easily noticed that in the former shrinkage was significantly reduced (II) (), the differences varying from 3–5%. According to Nieto, Castro, and Alzamora,[Citation29] tissue shrinkage of fruit before drying is related to osmotic pre-treatment. The increased volume of freeze-dried and vacuum-dried products obtained through osmotic dehydration resulted from the fact that drying methods caused porosity-increasing structural changes. Osmotic pre-treatment in a sucrose solution for strawberries, currants, cherries and plums at water activity of 0.5 caused volume growth of freeze-dried strawberries of about 8%.[Citation30]
Statistically significant differences in average shrinkage rates of strawberries osmotically dehydrated in glucose solution (IIB) and starch syrup (IIC) amount to 1.7%. As well as that, between strawberries osmotically dehydrated in sucrose solution (IIA) and starch syrup (IIC), and those osmotically dehydrated in sucrose solution (IIA) and glucose solution (IIB) no statistically important differences in shrinkage volume were found. Likewise, Erle, and Schubert,[Citation31] by osmotically dehydrating strawberries and then microwave-drying them, obtained a statistically significant shrinkage decrease in fruit after osmotic dehydration in sucrose solution in comparison to fruit deprived of osmotic pre-treatment. By contrast, Riva et al.[Citation32] who investigated the influence of osmotic dehydration in sucrose solution and sorbitol on properties of apricot convection-dried at a temperature of 70°C, demonstrated that in both cases shrinkage was substantial, with osmotic pre-treatment in sorbitol solution to a small extent increasing the volume of dried material. Additionally, Andreś, Bilbao, and Fito[Citation33] observed a decrease in volume in microwave-convection-dried apples which had been osmotically dehydrated in apple juice, in comparison to fruit not subjected to osmotic pre-treatment. They explained that this phenomenon was related to the greater porosity of non-dehydrated samples (71%) than that of osmotically dehydrated ones (42%).
Mechanical Properties of Freeze-Dried Strawberries
Assessment of the influence of osmotic dehydration and type of osmotic solution on the changes in mechanical properties of freeze-dried strawberries was carried out on the basis of differences in compression curves (). Osmotic dehydration clearly influenced the increment in compression force (F). Also, the type of used osmotic solution had an impact on the compression of freeze-dried strawberries. Only between fruit osmotically dehydrated in sucrose solution (IIA) and in starch syrup (IIC) were there no statistically significant discrepancies in the curves' shape. The compression force and compression work necessary to achieve a 25% deformation was calculated (). It turned out that osmotic dehydration before freeze-drying made it necessary to apply 2–3 times more work, the values ranging from 0.312 to 0.332 J, to obtain 25% deformation of the initial height for dried material than in the case of strawberries without osmotic dehydration (0.085 J) (). Strawberries osmotically dehydrated in a glucose solution (IIB) required the greatest compression work.
Table 1 Average compression work for freeze-dried strawberries. II: freeze-drying. Osmotic solutions: A: sucrose solution; B: glucose solution; and C: starch syrup
Figure 2 Influence of osmotic dehydration and type of osmotic solution on mechanical properties (compression test) of osmotically dehydrated freeze-dried strawberries. Type of osmotic solution: IIA: sucrose; IIB: glucose; IIC –: starch syrup; and II: without osmotic dehydration. F: compression force necessary for 25% deformation of dried material.
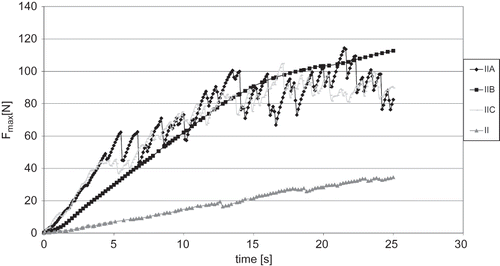
It was also proved that there was a statistically significant effect of osmotic dehydration on the compression force required to obtain 25% deformation of dried material, taking into consideration fruit both with and without osmotic pre-treatment freeze-dried at heating shelves temperature of 30°C (II) (). The differences in this case varied from 81 to 87 N. Furthermore, the analysis of the influences of osmotic solution type showed that insofar as osmotic dehydration caused more than a threefold increase in the necessary compression force, the differences resulting from osmotic solution type were statistically irrelevant ().
Figure 3 Influence of osmotic dehydration and type of osmotic solution on compression force (F) necessary for 25% dried material deformation of osmotically dehydrated freeze-dried strawberries. Type of osmotic solution: IIA: sucrose; IIB: glucose; IIC: starch syrup; and II: without osmotic dehydration.
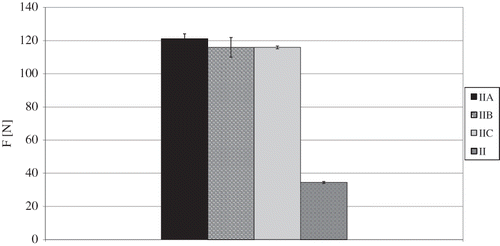
The results confirm the findings which Jakubczyk and Lewicki[Citation34] achieved with osmotically dehydrated in calcium chloride and convection-dried apple slices. Specifically, the fruit's mechanical properties were distinctly different than of those deprived of osmotic pre-treatment. The authors observed a considerable increase in compression resistance even after two-minute soaking in calcium chloride solution. They explained that calcium ions established connections with other middle lamella components of dried material, which could increase cellular cohesion and in consequence lead to the structural strengthening. Similar interactions were observed with pre-treatment in mannitol[Citation32]: there was an upsurge in mechanical resistance, and more than twofold growth of compression work for dry material after osmotic pre-treatment. Just as in the case of calcium chloride, the changes of mechanical properties were associated with structural transformations. During the immersion in mannitol, there might be a component exchange between the cell sap of the immersed tissue and the solution. The replacement of certain amount of water in the biopolymer structure could have increased the structure condensation during drying. Mannitol, filling intercellular spaces, is not removed during the drying process, thus strengthening the structure.
On the other hand, Lewicki and Łukaszuk[Citation14] demonstrated weakening of mechanical properties of convection-dried apples resulting from previous osmotic dehydration in sucrose solution. The dried material was stickier and less resistant to deformation in comparison to that with no osmotic pre-treatment. Also, Sitkiewicz, Lenart, and Lewicki,[Citation35] who used the convection method to dry apples osmotically dehydrated in sucrose solution, proved that osmotic pre-treatment weakens the mechanical resistance of plant material due to membrane semipermeability loss and following penetration of the osmotic solution into surface layers of the material. The increase of sucrose solution concentration in plant tissue caused elevated influence of sticky components on the rheological properties of osmotically dehydrated material. With the growing degree of osmotic dehydration, apples became softer and more plastic, their texture to a greater and greater extent differing from that of raw fruit. Moreover, it was shown that compression force is decreased fourfold in comparison to raw fruit. Those considerable differences illustrate the influence of osmotic pre-treatment on mechanical properties of apples.
Freeze-dried strawberries had different initial water activity aw, it was resolved to investigate the compression force after previously equalizing the water activity in the fruit (). Accordingly, freeze-dried strawberries were stored for three months in hygrostats with water activity from 0.225 to 0.810. It was demonstrated that osmotic dehydration in sucrose solution (IIA) caused a statistically significant increase in compression force necessary for obtaining 25% dry material deformation at water activity 0.225, in comparison to strawberries without osmotic pre-treatment (II), as well as those osmotically dehydrated in glucose solution (IIB), and starch syrup (IIC) (). Water activity rise from 0.225 to 0.432 caused a steep compression force decrease for strawberries osmotically dehydrated in sucrose solution (IIA), namely from 168N to 2N. For other freeze-dried fruit the differences were 40 times smaller. Finally, for strawberries both osmotically dehydrated and without osmotic pre-treatment higher water activity—in the range of 0.432–0.810—did not cause any changes in compression force for investigated freeze-dried material ().
Structure of Freeze-Dried Strawberries
The analysis of the influence of osmotic dehydration, type of osmotic solution, and drying method on changes in fruit structure was conducted using the images acquired with the scanning microscope under 50× and 100× magnification (, ) (). The images, obtained with birefringent interferometry technique, enabled observation of the degree to which sugars migrated into freeze-dried strawberry tissue ().
Table 2 Average area, perimeter, and Feret diameter values for an individual cell of convection-dried and freeze-dried strawberries. Osmotic solutions: A: sucrose solution; B: glucose solution; C: starch syrup; I: convective-drying; and II:– freeze-drying
Figure 7 Degree of impregnation of freeze-dried strawberry tissue not subjected to osmotic dehydration (II) by sugars naturally occurring in the fruit. (a) Fragment of the surface layer—zoom 600×; and (b) Fragment of the inner part of a freeze-dried strawberry—zoom 1200×.
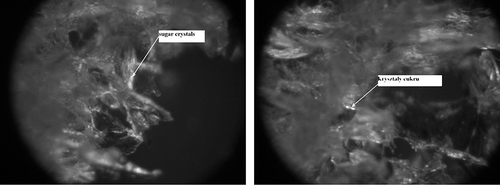
The microstructure examination of dried strawberries without osmotic dehydration showed significant structural changes in convection-dried fruit (I) (), in comparison to freeze-dried fruit (II) (). Convective-drying caused radical changes in the structure of strawberries. Under 100× magnification several large spaces were visible; with all likelihood, they appeared as a result of tearing apart of the cellular walls during convective-drying (). The remaining part of the tissue in the dried material formed a homogenous, compact substance.
Osmotic dehydration in a sucrose solution (IA) () before convective-drying of strawberries effected in greater structural relaxation in comparison to fruit without osmotic pre-treatment (I) (). As a result, the Feret diameter of thus formed internal spaces had greater value (). Also, under 100× magnification the impregnation of intercellular walls with sucrose was visible ().
Calculated average area, average perimeter, and Feret diameter for individual cells (spaces) of convection-dried strawberries without osmotic dehydration (I) were not significantly different in comparison to the values measured in fruit after osmotic pre-treatment (IA) (). In spite of similarities, application of osmotic pre-treatment made strawberries' structure more porous, and their cells were damaged to a smaller degree.
Freeze-dried fruit without osmotic dehydration (II) were characterised by a sensitive structure and thin cellular walls, which was visible under 100× magnification, individual cells having been regular and clearly outlined (). Freeze-dried strawberry tissue without osmotic dehydration (II) was characterised by a sensitive and porous structure with regular arrangement of sugar crystals. In the photographs, the crystals were visible as shining objects ().
The application of osmotic dehydration in sucrose solution (IIA) () caused structural strengthening of freeze-dried strawberries, in comparison to fruit without osmotic pre-treatment (II) (). As a consequence of tissue impregnation with sucrose, cellular walls became bulky, while cells nearest to the dried material's surface suffered substantial damage, which could be clearly seen under ×100 magnification (). In these places the substance was compact, whereas torn apart cellular walls formed large, irregular pores.
Changes in cells which occurred due to sucrose penetration during osmotic dehydration were also visible in juxtaposition of the average area,(average) perimeter, and Feret diameter of individual cells in dried strawberries osmotically dehydrated in sucrose solution (IIA) with these values in fruit without osmotic pre-treatment (II) (). Fruit osmotically dehydrated in a sucrose solution (IIA) were impregnated by the sugar only to a small degree. Sucrose, which during the osmotic pre-treatment penetrated the surface layer, appears mostly in an amorphous form, was invisible in the images taken using birefringent interferometry (). The glowing crystalline objects which were present inside fruit tissue were mostly sugar crystals naturally occurring in strawberries (). Their arrangement in freeze-dried material was similar to that in strawberries without osmotic dehydration (). Sucrose penetrated deep into cells, and during crystallization tore the cellular walls apart, which explained the damage to the surface layer ().
Figure 8 Degree of impregnation of freeze-dried strawberry tissue osmotically dehydrated in a sucrose solution (IIA) by sugars which penetrated from the osmotic solution. (a) Fragment of the surface layer—zoom 600×; and (b) Fragment of the inner part of a freeze-dried strawberry—zoom 1200×.
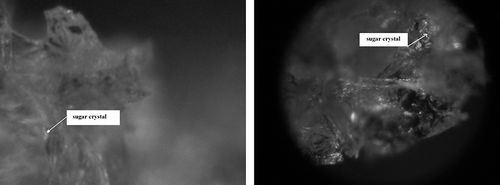
By the same token, glucose also strengthened the structure of freeze-dried strawberries (IIB) () in comparison to fruit deprived of osmotic pre-treatment (II) (), but it was shown that the average area of an individual cell (space) was almost twice as small than in the case of sucrose solution (IIA) (). These considerable differences in cell sizes resulted from the character of the sugar used for osmotic dehydration. Freeze-dried strawberries osmotically dehydrated in glucose solution (IIB) were characterized by a uniform impregnation with the sugar (). This phenomenon probably resulted from smaller molecular mass of glucose in comparison to sucrose, (allowing a deeper penetration of glucose inside the fruit). For the same reason cells which are near the surface of dried material, underwent deformation to a smaller degree. Hence, osmotic dehydration in a glucose solution allowed a uniform impregnation of strawberry tissue. Glucose, which penetrated the tissue during the osmotic dehydration, crystallized evenly in the whole freeze-dried material. The sugar penetrated deep into the fruit (). Shining glucose crystals were also present in the surface layer of osmotically dehydrated freeze-dried strawberries (IIB).
Figure 9 Degree of impregnation of freeze-dried strawberries osmotically dehydrated in a glucose solution (IIB) by sugars which penetrated from osmotic solution. (a) Fragment of the surface layer—zoom 600×; and (b) Fragment of the inert part of a freeze-dried strawberry—zoom 1200×.
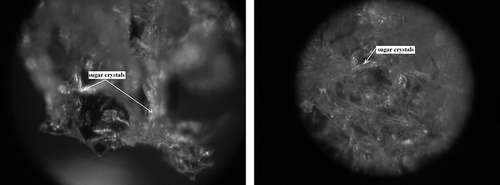
The presence of plate-like structures, typical to glucose crystals, was not observed. However, fibrous structures were discerned (), which are characteristically found in creamed honey.[Citation36] This particular form of glucose crystals means that freeze-dried strawberries osmotically dehydrated in a glucose solution (IIB) are characterized by a greater plasticity in comparison to fruit osmotically dehydrated in sucrose solution (IIA) () or starch syrup (IIC) ().[Citation1] The photograph presents a growing glucose crystal in the freeze-dried tissue.
Figure 11 Degree of impregnation of freeze-dried strawberries osmotically dehydrated in a starch syrup (IIC) by sugars which penetrated from osmotic solution. (a) Fragment of the surface layer—zoom 600×; and (b) Fragment of the inert part of a freeze-dried strawberry—zoom 1200×.
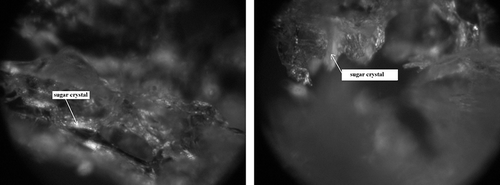
Figure 10 Forms of glucose crystals in freeze-dried strawberries osmotically dehydrated in a glucose solution (IIB). (a) Glucose crystals of fibrous structure; and (b) Form of glucose crystal growth.
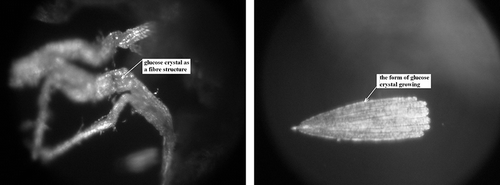
As regards starch syrup, it saturated freeze-dried strawberry tissue, strengthening the structure of the dried material (IIC) () in comparison to fruit without osmotic dehydration (II) (). Starch syrup filled the cells of freeze-dried strawberries, creating a small number of empty spaces (pores). The parameters of those spaces were similar to the average area, perimeter, and Feret diameter values for an individual cell of freeze-dried fruit osmotically dehydrated in a glucose solution (IIB) ().
Furthermore, starch syrup penetrated the tissue to a greater degree than sucrose, but still to a smaller extent than glucose. Most saturated was the surface layer, although the inner cells, far from the surface sugar crystals, were nonetheless visible (). The investigation of changes in surface structures of freeze-dried strawberries which had been stored for one year ascertained high stability of dried fruit (). In dried strawberries deprived of osmotic dehydration (II), similarly to fresh dried fruit (), sugar crystals, naturally occurring in strawberry tissue, were observed. To a large extent, these were glucose crystals having fibrous form (). For strawberries osmotically dehydrated in sucrose solution (IIA) which had been stored for one year, similarly to fresh freeze-dried fruit, sucrose appeared mostly in amorphous form, and therefore was invisible in photographs taken using birefringent interferometry (). The shining crystalline forms which are visible in the photograph () were most probably sugars that naturally occur in fruit tissue, or sucrose crystals ().
Figure 12 Forms of sugar crystals appearing on the surface of a freeze-dried strawberry not subjected to osmotic dehydration (II) after a year's storage. (a) Fragment of the surface layer—zoom 600×; and (b) Crystal of fibrous structure on the surface—zoom 1200×.
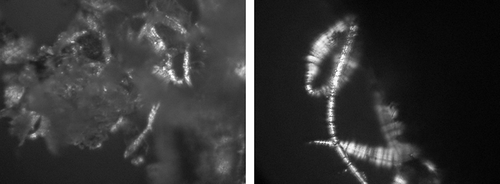
Figure 13 Forms of sugar crystal appearing on the surface of a freeze-dried strawberry osmotically dehydrated in sucrose solution (IIA), after a year's storage. (a) Fragment of the surface layer—zoom 600×; and (b) Typical sucrose crystal on surface—zoom 1200×.
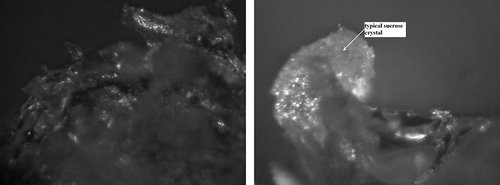
Comparing images 12 and 14, similar degree of surface impregnation in freeze-dried strawberries can be noticed, together with likeness of sugar crystal forms in the fruit deprived of osmotic dehydration (II) and osmotically dehydrated in glucose solution (IIB). For strawberries osmotically dehydrated in a glucose solution, similarly to fresh freeze-dried fruit (), the presence of glowing crystal forms of fibrous structure was confirmed ().
CONCLUSIONS
Osmotic dehydration significantly limited shrinkage of freeze-dried strawberries obtained at heating shelves temperature of 30°C, in comparison to fruit deprived of osmotic dehydration obtained in the same conditions. Furthermore, the shrinkage rate depended on the type of osmotic solution: the smallest volume changes were observed after osmotic dehydration in sucrose and glucose solutions, the differences between the two having been negligible. Osmotic pre-treatment caused almost threefold increase in resistance to compression in freeze-dried strawberries compared to fruit not subjected to osmotic dehydration. The type of osmotic solution, however, was without any consequence for the compression resistance. Osmotic dehydration in a sucrose solution significantly strengthened the structure of freeze-dried strawberries, regardless of the fruit's water activity. At the same time, osmotic pre-treatment in a glucose solution reduced mechanical resistance of freeze-dried strawberries, especially at water activity of 0.225. An increase of water activity from 0.225 to 0.432 for strawberries osmotically dehydrated in sucrose solution caused multiple decreases in compression force. Convective-drying caused distinct structural damage to strawberries. In consequence of cellular wall disruption, large irregular spaces were formed. The residual part of the dried fruit tissue formed a compact substance. Osmotic dehydration of strawberries in a sucrose solution followed by convective-drying caused structural relaxation in dried fruit, and cellular walls were impregnated by sugar. Despite similar sizes of individual cells in both kinds of dried fruit, application of osmotic pre-treatment caused strawberries to have a more porous structure and reduced the degree of their cell damage. Freeze-drying of strawberries deprived of osmotic dehydration at heating shelves temperature of 30°C rendered the structure of the dried material more sensitive, as well as led to the formation of regular cells with thin walls. Osmotic dehydration in a sucrose, or glucose solution, or in a starch syrup noticeably strengthened the structure of freeze-dried strawberries. Essentially, cellular walls were impregnated with sugar, and in effect of osmotic dehydration cells in the surface layer of dried material sustained partial damage. The application of glucose solution allowed us to obtain dried material with (vacant) spaces almost two times smaller than in the case of sucrose solution. As a result of the lower molecular mass of glucose, changes in the surface layer were less destructive, and formed spaces were bigger than in fruit without osmotic pre-treatment. Sucrose penetration from osmotic solution caused cell ruptures in the surface layer, while in the deeper layer large irregular spaces were formed. Also, osmotic pre-treatment in a glucose solution brought the greatest saturation of freeze-dried strawberry tissue. Presence of glucose crystal in fibrous form was observed in the tissue; they ensured greater plasticity of the dried material. Freeze-dried strawberries exhibited high stability during the storage for one year. For fruit deprived of osmotic dehydration and those osmotically dehydrated in a glucose solution, surface impregnation with either natural sugars, or glucose introduced by osmotic solution was observed. Similarly as in fresh freeze-dried fruit, the glucose crystals had fibrous structure. In strawberries osmotically dehydrated in a sucrose solution and stored for one year, similarly as in fresh freeze-dried strawberries, the presence of amorphous sugar forms was observed. The amorphous forms were invisible in the images obtained by birefringent interferometry. Basically, the glowing objects in the images were sugars naturally appearing in fruit tissue or several typical sucrose crystals.
REFERENCES
- Ciurzyńska , A. and Lenart , A. 2006 . Effect of osmotic dehydration on mechanical properties of freeze-dried strawberries. Food. Science . Technology. Quality , 2 : 56 – 67 . in Polish
- Lewicki , P.P. and Drzewucha-Bujak , J. 1998 . Effect of drying on tissue structure of selected fruits and vegetables , Edited by: Akritidis , B. , Marinos–Kouris , D. and Mujumolar , A.S. 1093 – 1097 . Thessaloniki, Hungary : Proceedings of the 11th International Drying Symposium . Drying’98
- Alvarez , C.A. , Aguerre , R. , Gόmez , R. , Vidales , S. , Alzamora , S.M. and Gerschenson , L.N. 1995 . Air dehydration of strawberries: Effects of blanching and osmotic pretreatments on the kinetics of moisture transport . Journal of Food Engineering , 25 : 167 – 178 .
- Lim , L.T. , Tang , J. and He , J. 1995 . Moisture sorption characteristics of freeze-dried blueberries . Journal of Food Science , 60 : 810 – 814 .
- Irzyniec , Z , Klimczak , J. and Michałowski , S. 1995 . Freeze-drying of black currant juice . Drying Technology , 13 : 417 – 424 .
- Raghavan , G.S.V. and Silveira , A.M. 2001 . Shrinkage characteristics of strawberries osmotically dehydrated in combination with microwave drying . Drying Technology , 19 : 405 – 414 .
- Corzo , O. , Bracho , N. and Marval , J. 2006 . Color change kinetics of sardine sheets during vacuum pulse osmotic dehydration . Journal of Food Engineering , 75 : 21 – 26 .
- Adams , J.B. 1991 . Review: Enzyme inactivation during heat processing of food-stuffs . International Journal of Food Science and Technology , 26 : 1 – 20 .
- Jackman , R.L. and Stanley , D.W. 1995 . Perspectives in the textural evaluation of plant food . Trends in Food Science and Technology , 6 : 187 – 194 .
- Ferrando , M. and Spiess , W. 2001 . Cellular response of plant tissue during the osmotic treatment with sucrose, maltose and trehalose solutions . Journal of Food Engineering , 49 : 115 – 127 .
- Barat , J.M.E. , Chiralt , A. and Fito , P. 1998 . Equilibrium in cellular food-osmotic solution systems as related to structure . Journal of Food Science , 63 : 836 – 840 .
- Lewicki , P.P. and Pawlak , G. 2003 . Effect of drying on microstructure of plant tissue . Drying Technology , 21 : 657 – 683 .
- Lewicki , P.P. and Porzecka-Pawlak , R. 2005 . Effect of osmotic dewatering on apple tissue structure . Journal of Food Engineering , 66 : 43 – 50 .
- Lewicki , P.P. and Łukaszuk , A. 2000 . Effect of osmotic dewatering on rheological properties of apple subjected to convective drying . Journal of Food Engineering , 45 : 119 – 126 .
- Lewicki , P.P. and Sitkiewicz , I. 1999 . Effect of pre-drying treatment and storage on rheological properties of dried onion . International Journal of Food Properties , 2 : 23 – 27 .
- Monsalve-Gonzalez , A. , Barbosa-Canovas , G.V. and Cavalieri , R.P. 1993 . Mass transfer and textural changes during processing of apples by combined methods . Journal of Food Science , 58 : 1118 – 1124 .
- Jakubczyk , E. , Sitkiewicz , I. and Lewicki , P.P. 1997 . Mechanical properties of dried apples , 25 – 31 . Miscolc, Hungary : Proceedings of the International Conference of Ph. D. Students, University of Miscolc Press . In
- Litwińska , M. 2003 . The influence of osmotic dehydration on properties of freeze-dried strawberries , SGGW, Warsaw : Master's thesis . in Polish
- Lenart , A. and Dąbrowska , R. 1999 . Kinetics of osmotic dehydration of apples with pectin coatings . Drying Technology , 17 : 1359 – 1375 .
- Ogonek , A. 2005 . Studies under the influence of edible films on mass exchange during osmotic dehydration of strawberries , SGGW, Warsaw : Doctoral thesis . in Polish
- Mazza , G. 1983 . Dehydration of carrots. Effect of pre-drying treatment on moisture transport and product quality . Journal of Food Technology , 18 : 113 – 123 .
- Bakier , S. 2005 . Analysis of the possibility of determining mass fraction of crystalline glucose of honey by the stereological method . Acta Agrophisica , 6 : 295 – 306 .
- Group , work . 2002 . Chosen problem sof statistical interface with the use of STATGRAPHICS packet, Red , Edited by: Grzegorzewski , P. Warsaw : Warsaw Polytechnic School . in Polish
- Krokida , M.K. and Maroulis , Z.B. 1997 . Effect of drying method on shrinkage and porosity . Drying Technology , 15 : 2441 – 2458 .
- Ratti , C. 2001 . Hot air and freeze-drying of high-value foods: a review . Journal of Food Engineering , 49 : 311 – 319 .
- Aguilera , J.M. 1999 . Microstructural principles of food processing and engineering , 2nd , 155 – 178 . Aspen : A Chapman and Hall Food Science Book . ed.
- Szarycz , M. , Kamiński , E. , Jałoszyński , K. and Szponarska , A. 2003 . The analysis of microwaves drying of parsley in conditions of low pressure . Part I. Drying kinetics of unblanched and blanched parsley. Acta Scientarum Polonorum , 2 : 17 – 27 . in Polish
- Shishehgarha , F. , Makhlouf , J. and Ratti , C. 2002 . Freeze-drying characteristics of strawberries . Drying Technology , 20 : 131 – 145 .
- Nieto , A. , Castro , M.A. and Alzamora , S.M. 2001 . Kinetics of moisture transfer during air drying of blanched and/or osmotically dehydrated mango . Journal of Food Engineering , 50 : 175 – 185 .
- Sitkiewicz , I. and Lenart , A. 2002 . The influence of water activity on physical properties of dried fruits osmotically dehydrated . Agriculture Engineering , 5 : 319 – 324 . in Polish
- Erle , U. and Schubert , H. 2001 . Combined osmotic and microwave - vacuum dehydration of apples and strawberries . Journal of Food Engineering , 49 : 193 – 199 .
- Riva , M. , Campologno , S. , Leva , A.A. and Maestrelli , A. 2005 . Torreggiani, D. Structure-property relationships in osmo–air-dehydrated apricot cubes . Food Research International , 38 : 533 – 542 .
- Andreś , A. , Bilbao , C. and Fito , P. 2004 . Drying kinetics of apple cylinders under combined hot air – microwave dehydration . Journal of Food Engineering , 63 : 71 – 78 .
- Jakubczyk , E. and Lewicki , P.P. 2002 . The influence of pre-treatment conditions before drying on mechanical properties of dried apples . Agriculture Engineering , 5 : 431 – 438 . in Polish
- Sitkiewicz , I. , Lenart , A. and Lewicki , P.P. 1996 . Mechanical properties of osmo-convection dried apples . Polish Journal of Food Nutrition Sciences , 5/64 : 105 – 112 .
- Bakier , S. 2004 . Description of phenomena occurring during the heating of crystallized honey . Acta Agrophisica , 3 : 415 – 424 .