Abstract
Polylactide (PLA), a biodegradable aliphatic polyester, has been studied extensively for its wide applications in various fields from food packaging to interior materials for automobiles. One of PLA's advantages is that the raw material, lactic acid can be derived from renewable resources, which makes PLA very attractive for the packaging and considered as green packaging. Although, the cost of PLA is relatively higher compared to petroleum based packaging materials and it is predicted that the price will go down after commercial success of the process. This paper presents a review on polylactide with focus on its chemistry, synthesis, properties, and applications. Plasticization effect on PLA, sorption isotherm and mechanical properties of PLA based packaging materials have been discussed. PLA/layered organo nanocomposites exhibit significant improvement in mechanical, thermal, optical, and physicochemical properties over pure PLA. This review includes brief overview of PLA based nanocomposite, their mechanical properties, potential as packaging materials for industrial usage and their environmental implications.
INTRODUCTION
Lactic acid based polymers are known as polylactides (PLA). PLA are produced either by ring-opening polymerization (ROP) of lactides or by condensation polymerization of the lactic acid monomers, and these monomer are obtained from the fermentation of corn, beet-sugar, cane-sugar etc. PLA has high mechanical properties, thermal plasticity, fabric ability, and biocompatibility.[Citation1,Citation2]
Polylactides have been of significant research interest due to their biocompatibility and biodegradability that leading to applications in medical science and biotechnology. The biopolymer possesses desirable characteristics include their decomposition into naturally occurring metabolites via hydrolysis or enzymatic processes.[Citation3] Over the past decades, the degradation of PLA materials has been studied for practical medical applications such as drug delivery systems, sutures, and surgical implants.[Citation4–6] Polyanhydrides of PLA have been tested for implantations including architecturally fabricated stents that could replace conventional metallic stents.[Citation7] The advantage of polylactide based biopolymers over conventional metallic or non-biodegradable polymers is to ease of removal by the body system itself and retention of shape during time.
PLA are exploring as an alternative solution to solve the ecological problem of plastic waste accumulation,[Citation8–9] with a major focus on packaging. PLA, due to its biodegradation ability, presents the major advantage to enter in the natural cycle implying its return to the biomass. The use of PLA in food packaging has already received significant attention[Citation10–12] and currently, PLA-based packaging and containers including bottled water, juice and yoghurt are used in Europe, Japan and North America for supermarket products.[Citation13] PLA-based packaging materials have been regarded as safe (GRAS) which put it in a unique position for food applications. Furthermore, it has been proposed as a renewable and degradable plastic for uses in service ware, waste-composting bags, mulch films, controlled release matrices for fertilizers, pesticides, and herbicides.[Citation14] All these attributes of PLA lead to development of green packaging which is currently focus of research and demand from common people to environmentalists throughout the world.
To improve the physical properties like mechanical, thermal and electrical of PLA for industrial and commodity applications various types of fillers have been added in nano-scale and the combination between the filler and the PLA is termed as nanocomposite. The addition is commercially advantageous, because the physical properties are readily manipulated by the type and concentration of fillers. PLA possesses a relatively high melting temperature and moderate glass transition temperature (Tg) compared to other biodegradable aliphatic polyester and, therefore, PLA have been experimented for developing nanocomposites.[Citation15] Talc and montmorillonite are cost-effective fillers for PLA to improve crystallinity, thermal stability, and mechanical properties.[Citation16,Citation17] This review will provide brief discussion on various aspects of polylactides starting from the synthesis of PLA from renewable sources to PLA based nanocomposite with major emphasis on food packaging.
WHAT IS POLYLACTIDE?
PLA is a biodegradable thermoplastic derived from lactic acid. Both polylactide and poly(lactic acid) with abbreviation of PLA in fact are the same chemical products; only they differ from each other in means of production. Lactic acid (2-hydroxy propionic acid) is the most widely occurring carboxylic acid in nature. It has a hydroxyl group adjacent to the carboxyl group, making it an alpha hydroxy acid (AHA). Because of chirality, lactic acid has two optical isomers: L-(+)-lactic acid or (S)-lactic acid and D-(−)-lactic acid or (R)-lactic acid. L-lactic acid is the natural and biologically important isomer, but D-form can be produced by microorganisms or by racemization. Stereoisomers of lactic acid are shown in .
Lactic acid is primarily found in fermented milk products, such as yogurt, kefir, leban, and some cottage cheeses. Lactic acid is commercially manufactured by bacterial fermentation process using various substrates like corn, potato, beet, cane sugar, dairy products and even from agricultural waste materials. The common microorganisms used for the process are Bacillus acidilacti, Lactobacillus delbueckii or Lactobacillus bulgaricus. The choice of micro-organism basically depends upon the nature of carbohydrate to be fermented. Details of lactic acid fermentation are available elsewhere.[Citation18]
BRIEF SYNTHESIS PROCESS OF PLA
Poly(lactic acid) is synthesized using a polycondensation reaction starting from lactic acid. The major limitation of this polycondensation reaction is that it results in polymers of low molecular weight. Water generated during the polymerization has to be removed during the polymerization process, and rapidly equilibrium occurs between polymerization and the depolymerization reaction. In addition, this polymerization requires long reaction times in combination with high temperatures. To overcome the limitation, lactic acid is initially oligomerized and catalytically dimerized to produce the cyclic lactide monomer. The high molecular weight polylactide is made from lactide monomer by ring opening polymerization (ROP). Aluminum[Citation19,Citation20] and tin alkoxides[Citation21] are commonly used catalysts for the polymerization of lactides. A major difference between the tin and the aluminum-based catalysts is that tin catalysts are good trans-esterification catalysts, whereas aluminum catalysts are not. Tin catalysts have the advantage of being more hydrolytically stable than those of aluminum and can be easier to handle and use in polymerizations. Tin 2-ethylhexanoate (stannous octoate, or Sn(Oct)2) and lauryl alcohol (1-dodecanol) have been used frequently as initiator and co-initiator, respectively, for the synthesis of PLA homopolymers[Citation22] in relatively short time periods with high conversion and low racemization. This catalyst has been approved by the Food and Drug Administration (FDA) for food contact,[Citation23] making it ideal for many food packaging applications including fruit juices and mineral water. The process does not produce any water and, therefore, a wide range of molecular weight can be achieved by the process. The schematic polymerization reaction and process of lactic acid are illustrated in and , respectively.
The stereochemical composition of the lactide monomer stream can determine the stereochemical composition of the resulting polymer since bonds to the chiral carbons are not broken in the polymerizations. Polymerization of L-lactide produces poly(L-lactide) and polymerization of D-lactide yields poly(D-lactide). Poly(L-lactide) and poly(D-lactide) have identical properties except for stereochemistry. However, racemic (50% D- and 50% L-Lactide) mixture gives poly (DL-lactide), which is an amorphous polymer. In addition, PLA can be produced with varying fractions of L and D lactide.
The unreacted monomer has to be removed by washing with ethanol after polymerization process since the residual monomer in the polymeric matrix can serve as plasticizer and, lower the mechanical strength and thermal stability of PLA. In addition, lactide monomer remaining in the final product can also decrease shelf life. During processing, significant amounts of residual monomer can cause problems such as changes in viscosity and rheological properties.[Citation23]
PHYSICAL PROPERTIES
Thermal Properties
Understanding of the thermodynamic properties of PLA requires quantitative thermal analysis and interpretation of the underlying molecular motion.[Citation24] Because of the chirality of the lactyl unit, lactide exists in three diasteroisomeric forms: L-lactide, D-lactide and meso-lactide as shown in . Among the various polylactides, optically pure polylactides, poly(L-lactide) (PLLA) and poly(D-lactide) (PDLA), are crystalline polymers with a melting point around 180°C.[Citation25,Citation26] The atactic polymer, poly-(DL-lactide) is an amorphous material with a glass transition temperature of 50–57°C.[Citation21,Citation26] It is well established that the properties of polylactides vary to a large extent depending on the ratio and the distribution of the two isomers and molecular weight of the polymer.
There has been renewed interest in crystallization of lactides, recently. Nature derived lactides (agricultural source) are mostly in L-form and exhibit crystalline behavior. The crystallization of behavior of polylactides depends on the thermal history (i.e., annealing),[Citation27] amount and type of additives,[Citation28] and stereo sequence distribution.[Citation16] In addition, crystallization depends upon optical purity. It is reported that at least 72% threshold optical purity in composition is required to obtain crystallanity of polylactides.[Citation16,Citation29] Semi-crystalline nature of PLA has been observed during cooling of melt.[Citation30] The heat of crystallization measured by differential scanning calorimetry may depend on the sterosequence distribution in the polymer. It is also important to know the percent of crystallinity since it strongly influences the physical properties of the polymer.[Citation31]
Thermal analysis of a series of polylactides (PLA) based on number average molecular weight (Mn), and the nature of isomer (D, L and DL) was reported by Ahmed et al.[Citation26] It indicated that the glass transition temperature (Tg) of PLA increased as function of molecular weight irrespective of isomer type except sample with high polydispersity index (). The melting temperature (Tm) and enthalpy of crystal fusion (ΔHf) of L-isomer increased as Mn was increased from 1100 to 27500. The degree of crystallinity (χc%) increased as function of molecular weight however, no crystallization peak was detected in the lower molecular weight range (550–1400). The non-isothermal crystallization behavior of the PLA melt was significantly influenced by the cooling rate. Both D and L isomers exhibited insignificant difference in thermal properties and DL lactides exhibited amorphous behavior at identical molecular weight. Change in microstructure showed significant difference between two isomers. Typical thermograms for L and DL-PLA are presented in .
Table 1 Effect of isomer on thermal properties of PLA
Rheological Properties
Rheological properties are important tools in evaluating thermoplastics for their performance during processing operations and, therefore, understanding of melt rheology of PLA is crucial to in order to facilitate effective processing. Intrinsic factors affecting the flow characteristics of PLA are molecular weight distribution, degree, and type of branching, optical composition, optical block length distributions, and melt stability. PLA is a pseudoplastic, non-Newtonian fluid. Above the melting point, PLA behaves as a classic flexible-chain polymer across all optical compositions.
Generally the melting temperature (Tm) for high molecular weight PLA is about 170°C and it is worth to study melt rheology above 170°C.[Citation32] Rheograms of PLA containing 100% L-form (MW = 750000 g/mol) at 180°C exhibit plateau region at higher frequency range. Results for high molecular weight samples suggest that the plateau modulus is approximately 5 × 105 Pa. In addition, the zero shear viscosity, (ηo), for PLA is found to roughly scale with the expected 3.4 to 4 power with molecular weight.[Citation32,Citation33]
Flow viscosity measurement of amorphous and semi crystalline PLA (molar mass of 88000 Da) at 150 and 170°C and at various shear rates (30–150 rpm screw speeds) using a tube rheometer on an extruder indicated semi crystalline PLA had a higher shear viscosity (3530 Pa.s) over amorphous PLA (3340 Pa.s).[Citation14] It is reasoned that the molecules of semi crystalline PLA were arranged in organized pattern, which provided stronger intermolecular forces and relatively greater resistance to flow. On the contrary, the arrangement of the molecules of amorphous PLA was random, which in turn exhibited less resistance to flow. In general, materials with crystalline structures have stronger physical and mechanic properties than amorphous materials.
PLA behaved as a true viscoelastic fluid. The viscosity and the first normal stress difference in steady shear flow were estimated using the Cox-Merz rule and Laun's empirical relation, respectively. The latter relation relates the steady state shear flow to the dynamic storage and loss modulus. The dynamic and steady shear viscosities of PLA melt superimposed with each other and confirming applicability of the Cox-Merz rule.[Citation32,Citation34] As the temperature increased, the shear viscosity decreased for both types of PLA. The PLA melt was characterized as a pseudoplastic, non-Newtonian fluid. Power law equations described well the behavior of the PLA melts.
The solution viscosities of polylactides is usually measured in chloroform known as intrinsic viscosity (dL/g) with an Ubbelohde viscosimeter at 30°C and used for molecular weight calculation using Mark-Houwink relationship[Citation35] as shown below:
Thus, log-log plots of [η] against molecular weight (M) have the intercept log(K) and slope a. The K and a value for PLA were found to be 5.45×10−4 and 0.72, respectively. The slope (a) contains information about the shape of the molecules.
Infrared Spectroscopy
Fourier transform infrared spectroscopy (FTIR) has been used extensively for PLA studies for its high sensitivity and low cost. The instrumentation is readily available allowing on-line measurements during film processing as well as in situ monitoring of polymerization reactions in conjunction with a remote attenuated total reflectance (ATR) probe. The FTIR has been used for detection of unreacted monomer (at 934 cm−1), conversion analysis, lactide concentration determination and crystallinity studies of PLA.[Citation34,Citation35] Degée et al.[Citation36] used the ratio of the absorbance of the peak at 935 cm−1 to the absorbance at 1383 cm−1 to establish a calibration plot independent of the absolute sample concentration. This calibration curve was subsequently used in their study to analyze lactide conversion during reactive extrusion as a function of time.
Braun et al.[Citation35] used FTIR spectroscopy for measuring lactide concentration in a polylactide (PLA) matrix. The lactide ring breathing mode peak at 935 cm−1 is rationed against the asymmetric bending mode of methyl groups at 1454 cm−1; because the methyl group is present both in lactide monomer and the polymer, this ratio provides a direct measure of lactide concentration. Using well-defined mixtures of lactide and PLA, a calibration curve is established. For lactide concentrations corresponding to conversions above 75%, a linear relationship between conversion and the peak area ratio exists. Below 75% conversion, the development of a double peak at 1454 cm−1 leads to deviations from linearity; data can then be accurately represented by a second-order polynomial relating conversion to the peak area ratio.
Sarusa et al.[Citation34] observed a shift to lower wave numbers in the C = O stretching band of polylactides in the stereocomplex with regard to that in the homocrystal. This band shift is accompanied by similar displacements in the C-H spectral bands. These results are interpreted in terms of H-bonding forces causing specific CH3 … O = C and CαH … O = C interactions between both stereoisomers of polylactide.
There are numerous studies on FTIR to solid-state PLA deal with its crystalline structure.[Citation37] The major focus of IR studies is on the spectral changes observed in the carbonyl (C = O) stretching and in the ester (C-O-C) asymmetric stretching regions. From PLA crystallinity point of view, the interesting band of the FTIR spectra ranges between 1300 and 1150 cm−1 (). The spectrum of both D and L isomer of PLA are almost identical. Bands at 1269, and 1215–1186 cm−1 are known as crystalline bands in PLLA structures. These consist of vibrational components, i.e., C-COO stretching, O-CH stretching, CH3 rocking, and CH bending.[Citation38] Both D and L samples exhibited a shoulder between 1240 and 1200 cm−1 that clearly distinguished from the DL sample where the shoulder was not present. A small shift of the crystalline band might be affected by preparation of sample, molecular weight and other factors. The absence of crystalline band in low molecular weight sample between 1215 and 1186 cm−1 justifies the absence of crystallanity. These observations are duly supported by earlier FTIR work on PLA samples.[Citation39]
APPLICATIONS OF PLA
Food Packaging
Present global concern on petrochemical based plastic materials [polyethyleneterephthalate (PET), polyvinylchloride (PVC), polyethylene, polystyrene (PS), polyamide (PA)] has created much interest in biodegradable or green packaging materials. Biodegradable plastics have matured enough from its infancy and polymers with true susceptibility with microbial degradation are reality today. The compostability attribute is very significant for biopolymer materials because while recycling is energy expensive, composting allows disposal of the packages in the soil by transforming into water, carbon dioxide and inorganic compounds.[Citation40] Biodegradation standards assess the propensity of a material to degrade biologically. Few years back, the US Composting Council and the Biodegradable Products Institute have initiated certification to those materials suitable for composting following ASTM guidelines (D 6400-99). According to the guidelines, ‘Biodegradable Plastic’ is defined as a degradable plastic in which the degradation results from the action of naturally occurring microorganisms like bacteria, fungi and algae (ASTM D 6400-99).
Biodegradable or green packaging must accomplish basic requirements to be an ideal candidate for food following traditional packaging, which includes barrier properties (water vapor, gases, light, and aroma), optical properties (transparency), strength, welding and molding properties, printing properties, migration resistant, chemical, and temperature resistance properties, disposal requirements, antistatic properties, retaining sensory properties, and above all strictly follow food safety.
Poly(lactide) (PLA) have gained growing attention in the last one decade as food packaging materials because they can easily be obtained from renewable resources; their production consumes quantities of carbon dioxide; they can be recycled and composted; and their physical and mechanical properties can be tailored through polymer architecture.[Citation10,Citation11,Citation41] Although PLA production costs are relatively high over conventional plastics, there are predictions that this will change with time as production volumes and demand both increase.[Citation42]
Some of the beneficial characteristics of PLA fiber products include its natural soft feel, ease of processing, and unique stain and soil resistance. PLA has already been approved for its intended use in fabricating articles for contact with food by the Food and Drug Administration (FDA). PLA is already being exploited for short shelf-life food packaging like containers, drinking cups, sundae and salad cups, over-wrap and lamination films, and blister packages.[Citation43] PLA-based packaging materials are currently used for supermarket products in Europe and North America for packaging bottled water, juices, and yogurts (; ). The containers meet German and EU food standards. PLA excels at resistance to stain in standard tests with coffee, tea, cola, catsup and other food products. Therefore, PLA is gradually moving towards a ‘green’ food packaging material.
Table 2 Potential food application of PLA based packaging materials
Food industry and consumers' are looking for food that can retain aroma and freshness after processing. Novel processing technologies (e.g., high-pressure processing, pulse-electric heating, Ohmic heating, and osmotic dehydration) have generated interest to fulfill the vacuum. Definitely, there is a need to develop food packaging materials suitable for those novel technologies. Recently, Ahmed et al.[Citation46] have studied suitability of PLA based packaging materials under high-pressure processing environment. Effect of high-pressure treatment (350, 450, and 650 MPa for a holding time of 15 min) on thermal properties of amorphous and semi-crystalline polylactides (D, L and DL) revealed that the Tg decreased when pressure was applied to lactides. However, both melting and crystallization peak of L isomer were significantly reduced at 650 MPa and the observation was quantified by decrease in fusion (ΔHm) and crystallization enthalpy (ΔHc). A typical pressure effect on PLA crystallinity is shown in . This study suggests that PLA could be further experimented for development of food packaging material under high pressure treatment either individually or in combination with other materials.
Mechanical Behavior and Plasticization of PLA
Mechanical and barrier properties of PLA have been studied extensively.[Citation47,Citation50] Mechanical parameters indicated that PLA is a brittle material (tensile strength = 32 MPa) with high Young modulus (2.3 GPa) and low percentage elongation of break (EB) (5%).[Citation49] The tensile strength values obtained for L-polylactides are similar to PS under the same testing conditions but lower than PET.[Citation47] The melting temperature (Tm) and glass transition temperature (Tg) of PLA are significantly lower than PET and PS (), which improve heat sealing and thermal processing capabilities of PLA. The barrier properties of PLA revealed that both O2 and CO2 permeability coefficients are lower than PS but analogous to those of PET (). Interestingly, the water vapor permeability coefficients of PLA do not vary significantly with relative humidity although PLA polymers are polar in nature. The decrease in water permeability coefficient of PLA with increasing temperature has added another advantage of PLA for potential use in multilayer structures to counterbalance, at least in part, the loss of water barrier with temperature shown by most polymers.
Table 3 Comparison of thermal properties (heating rate 10°C/min) of PLA and synthetic polymers.Footnote*
Table 4 Comparison of water vapor and oxygen transmission rate of PLA and synthetic polymers.Footnote*
Since PLA is rigid and brittle with low ability to plastic deformation below glass transition temperature (Tg) (50–60°C); it is necessary to plasticize PLA in order to produce flexible films.[Citation51] Various polymers are reported in the literature for efficient plasticizing for PLA including poly(3-methyl-1,4-dioxan-2-one), poly(ethylene oxide), citrate esters, triacetine, and poly(ethylene glycol)s (PEGs). The transition from brittle to ductile behavior in the plasticized polylactide occurs when Tg is shifted to 35°C.[Citation52] Martino et al.[Citation49] have evaluated four different adipate based plasticizers (Di-2Etylhexyl Adipate and three commercial polymeric adipates with different viscosities) for making PLA based films. Incorporation of plasticizer to PLA films has lowered the Tg significantly. At highest level of plasticizer concentration, the Tg value of the blend reached minimum.
Kulinski et al.[Citation52] used poly(propylene glycol) (PPG) as a plasticizer for PLA having Mw of 530 and 1123 g/mol at 5–12.5 wt % and compared with a similar Mw (578 g/mol) of polyethylene glycol (PEG). The advantage of PPG is that it does not crystallize, has low Tg, and is easily miscible with PLA. The plasticizers decreased Tg from 55.7°C for neat PLA to 43–45, 38–39, 31–34, and 27–32 for the blends with 5, 7.5, 10, and 12.5 wt % of plasticizers, respectively. Tg of blends of PLA/PPG and PLA/PEG with similar Mw was alike however lower than Tg of PLA/PPG with higher Mw blends. For neat PLA, the crystallization and melting temperature were recorded at 119 and 134°C, respectively. With the increase of plasticizer content in a blend, the crystallization peak temperature decreased to 104°C and the corresponding melting temperature dropped to 130°C. Both PPGs enhanced the crystallizability of PLA although less than PEG. The plasticizers improved the ability of PLA to plastic deformation, which was reflected in a decrease of yield stress and in an increase of elongation at break. The effect was facilitated by the higher PPG content and by lower molecular weight of PPG.
Neat PLA yielded at the deformation of about 5% and the stress of 41 MPa and exhibited some ability to plastic flow; the average elongation and stress at break were around 64% and 26 MPa, respectively. The yield stress decreased for all of the blends compared to neat PLA. The elongation at break exceeded that of neat PLA beginning with the plasticizer content of 7.5 wt % and reached 500–700% for the plasticizer content of 12.5 wt %. The stress at break of the blends, in the range of 17.5–22.8 MPa, was generally lower than that of neat PLA, 25.5 MPa; its relatively high values for the blends with 10 and 12.5 wt % of plasticizers resulted from the strain hardening duly supported by stress-strain dependencies.
Van Aardt et al.[Citation53] studied rates of release of antioxidants from antioxidant-loaded PLA based films into water, oil and dry milk products. Poly(lactide-co-glycolide) (PLGA) (50:50) films loaded with antioxidants, [2% α-tocopherol, or a combination of 1% butylated hydroxytoluene (BHT) and 1% butylated hydroxyanisole (BHA)], were used in water and oil at 4 and 25°C. In water medium, PLGA (50:50) showed hydrolytic degradation of the polymer, and release of BHT into water. Polymer degradation did not take place when antioxidant-loaded films were stored in oil, whole milk powder (3.01% moisture) and buttermilk powder (4.60% moisture). However, α-tocopherol, BHA and BHT were released through diffusion from 0 to 21.9, 0 to 60.0, and 0 to 192.0 mg.kg−1 milk fat, respectively, in whole milk powder after four weeks of storage at 25°C. Pentanal, and not heptanal or hexanal, was significantly decreased when buttermilk powders were packaged in contact with BHA/BHT-loaded PLGA films. Potential use of degradable polymers as unique active packaging options for sustained delivery of antioxidants could be a benefit to the dairy industry by limiting the oxidation of high-fat dairy products.
One recent study suggests that the incorporation of bacteriocins into PLA polymer could provide a possible delivery system for improving the efficacy of bacteriocins in food applications.[Citation13] In this study, only PLA/nisin films were evaluated. However, the PLA/nisin polymer can be used to make bottles or coated on the bottle surface for use in liquid food packaging, as well as being made into films or coated on the surface of films for use in solid food packaging.
Moisture Sorption of PLA
For biodegradable packaging materials, the sorption and the diffusion of low molecular components in the materials play an important role. Some modifications of polymers by swelling, hydrolysis or plasticization, can result from the sorption of water, which is easy due to presence of polar and hydrophilic groups. Although the importance of moisture penetration in evaluating the performance, degradation, and life cycle of polymer products, there are limited literatures available on moisture transport in polylactides (PLA) and blends or copolymers of PLA for packaging applications. A typical sorption isotherm at 20°C is presented in . It clearly indicates water absorption increase significantly above 60% RH.
Diffusion coefficients of water in PLA and other biopolymers were measured by gravimetric techniques and changes in solubility were correlated with hydrophobicity of biopolymers and related to formation of clusters of water molecules. Sharp et al.[Citation54] studied the water uptake of poly (DL-lactide) of 50:50 copolymer of PLA with glycolic acid by means of Quartz Crystal Microbalance (QCM), relative humidity was controlled within the sample chamber by using saturated salt solution. (The Quartz Crystal Microbalance (QCM) is a very sensitive tool to detect changes in weight () and therefore used as a helpful method to sense adsorption processes at solid/gas or solid/liquid interfaces. The basis of the QCM is thin quartz exhibiting the inverse piezoelectric effect. Applying an alternating current excites a mechanical oscillation of the quartz plate. Using the specific resonance frequency of the quartz leads to a direct proportionality of the mass load and the frequency change.) It was found that the solubility of water in a homopolymer is significantly higher than the copolymer, and that, with an increase in Mn, there is a slightly higher sorption in the polymer. Sharp et al[Citation52] also found that increase in vapor activity causes an increase in the initial rate of sorption, however on the contrary, also causes an increase in the time required to reach equilibrium. DSC measurement indicated a decrease in Tg of the polymer film with an increase in the moisture sorption due to plasticization, which should increase the rate of diffusion through the film, thus reducing the decrease in the sorption rate over time.
Figure 10 Quartz Crystal Microbalance used for sorption studies of PLA. (Figure provided in color online.)
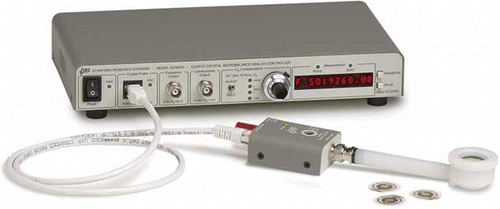
Auras et al.[Citation55] measured water vapor permeability in PLA at various temperatures and relative humidities and interestingly the permeability was found to be independent of relative humidity and decreased with increasing temperature. In another study, the effect of moisture sorption on stability of PLA films at selected relative humidity (11–98% RH) and temperature (5 and 25°C), was investigated by Holm et al.[Citation56] The number average molecular weight (Mn) and tensile strength decreased as humidity and temperature were increased. Hydrolysis of the PLA ester linkages resulted in a 75% decrease over 130 d at 25°C and 98% RH. At lower temperatures, the decrease amounted to 35%. An equilibrium moisture sorption isotherm could not be achieved as the irreversible hydrolysis of PLA induced an ongoing moisture uptake. The rate increased when the humidity and temperature increased from 5°C to 25°C. After 189 d at 98% RH, moisture sorption was found to be 7 g and 86 g per 100 g at 5°C and 25°C, respectively. A minimum loss of tensile strength was justified by reversible plasticization by moisture. Only at 98% RH and 25°C, loss of tensile strength became distinct (45%). Consequently, the PLA material is in general expected to be mechanically stable when packaging foods covering the region from dry to moist food and storage conditions from chill to ambient temperatures.
Shogren[Citation57] observed that PLA permeability to water decreases with increasing temperature although permeability to both CO2 and O2 increased with temperature. Auras et al.[Citation58] have carried out gravimetric sorption tests to estimate the diffusion (D), solubility (S) and permeability (P) coefficients for ethyl acetate and d-limonene in PLA films. For ethyl acetate, values of P = 1.22 × 10−17 kgmm−2 s−1 Pa−1, D = 2.63 × 10−15 m2 s−1, and S = 4.62 × 10−3 kgm−3 Pa−1) at 45°C and a partial pressure of 12 654 Pa were obtained. For d-limonene, no trace was detected after 21 days of testing at 45°C and 258 Pa, which indicates a permeability coefficient lower than 9.96 × 10−21 kgmm−2 s−1 Pa−1. These data indicated PLA film has good aroma barrier to ethyl acetate and d-limonene. The low ethyl acetate and d-limonene permeability coefficients for PLA are an indication that PLA is not likely to allow flavor loss of these and similar chemical compounds by either permeation or scalping in a packaging application. Therefore, PLA could likely be used in applications for citrus based juices and cleaning products.
Singh recently[Citation59] did a systematic study on moisture sorption in PLA as function of molecular weight and aliphatic content via end group modification. Moisture sorption in PLA was measured with Quartz Crystal Microbalance (QCM). Initial experiments of QCM have shown that by increasing the aliphatic content of the end group there was a significant decrease of up to 15% in the moisture sorption of water in PLA.
Influence of the PLA crystallinity on the thermal and barrier properties (oxygen, helium, ethyl acetate) of film indicated that the degree of crystallinity have no effect on the helium and oxygen barrier properties while the aroma barrier properties remain under investigation.[Citation60] The Tg diminishes after the aroma sorption. Therefore, ethyl acetate has a plasticizing effect on the PLA regardless the degree of crystallinity.
Authors have carried out sorption isotherm effect on melting and crystallization of PLA based (polyethylene glycol and starch) film and found there was no significant effect on melting (Tm) and crystallization temperature (Tc) and their corresponding enthalpies. Effect of plasticization of PLA during sorption on glass transition temperature (Tg) was not distinct as melting curve for polyethylene glycol predominated over Tg of PLA.
Lactide Migration
As PLA-based packaging materials comes in contact with food materials and one can expect migration of decomposed PLA components during storage since lactide is progressively decomposed under water-rich conditions. The major migrants from PLA to are lactic acid, lactide and oligomers. Few studies have been reported on the migration of PLA into food materials and food safety. During lactide migration from PLA, it is necessary to measure simultaneously the lactic acid and oligomers in migration solution, and to consider them as these total amounts.[Citation61] Authors found the presence of lactic acid, lactide and oligomers in PLA migration solutions. Further, PLA was stable at 40°C over 6 months whereas, the polymer was decomposed at 60°C or above the glass-transition temperature and the migrant levels increased. The migration was noteworthy in PLA that contained high levels of D-lactic acid. The molecular weights of the PLA sheets were measured after the migration tests, and the degradation of the PLA was obvious.
With regard to lactic acid toxicity, the Joint FAO/WHO Expert Committee on Food Additives (JECFA) recommended that neither D-lactic acid nor (DL)-lactic acid should be used in infant foods (World Health Organization (WHO) 1974). The toxicity of lactide was previously investigated by two- and 13-week studies on beagles. The findings revealed the presence of gross and microscopic lesions indicative of irritation, and suggested a no observed adverse effect level (NOAEL) of 100 mg kg/body weight/day.[Citation62]
Nanocomposite
To enhance barrier and mechanical properties, PLA has been incorporated with other synthetic polymers or mixed with fillers for cost reduction and/or performance improvement (e.g., with protein or starch, inorganic fillers, and natural fiber flax) or, chemically modified with the aim of extending their applications in more special or severe circumstances.[Citation63,Citation64] The use of nanoscale fillers is leading to the development of polymer nanocomposites and represents a better alternative to these traditional polymer composites.[Citation65–66]
Last few years, polymer nanocomposites have emerged as a new class of materials and attracted researchers from almost all disciplines. Polymer/layered silicate nanocomposite exhibit significant improvement of mechanical, thermal, optical, and physicochemical properties over pure polymer or conventional composites. Such nano-hybrid composites possess very unusual properties, different from their microscale counterparts. These include high moduli, increased strength and heat resistance, and decreased gas permeability and flammability.[Citation15] Nanocomposites also provide additional benefits like low density, transparency, good flow, better surface properties and recyclability. The application of nanocomposites promises to expand the use of edible and biodegradable films.[Citation67–68] As far as food safety is concerned, nano-structured materials will prevent the incursion of micro-organisms. Embedded nano-sensors in the packaging will aware the consumer if a food has been spoiled or not.
Nanocomposite Preparation
In general, polymer nanocomposites are made by dispersing inorganic or organic nanoparticles into either a thermoplastic or thermoset polymer. Nanoparticles can be three-dimensional spherical and polyhedral nanoparticles (e.g., colloidal silica), two-dimensional nanofibers (e.g., nanotube and whisker) or one-dimensional disc-like nanoparticles (e.g., clay platelet). Such nanoparticles offer enormous advantages over traditional macro- or micro-particles (e.g., talc, glass, and carbon fibers) due to their higher surface area and aspect ratio, improved adhesion between nanoparticle and polymer, and lower amount of loading to achieve equivalent properties.[Citation69] Details of clay based nanocomposites are available in a review article authored by Zeng et al.[Citation69]
There are different techniques to make clay-based polymer nanocomposites, including: in-situ polymerization, solution exfoliation, and melt intercalation. The most successful technique to prepare polymer/layered silicate nanocomposites is to intercalate polymers into the silicate galleries.[Citation15] Usually, intercalation of polymer chains into silicate galleries is prepared by either: insertion of suitable monomers in the silicate galleries and subsequent polymerization () or direct insertion of polymer chains into the silicate galleries from either solution or the melt. Basically, the process involves the annealing and mixture of polymer and layered silicate nano-materials above the softening temperature of the polymer. During annealing, the polymer chains from the melted bulk diffuse in to the galleries of silicate galleries.
PLA based nanocomposites are prepared by both solvent casting and melt processing techniques. Solvent casting of mixtures of PLA and organophilic clay in chloroform resulted in materials with an enhanced crystallization tendency and increased Young's modulus.[Citation70] However, the glass transition temperature increases only slightly with increasing clay content. This may be due to the micro-composite structure rather than nanocomposite structure. Bandyopadhyay et al.[Citation71] prepared PLA-clay nanocomposites with much improved mechanical and thermal properties.
Extrusion is the mostly used for making nanocomposites followed by film making or other uses. The PLA/layered silicate nanocomposites, prepared by simple melt extrusion of PLA and organically modified montmorillonite, exhibited remarkable improvement of material properties in both solid and melt states compared to the matrix without clay.[Citation15] Incorporation of montmorillonite (3 to 7%, w/w) to PLA does not influence Tg whereas Tm increased from 168 to 170°C (). The crystallinity (%) shows non-systematic behavior with inclusion of clay. The elastic modulas of composite increased significantly with incorporation of nanocomposite. The internal structures of the nanocomposites in the nanometer scale were observed through TEM analysis, which allows a qualitative understanding of the internal structure through direct visualization. On the other hand, WAXD offers a convenient method to determine the interlayer spacing of the clay layers in the original clay and in the intercalated polymer/clay nanocomposites.
Table 5 Thermal and rheological data of nanoclay incorporated PLA composite
Maiti et al.[Citation72] prepared a series of PLA/layered silicate nanocomposites with three different types of pristine layered silicates, i.e., saponite, MMT, and synthetic mica, and each was modified with alkylphosphonium salts having different chain lengths. Their study showed that miscibility of an organic modifier (phosphonium salt) and PLA is enhanced with a higher chain length of modifier. They also studied the effects of dispersion, intercalation and aspect ratio of the clay on the material properties.
Plackett et al.[Citation42] evaluated inclusion of nanoclay in PLA for use as films to improve film barrier properties on packaged cheese. Combinations of PLA and nanoclay (5% w/w) were prepared by melt extrusion as a possible route to films with the required barrier properties. The neat PLA films were highly transparent in the visible spectrum (∼400–800 nm). A minor reduction in light transmission was noticed for films containing nanoclay at the 5% w/w level. However, the differences were insignificant. Nanoclays introduced into the films has enhanced barrier properties resulted in a further increase in moisture sorption. Films made from different ratios of L and D-isomers of PLA and placed at high relative humidity environment has indicated that less moisture is absorbed in pure L-form films compared to poly(D, L-lactide) films and associated lower weight loss is expected. Therefore, using a crystalline PLA packaging material for foods is preferable in terms of moisture sorption. A proprietary impact modifier was found to be effective in reducing the brittleness of the PLA to that of a conventional amorphous packaging film.
Zhang et al.[Citation73] studied blends of soy protein (as an isolate, SPI, and concentrate, SPC) and a semi-crystalline polylactide (PLA) (SP to PLA ratio 30:70 to 70:30 w/w). Blends were extruded using a twin-screw extruder. The compatibility was improved by adding poly(2-ethyl-2-oxazoline) (PEOX) in the blends, and the resulting blends showed an obvious increase in tensile strength and a reduction in water absorption for SPI/PLA blends. Rheological study revealed that there were strong molecular interactions in the melts of the blends attributed to the SP. The blend exhibited significantly higher viscosity over neat PLA, especially at low shear rates. Phase morphology, DMA results, and mechanical properties indicated that SPC had a higher compatibility with PLA than SPI, which resulted in improved phase structures and higher mechanical properties of SPC/PLA blends. The mechanical properties of SPC/PLA blends were greatly enhanced with the increasing PLA contents, while the mechanical properties of SPI/PLA blends established only limited increases. The PLA component in the blends was found to be highly amorphous in injection molded articles due to the rapid cooling in the mold. SP induced and accelerated cold crystallization of PLA in the blends, resulting in a higher PLA crystallinity. This suggests that mechanical properties of the blends could be manipulated under post-process thermal treatments to suit different applications.
Biomedical Application
Synthetic polymers have been used in the field of biomedical application for several decades due to its biocompatibility. Biocompatibility is a widely discussed expression. The most common definition of biocompatibility is “the ability of a material to perform with an appropriate host response in a specific application.”[Citation74] There are number of requirements that need to be fulfilled for a material to be considered as biocompatible. The material has to be non-toxic, non-cancerogenic, non-mutagenic, non-allergenic, and free from contaminants. Also, the material has to have no adverse immunological responses and harmless degradation products.
Initially, biostable polymers were used, and later on, degradable polymers were introduced in the 1960s when PLA was used as a material for tissue replacement.[Citation75] In the early 1970s, controlled drug delivery system has attracted increasing attention with the aim of achieving the controlled, systematic, or site-specific drug release over an extended period of time. This technology has numerous advantages over conventional therapeutic systems, by prolonging duration time, reducing side effects, retaining drug bioactivity, and thus improving the therapeutic efficiency.[Citation76] A powerful approach for controlling drug delivery is to incorporate the drug into biodegradable polymeric nano or microparticles, which can achieve a controlled and sustained fashion through the drug diffusion or/and the polymeric carrier degradation. The advantages of nano- or microparticle drug delivery systems are the injectable property, which can avoid the inconvenient surgical insertion, and the possibility to achieve passive targeting when their sizes are in particular ranges.[Citation77,Citation78]
However, PLA is hydrophobic in nature and while the size of their drug delivery systems reduces to the nanometer range, the nanoparticles are thermodynamically unstable and have a tendency to aggregate. Moreover, the hydrophobic nanoparticles are usually eliminated by the reticuloendothelial system after intravenous injection. To overcome these limitations, nanoparticles formed by the self-assembling of amphiphilic polymers are presently studied as drug carriers.[Citation79] The amphiphilic nanoparticles exhibit thermodynamic stability in aqueous solutions and also increased blood circulation time, which is critical for effective drug delivery. Compared with the block copolymers, amphiphilic graft copolymers can easily form micelles because of the possibility of forming micelles within one or several polymer chains. Moreover, since graft copolymers have multi grafted branches, the hydrophilic/hydrophobic balance can be readily controlled by adjusting the relative grafting density.[Citation80]
Recently, bioresorbable polymeric stents have attracted much attention as alternatives to metallic stents. Bioresorbable polymeric vascular stents have the potential to remain in situ for a predicted period of time, keeping the vessel wall patent and then degrading to nontoxic substances. Accumulating evidence indicates that the use of a bioresorbable coronary stent dramatically decreases the need for prosthesis after six months.[Citation81] Bioresorbable stents are preferable for treatment of tracheomalacia in newborns and infants because removal surgery is not necessary. Moreover, bioresorbable stents can be used as support devices as well as platforms for drug and protein delivery to the conduit wall in all of the above-mentioned applications. Some commercial companies like Bioabsorbable Therapeutics, Inc. (BTI), a California based company has developed and utilized novel, proprietary anti-inflammatory polymers to create fully bioabsorbable coatings and stents. BTI's polymer-called Salix™-is itself therapeutic. Salicylic acid, the active ingredient in aspirin, is incorporated into the backbone of Salix. As Salix degrades, salicylic acid is released and taken up by the artery wall to reduce inflammation and restenosis. The Salix polymer maintains strength to provide excellent vessel scaffolding and resistance to recoil when used to create a completely bioabsorbable stent. Useful combination of copolymers and blends can be created to alter the mechanical properties and drug-release profiles of bioactive agents from polymeric structure based on selected polymers. For example, a combination of poly(glycolic acid) and poly(lactic acid) could produce a desirable mechanical strength and modulas as shown in . The scope of PLA for biomedical applications is enormous and there are plenty of literatures on the subject, which is available elsewhere. Due to limitation of the subject in the present journal, biomedical applications are not explored in this review.
Table 6 Characteristics of typical bioresorbable PLA based polymers
CONCLUSION
For biodegradable packaging materials to compete with conventional synthetic polymers, the critical mechanical, thermal, and barrier properties for the intended application must be comparable. The nanocomposite concept shows a motivating way for creating new and innovative materials including biodegradable polymers. PLA based biodegradable nanocomposites have been prepared with improved mechanical, barrier optical, and thermal properties and can be used in the application of food packaging adequately. Nanocomposites can also be intended to be used as a carrier of antimicrobials, drug delivery and additives. Recent studies have demonstrated their ability to stabilize the additives and efficiently control their diffusion into the food system. This control can be especially important for long-term storage of food or for imparting specific desirable characteristics, such as flavor, to a food system. Although there are numerous possibilities existing for packaging in bio-based nanocomposite material, the low level of production, some property limitation, and high costs confine them for a wide range of applications. Therefore, improvements in nanocomposite formulation, production practices, economies of scale, and increasing costs for fossil resources could all be necessary to produce a more favorable economic situation for biodegradable polymers.
REFERENCES
- Fetters , L.J. , Lohse , D.J. , Richter , D. , Witten , T.A. and Zirkel , A. 1994 . Connection between polymer molecular weight, density, chain dimensions, and melt viscoelastic properties . Macromolecules , 27 : 4639 – 4647 .
- Tullo , A. 2000 . Plastic found at the end maize . Chem. Eng. News , 78 : 13
- Saltzman , W.M. 2001 . Drug Delivery: Engineering principles for drug therapy , 334 – 336 . New York : Oxford University Press .
- Arshady , R. 1991 . Preparation of biodegradable microspheres and microcapsules: Polylactides and related polyesters . J. Control. Rel. , 17 : 1 – 22 .
- Tams , J. , Joziasse , C.A.P. , Bos , R.R.M. , Rozema , F.R. , Grijpma , D.W. and Pennings , A.J. 1995 . High-impact poly(L/D-lactide) for fracture fixation: in vitro degradation and animal pilot study . Biomaterials , 16 : 1409 – 1415 .
- Ikada , Y. , Shikinami , Y. , Hara , Y. , Tagawa , M. and Fukuda , E. 1996 . J. Biomed. Mater. Res. , 30 : 553
- Varshney , S.K. , Hnojewyj , O. , Zhang , J. and Rivelli , P. Polyanhydride Polymers and Their Uses in Biomedical Devices . US Patent 7,674,285 B2 . 2010 .
- Vert , M. , Schwarch , G. and Coudane , J. 1995 . Present and future of PLA polymers . J Macromol Sci; Pure Appl. Chem. , A32 ( 4 ) : 787 – 796 .
- Hyon , S-H , Jamshidi , K. and Ikada , Y. 1997 . Synthesis of polylactides with different molecular weights . Biomaterials , 18 : 1503 – 1508 .
- Sinclair , R.G. 1996 . The case for polylactic acid as a commodity packaging plastic. J. Macromolecular Sci . Part A: Pure and Applied Chemistry , 33 : 585 – 597 .
- Haugaard , V.K. , Weber , C.J. , Danielsen , B. and Bertelsen , G. 2002 . Quality changes in orange juice packaged in materials based on polylactate . Eur. Food Res. Technol. , 214 : 423 – 428 .
- Frederiksen , C.S. , Haugaard , V.K. , Poll , L. and Becker , E.M. 2003 . Light-induced quality changes in plain yogurt packaged in polylactate and polystyrene . Eur. Food Res. Technol. , 217 : 61 – 69 .
- Jin , T. and Zhang , H. 2008 . Biodegradable Polylactic acid polymer with nisin for use in antimicrobial food packaging . J. Food Sci. , 73 ( 3 ) : M127 – 134 .
- Fang , Q. and Hanna , M.A. 1999 . Rheological properties of amorphous and semicrystalline polylactic acid polymers . Ind. Crops and Products , 10 : 47 – 53 .
- Sinha Ray , S. , Maiti , P. , Okamoto , M. , Yamada , K. and Ueda , K. 2002 . New polylactide/layered silicate nanocomposites. 1. Preparation, characterization and properties . Macromolecule , 35 : 3104 – 3110 .
- Thakur , K.A.M. , Kean , R.T. , Hall , E.S. , Kolstad , J.J. , Lindgren , T.A. , Doscotch , M.A. , Siepmann , J.I. and Munson , E.J. 1996 . Solid State 13C CP-MAS NMR Studies of the Crystallinity and Morphology of Poly(L-lactide) . Macromolecules , 29 : 8844 – 8851 .
- Sinha Ray , S. , Yamada , K. , Okamoto , M. and Ueda , K. 2003 . New polylactide/layered silicate nanocomposites. 2. Concurrent improvements of material properties, biodegradability and melt rheology . Polymer , 44 : 857 – 866 .
- Narayanan , N. , Roychoudhury , P.K. and Srivastava , A.L. 2004 . (+) lactic acid fermentation and its product polymerization Electronic Journal of Biotechnology , 7 ( 2 ) : 15
- Duda , A. and Penczek , S. 1995 . Polymerization of epsilon-Caprolactone initiated by aluminum isopropoxide trimer and/or tetramer . Macromolecules , 28 : 5981
- Ph , Dubois , Ropson , N. , Jrme , R. and Ph , Teyssi . 1996 . Macromolecular Engineering of Polylactones and Polylactides. 19. Kinetics of Ring-Opening Polymerization of ϵ-Caprolactone Initiated with Functional Aluminum Alkoxides . Macromolecules , 29 ( 6 ) : 1965 – 1975 .
- Jamshidi , K. , Hyon , S.-H. and Ikada , Y. 1988 . Thermal characterization of polylactides . Polymer , 29 : 2229 – 2234 .
- Tsuji , H. 2005 . Poly(lactide) stereocomplexes: formation, structure, properties, degradation, and applications . Macromol. Biosci. , 5 : 569 – 597 .
- Hartmann , M.H. 1998 . “ High molecular weight polylactic acid polymers ” . In Biopolymers from Renewable Resources , Edited by: Kaplan , D.H. 367 – 411 . Berlin : Springer-Verlag .
- Wunderlich , B. 2002 . “ Heat capacity of polymers ” . In Handbook of Thermal Analysis and Calorimetry , Edited by: Cheng , S.Z.D. Vol. 3 , 20 – 22 . Amsterdam : Elsevier Science .
- Kline , J. and Kline , H.H. 1959 . Stereochemistry of macromolecular compounds-high-molecular, particularly optical active, polyesters of lactic acid . Makromol Chem , 30 : 23
- Ahmed , J. , Zhang , J. , Song , Z. and Varshnet , S.K. 2009 . Thermal properties of polylactides: effect of molar mass and nature of lactide isomer . Journal of Thermal Analysis and Calorimetry , 95 : 957 – 964 .
- Tsuji , H. and Ikada , Y. 1995 . Properties and morphologies of poly(-lactide): 1 . Annealing condition effects on properties and morphologies of poly(-lactide) Polymer , 36 : 2709 – 2716 .
- Brochu , S. , Prud'homme , R.E. , Barakat , I. and Jérôme , R. 1995 . Stereocomplexation and morphology of polylactides . Macromolecules , 28 : 5230 – 5239 .
- Tsuji , H. , Horii , F. , Nakagawa , M. , Ikada , Y. , Odani , H. and Kitamaru , R. 1992 . Macromolecules , 25 : 4114 – 4118 .
- Kalb , B. and Penings , A.J. 1980 . General crystallization behaviour of poly(L-lactic acid) . Polymer , 21 : 607 – 612 .
- Perego , G , Cella , GD and Bastioli , C. 1996 . Effect of molar mass and crystallinity on poly(lactic acid) mechanical properties . Journal of Applied Polymer Science , 59 : 37 – 43 .
- Palade , L.-I. , Lehermeier , H.J. and Dorgan , J.R. 2001 . Melt Rheology of High L-Content Poly(lactic acid) . Macromolecules , 34 : 1384 – 1390 .
- Dorgan , J.R. and Williams , J.S. 1999 . Melt rheology of poly(lactic acid): Entanglement and chain architecture effects . J. Rheol. , 43 : 1141 – 1155 .
- Sarasua , J.R. , López-Rodríguez , N. , López-Arraiza , A. and Meaurio , E. 2005 . Stereoselective crystallization and specific interactions in polylactides . Macromolecules , 38 : 8362
- Schindler , A. and Harper , D. 1979 . Polylactide. II., Viscosity-molecular weight relationships and unperturbed chain dimensions . Journal of Polymer Science & Polymer Chemistry , 17 : 2593
- Braun , B. , Dorgan , J.R. and Dec , S.F. 2006 . Infrared Spectroscopic Determination of Lactide Concentration in Polylactide . An Improved Methodology Macromolecules , 39 ( 26 ) : 9302 – 9310 .
- Ph , Degée , Ph , Dubois , Jacobsen , S. , Fritz , H.-G. and Jérôme , R. 1999 . Beneficial effect of triphenylphosphine on the bulk polymerization of L,L-lactide promoted by 2-ethylhexanoic acid tin (II) salt Journal of Polymer Science.: Part A . Polymer Chemistry , 37 : 2413 – 2420 .
- Meaurio , E. , Lpez-Rodrguez , N. and Sarasua , J.R. 2006 . Infrared Spectrum of Poly(L-lactide): Application to Crystallinity Studies . Macromolecules , 39 : 9291 – 9301 .
- Kang , S.H. , Hsu , S.L. , Stidham , H.D. , Smith , P.B. , Leugers , M.A. and Yang , X. 2001 . A spectroscopic analysis of poly(lactic acid) structure . Macromolecules , 34 : 4542 – 4548 .
- Urayama , H. , Moon , S.I. and Kimura , Y. 2003 . Macromol. Microstructure and thermal properties of polylactides with different L- and D- unit sequences: importance of the helical nature of the L-sequenced segments . Mater. Eng. , 288 : 137 – 143 .
- Siracusa , V. , Rocculi , P. , Romani , S. and Rosa , M.D. 2008 . Biodegradable polymers for food packaging: a review . Trends in Food Science & Technology , 19 : 634 – 643 .
- Auras , R. , Harte , B. and Selke , S. 2004 . Effect of water on the oxygen barrier properties of poly(ethylene terephthalate) and polylactide films . J. App. Polymer Sci. , 92 : 1790 – 1803 .
- Plackett , D.V. , Holm , V.K. , Johansen , P. , Ndoni , S. , Nielsen , P.V. , Sipilainen-Malm , T. , Södergård , A. and Verstichel , S. 2006 . Characterization of L-Polylactide and L-Polylactide–polycaprolactone Co-polymer films for use in cheese-packaging applications . Packaging Technology & Science , 19 : 1 – 24 .
- Whiteman , N. 2002 . “ Bio-based materials: a reality in the packaging industry ” . In Packexpo , 37 – 42 . Chicago , IL : PMMI .
- Haugaard , V.K. , Weber , C.J. , Danielsen , B. and Bertelsen , G. 2002 . Quality changes in orange juice packaged inmaterials based on polylactate . European Food Research Technology , 214 : 423 – 428 .
- Frederiksen , C.S. , Haugaard , V.K. , Poll , L. and Becker , E.M. 2003 . Light-induced quality changes in plain yogurt packaged in polylactate and polystyrene . European Food Research Technology , 217 : 61 – 69 .
- Ahmed , J. , Varshney , S.K. , Zhang , J.X. and Ramaswamy , H.S. 2009 . Effect of high pressure treatment on thermal properties of polylactides . Journal of Food Engineering , 93 : 308 – 312 .
- Auras , R. , Harte , B. , Selke , S. and Hernandez , R. 2003 . Mechanical, physical and barrier properties of poly(lactide) films . Journal of Plastic Film and Sheeting , 19 ( 2 ) : 123 – 135 .
- Auras , R. , Singh , S.P. and Singh , J.J. 2005 . Evaluation of oriented poly(-lactide) polymers vs. existing PET and oriented PS for fresh food service containers . Packaging Technology and Science , 18 : 207 – 216 .
- Martino , V.P. , Ruseckaite , R.A. and Jiménez , A. Processing and Mechanical characterization of plasticized Poly (lactide acid) films for food packaging Proceeding of the 8th Polymers for Advanced Technologies International Symposium Budapest . Hungary . September . pp. 13 – 16 .
- Bhardwaj , R. and Mohanty , A.K. 2007 . Modification of Brittle Polylactide by Novel Hyperbranched Polymer-Based Nanostructures . Biomacromolecules , 8 : 2476 – 2484 .
- Ljungberg , N. and The , Wesslén, B. 2002 . Effects of Plasticizers on the Dynamic Mechanical and Thermal Properties of Poly(Lactic Acid) . Journal of Applied Polymer Science , 86 : 1227
- Kulinski , Z. , Piorkowska , E. , Gadzinowska , K. and Stasiak , M. 2006 . Plasticization of Poly(L-lactide) with Poly(propylene glycol) . Biomacromolecules , 7 : 2128 – 2135 .
- van Aardt , M. , Duncan , S.E. , Marcy , J.E. , Long , T.E. , O'Keefe , S.F. and Sims , S.R. 2007 . Release of antioxidants from poly(lactide-co-glycolide) films into dry milk products and food simulating liquids . International Journal of Food Science and Technology , 42 : 1327 – 1337 .
- Sharp , J.S. , Forrest , J.A. and Jones , R.A.L. 2001 . Swelling of Poly(DL-lactide) and polylactide-coglycolide in humid environments . Macromolecules , 34 ( 25 ) : 8752 – 8760 .
- Auras , R. 2004 . Investigation of polylactides as a packaging materials, PhD thesis, School of Packaging , 268 East Lansing , MI : Michigan State University .
- Holm , V.K. , Ndoni , S. and Risbo , J. 2006 . The Stability of Poly(lactic acid) Packaging Films as Influenced by Humidity and Temperature . Journal of Food Science , 71 : E40 – 45 .
- Shogren , R. 1997 . Water Vapor Permeability of Biodegradable Polymers . Journal of Environmental Polymer Degradation , 5 ( 2 ) : 91 – 95 .
- Auras , R. , Bruce , H. and Susan , S. 2006 . Sorption of ethyl acetate and d-limonene in poly(lactide) polymers . Journal of the Science of Food & Agriculture , 86 : 648 – 656 .
- Singh , V. M. 2008 . Synthesis of Polylactide with Varying Molecular Weight and Aliphatic Content , 60 – 65 . Philadelphia , PA : Effect on Moisture Sorption, Master's Thesis, Drexel University .
- Colomines , G. , Domenek , S. , Ducruet , V. and Guinault , A. 2008 . Influences of the crystallisation rate on thermal and barrier properties of polylactide acid (PLA) food packaging films . International Journal of Material Form , : 607 – 610 .
- Mutsuga , M. , Kawamura , Y. and Tanamoto , K. 2008 . Migration of lactic acid, lactide and oligomers from polylactide food-contact materials . Food Additives and Contaminants , 25 : 1283 – 1290 .
- Hebert , C.D. , Giles , H.D. , Heath , J.E. , Hogan , D.B. , Modderman , J.P. and Conn , R.E. 1999 . Toxicity of lactide in dog after 2 and 13 weeks of daily oral dosing . Food Chemistry & Toxicology , 37 : 335 – 342 .
- Guilbert , S. , Cuq , B. and Gontard , N. 1997 . Recent innovation in edible and/or biodegradable packaging materials . Food Additives and Contaminants , 14 ( 6 ) : 741 – 751 .
- Petersen , K. , Nielsen , P.V. , Bertelsen , G. , Lawther , M. , Olsen , M.B. and Nilssonk , N.H. 1999 . Potential of biobased materials for food packaging . Trends in Food Science & Technology , 10 : 52 – 68 .
- Alexandre , M. and Dubois , P. 2000 . Polymer-layered silicate nanocomposites preparation, properties and uses of a new class of materials . Material Science and Engineering , 28 : 1 – 11 .
- Sinha Ray , S. and Okamoto , M. 2003 . Polymer/layered silicate nanocomposites: a review from preparation to processing . Progress in Polymer Science , 28 : 1539 – 1641 .
- Lagarn , J.M. , Cabedo , L. , Cava , D. , Feijoo , J.L. , Gavara , R. and Gimenez , E. 2005 . Improving packaged food quality and safety. Part 2: nanocomposites . Food Additives and Contaminants , 22 ( 10 ) : 994 – 998 .
- Sinha Ray , S. and Bousmina , M. 2005 . Biodegradable polymers and their layered silicate nanocomposites: in greening the 21st century materials world . Progress in Material Science , 50 : 962 – 1079 .
- Zeng , Q.H. , Yu , A.B. , (Max) Lu , G.Q. and Paul , D.R. 2005 . Clay-Based Polymer Nanocomposites: Research and Commercial Development . Journal of Nanoscience and Nanotechnology , 5 : 1574 – 1592 .
- Ogawa , M. and Kuroda , K. 1997 . Preparation of Inorganic–Organic Nanocomposites through Intercalation of Organoammonium Ions into Layered Silicates . Bulletin of the Chemical Society of Japan , 70 : 2593 – 2618 .
- Bandyopadhyay , S. , Chen , R. and Giannelis , E.P. 1999 . Biodegradable organic inorganic hybrids based on poly(L-lactic acid) . Polymer Material Science & Engineering , 81 : 159 – 160 .
- Maiti , P. , Yamada , K. , Okamoto , M. , Ueda , K. and Okamoto , K. 2002 . New polylactide/layered silicate nanocomposites: role of organoclay . Chemistry of Materials , 14 : 4654 – 4661 .
- Zhang , J. , Jiang , L. and Zh , L. 2006 . Morphology and Properties of Soy Protein and Polylactide Blends . Biomacromolecules , 7 : 1551 – 1561 .
- Williams , D.F. 1999 . The Williams Dictionary of Biomaterials , 343 Liverpool , , UK : Liverpool University Press .
- Kulkarni , R.K. , Pani , K.C. , Neuman , C. and Leonard , F. 1966 . Polylactic Acid for Surgical Implants Archives of Surgery , 93 : 839 – 843 .
- Edlund , U. and Albertsson , A.C. 2002 . Degradable polymer microspheres for controlled drug delivery . Advances in Polymer Science , 157 : 67
- Jain , AR. 2000 . The manufacturing techniques of various drug loaded biodegradable poly(lactide-co-glycolide) (PLGA) devices . Biomaterials , 23 : 2475 – 2490 .
- Akhtar , S. and Lewis , K. 1997 . Antisense oligonucleotide delivery to cultured macrophages is improved by incorporation into sustained-release biodegradable polymer microspheres . International Journal of Pharmaceutics , 151 : 57
- Miao , Z.M. , Cheng , S.X. , Zhang , X.Z. and Ren-Xi , Zhuo . 2006 . Study on drug release behaviors of Poly-r,â-[N-(2-hydroxyethyl)-L-aspartamide]-g-poly(ϵ-caprolactone) nano- and microparticles . Biomacromolecules , 7 : 2020 – 2026 .
- Peppas , L.B. 1995 . Recent advances on the use of biodegradable microparticles and nanoparticles in controlled drug delivery . International Journal of Pharmaceutics , 116 : 1 – 9 .
- Zilberman , M. and Eberhart , R.C. 2006 . Synthetic Biodegradable Polymers as Medical Devices from The Annual Review of Biomedical Engineering , 8 : 153 – 180 .