Abstract
Raw milk was subjected to conventional isothermal water bath heating, continuous flow microwave heating and continuous flow thermal holding in the pasteurization temperature range (60–75°C), and then immediately cooled in an ice-water bath. The associated alkaline phosphatase (ALP) residual activities were evaluated. Based on the gathered time-temperature profiles, the come-up time (CUT) and come-down time (CDT) contributions to enzyme inactivation were assessed and adjusted prior to first order rate kinetic data handling. The time-corrected D-values of ALP varied from 1250 s at 60°C to 1.7 s at 75°C with a z-value of 5.2°C under conventional batch heating conditions, 128 s at 65°C to 13.5 s at 70°C, with a z-value of 5.2°C under continuous-flow thermal holding condition, 17.6 s at 65°C to 1.7 s at 70°C with a z-value of 4.9°C under continuous-flow microwave heating condition. D values associated ALP inactivation under microwave heating were therefore an order of magnitude lower than under conventional thermal heating. The results thus emphasize that ALP inactivation occurred much faster under microwave heating condition than under conventional heating thereby confirming the existence of enhanced thermal effects from microwave. Because of the enhanced effects, MW pasteurization would reduce the severity of the treatment and hence potentially offer pasteurized milk of higher quality.
INTRODUCTION
Milk serves as an excellent culture and protective medium for certain microorganisms, particularly bacterial pathogens, whose multiplication depends mainly on temperature and competing microorganisms and their metabolic products. The purpose of milk pasteurization is to minimize possible health hazards arising from pathogens and extend temporarily its shelf life with minimal chemical, physical and organoleptic changes. Alkaline phosphatase (ALP) is an indigenous enzyme in raw milk. Because the thermal resistance of ALP is recognized to be slightly greater than that of pathogens commonly present in milk, a negative test for ALP is used as an indicator of adequate pasteurization. Traditional pasteurization of milk is routinely carried out under continuous high-temperature short-time (HTST) heating conditions using heat exchangers (tubular/plate) followed by a brief period of holding (72–75°C for 15–20 seconds), subsequent cooling in heat exchangers (<4°C within 2 h), followed by sealing in plastic or glass packages under sanitary conditions. A drawback for these continuous HTST pasteurization processes is the equipment fouling due to food components exposed to the high temperature inner surface of heat exchangers.
Microwave heating provides a better alternative to solve the fouling problem of heat transfer surface inherent in conventional heat exchanger (plate/tubular). This is mainly due to the internal volumetric heating associated with microwaves keeping the tube surfaces relatively cooler than the product. Continuous-flow microwave heating is a promising technique for milk pasteurization due to the associated fast heating rate, high quality retention, and uniform heating, with energy saving opportunities, prevention of surface fouling and easy access to clean-up. A number of studies have reported successful use of microwave for pasteurization of milk.[Citation1–12] Clare et al.[Citation13] compared the sensory, microbiological and biochemical parameters of microwave versus indirect UHT fluid milk during storage. They concluded that microwave technology may provide a useful alternative processing method for delivery of aseptic milk products that retain a long shelf life. Little attention, however, has so far been given for establishing kinetic data of ALP inactivation for continuous-flow microwave heating, although there has been reference to its use in conventional thermal processing applications.[Citation14]
In order to establish a processing schedule for milk pasteurization based on ALP inactivation, kinetic data of ALP under the appropriate conditions are required. The objectives of this study were, therefore, (1) to evaluate the inactivation kinetic parameters (D and z-values) of ALP in milk during conventional batch heating in the pasteurization temperature range (60–75°C) (base data); (2) to evaluate the inactivation kinetic parameters of ALP during continuous-flow microwave heating and continuous flow thermal holding in the temperature range of 65–70°C; and (3) to compare the inactivation kinetic parameters of ALP during continuous-flow microwave heating with conventional heating.
METHODOLOGY
Preparation of Milk Samples
Raw, fresh milk was obtained from a local farm late in the afternoon on the day preceding experimentation and kept at 4°C until use. 100 ml of raw milk was heated in water bath at 100°C for 20 min then immediately cooled in ice-water bath and stored at 4°C. The boiled milk was used as control blank (no residual ALP activity).
Conventional Water Bath Thermal Treatment
Two-ml aliquots of raw milk (20°C) in glass tubes (13-mm O.D., 100-mm length) were subjected to heat treatments in a well-agitated water bath (Model: 1267-62, Cole-Parmer Instrument Company) at selected temperatures for different time intervals, then cooled immediately in an ice-water bath. Temperatures employed for heat treatment were 60, 65, 70 and 75°C, respectively. All treatments were duplicated. ALP residual activities were determined as detailed later. The come-up time (CUT) and come-down time (CDT) temperature profiles were gathered during the heat treatment using T-type thin-wire (0.381 mm diameter) copper-constantan thermocouples (Omega Engineering Inc., Stamford, CT) attached to a data-logger (Hewlett Packard 34970A, 20-channel multiplexer).
Effectiveness of heating time and kinetic data analyses
The CUT and CDT were taken into account for correcting the kinetic parameters (D and z-values). Briefly, the heating times (including the come-up period) were first considered without any correction, and based on a semi-logarithmic relationship of residual activity of ALP with time (first order kinetics), the decimal reduction time (D-value, or time to reduce 90% of ALP activity) was computed for each temperature (as the negative reciprocal regression slopes of the linear portion of the semi-logarithmic curve). The D-values on logarithmic scale were then plotted against the temperature to obtain the temperature sensitivity indicator, z (again as the negative reciprocal regression slope of the log D versus temperature curve). Using this computed z value and the gathered time-temperature profile during both CUT and CDT, the effective heating time (te) during both CUT and CDT were obtained using the process lethality EquationEq. (1)[Citation15–17]:
Continuous-flow Microwave and Thermal Holding Treatments
Two domestic microwave ovens with a nominal output of 1000 W at 2450 MHz (Sunbeam, Model: SMW 1150, Curtis International Ltd. 315 Attwell Drive, Toronto, Ontario, M9W 5C1) were modified to set up continuous-flow microwave heating system (). The microwave ovens had cavity dimensions: 38.4-cm long, 34.9-cm wide, and 21.9-cm high. The two ovens were connected by an insulated short Norprene® tubing (7.9 mm ID, Cole-Parmer Instrument Company). Two helical coils (coil diameter: 105 mm, tube ID: 7.9 mm; turns; coil height: 110 mm, coil volume 100 ml) made of Pyrex® glass tubing were centrally located in the two microwave oven cavities. The test fluid in an insulated stainless steel container (25 liters) was circulated through coils for microwave heating using a calibrated variable-speed metering pump (Masterflex®, Model No: 7524-40, Cole-Parmer Instrument Company). Inlet (T1), middle (T2) and outlet temperatures (T3), as well as the temperature after cooling (T4) were continuously gathered using specially prepared T-type thin-wire thermocouples (Omega Engineering Inc., Stamford, CT) centrally inserted into the tubing just outside the microwave ovens with its surface parallel to the direction of the fluid flow and attached to the data-logger (Hewlett Packard 34970A, 20 channels multiplexer). Each thermocouple was welded on a copper fin and calibrated against a mercury-in-glass thermometer (ASTM – American Standard for Testing Materials – certified) before installation. The accuracy of the temperature measured by the modified thermocouple was ± 0.1°C.
Figure 1 A schematic diagram of continuous-flow microwave heating and isothermal holding system set-up. (Figure provided in color online.)
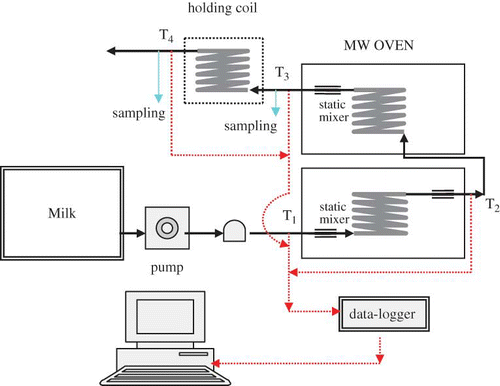
Flow rates were measured at the outlet. The average residence time of the test liquid in each coil was determined by dividing the coil volume by the steady state volumetric flow rate of the liquid running through the system. In order to maintain a stable flow rate, one plastic buffer (Cole-Parmer Instrument Company) was installed between metering pump and the first oven, and the direction of the fluid flow in the MW system was kept upward in order to have a better control of flow. Static mixers (8-mm diameter, 50-mm length, Teflon, Omega Engineering Inc.) were installed at the inlet and outlet ports of each glass coil to ensure the better mixing condition and reduce temperature gradient across the radius of the tube. In the thermal holding set-up, an insulated short Norprene® tubing (7.9 mm id) was used to introduce the microwave heated fluid exiting from the second oven to a well insulated helical coil (coil diameter: 106 mm, tube inner diameter: 7.9 mm; turns; coil height: 110 mm, coil volume 100 ml) made from Pyrex® glass tubing. One T-type thin-wire (0.381) thermocouple (modified as detailed earlier) was installed immediately outside the holding coil and attached to the data-logger.
Before each run, raw milk was preheated to required initial temperatures (10–30°C) in a water bath (≤50°C) for 10–20 min then immediately transferred to the well insulated stainless steel container (storage tank) for microwave treatment. The conventional D-value of ALP at 60°C is about 25 min with a z-value of 5–7°C, and this would bring the D-value at 50°C to be over 1500 min, thus the preheating treatment would not affect ALP activity in raw milk. The preheated milk was run through the system long enough to purge out water used for stabilizing the system or microwave heated milk previously present in the system and to establish the steady state condition indicated by constant temperatures at the four thermocouple locations. The initial temperature and flow rate were pre-adjusted (based on preliminary runs) to obtain several heating times at each exit temperature. During microwave heating, temperatures at four thermocouple locations were recorded at every 1 second intervals. After establishing steady state conditions, test sample was withdrawn first from the exit of the holding coil (downstream) followed by the exit after the second oven at temperatures between 65 and 70°C. The treated samples (<10 mL) were collected into a pre-cooled glass conical flask and cooled immediately in ice-water bath. All treatments were duplicated. Subsequently, ALP residual activities were determined as detailed later. After completing an experiment, the stainless steel storage container was washed and then water was run through the system at high flow rate for 20 min to purge out residual milk in the system, and then microwave ovens were turned on, the system was sanitized by circulating hot water in a closed system for 30 min at low flow rates to maintain all exit temperature at >70°C.
Effectiveness of microwave heating time and kinetic data analyses
Continuous-flow microwave heating used in the study is a fully non-isothermal heating treatment consisting of a come-up period inside the oven during microwave heating and a come-down period outside during cooling. There is no MW hold period. The holding tube subsequent to MW ovens, when used, will only provide continuous flow thermal hold. The temperature of test samples increased from an initial temperature at the entry port of MW Oven #1 to a final temperature at the exit port of MW Oven #2. It is necessary to determine temperature profile inside each oven to estimate effective microwave heating time. Tajchakavit and Ramaswamy[Citation18] used a Luxtron fiber optic probe to measure temperatures of test samples inside microwave oven at three locations (0.25, 0.5, and 0.75 tube length from the entrance) along the coil length for continuous-flow microwave heating system. They reported that temperature rise in microwave oven was slightly non-linear (convex) with the temperature registered generally higher than in a linearly increasing profile. The temperatures along the coil length inside each microwave oven were modeled as follows (based on the inlet and outlet temperatures):
Again briefly, as described earlier with conventional heating, the D-values at the exit temperature were first calculated from the regression of log residual ALP activity versus uncorrected heating time (i.e., using the average residence time), and then the z-value was obtained as negative reciprocal slope of log D versus temperature. Using the calculated z value, the heating times were then corrected using EquationEq. (1), and corrected D-values, and subsequently the corrected z, were calculated. As before, this step was repeated until 0.5% convergence of z-value was obtained.[Citation18]
Because there were two microwave ovens, the effective heating time, te = te1 + te2, where te1, te2 were the effective heating times for MW Oven 1, MW Oven 2, respectively. It should be recognized that the mechanism by which microwave heats foods is different from the conventional, and that this could have an entirely different influence on ALP inactivation. In the microwave heating system, heating is done inside the microwave oven and cooling outside. The calculation of effective portion of come-down time (CDT) for microwave heating was similar to that for conventional heating. The CDT contribution to the ALP inactivation was relatively very small as compared with total effective time (detailed later) since the sample temperature was almost instantaneously dropped to below lethal levels in the pre-cooled conical flask surrounded by crushed ice, thus the contribution of CDT for ALP inactivation could almost be ignored.
Estimation of ALP activity and data analysis
After heat treatment, the residual ALP activity in milk was measured essentially according to AOAC method[Citation19] quantitative spectrophotometric procedures. Phenol calibration curves were run with each batch of unknowns.
RESULTS AND DISCUSSION
Inactivation Kinetics During Conventional Batch Heating
Although test samples in the well agitated water bath were only 2 ml, a certain time lag was inevitable before the sample reached the target temperatures. Likewise a small delay was accompanied during cooling. Ignoring CUT and CDT contributions to lethality could result in errors in kinetic parameters determination especially at high temperatures where a short time can induce appreciable inactivation.
shows a typical come-up period time-temperature profile for milk subject to conventional isothermal water bath heating at 70°C. The lethal rate curve was constructed by plotting the lethal rate for each data point against associated heating time. The effective time was calculated by integration of lethal rate curve. As illustrated in , the effective portion of come-up period was larger than that of come-down period, where heating at 70°C for 110 s yielded an effective time of 32.2 s. A coupled come-down temperature profile is also illustrated in for the same condition. The temperatures decreased rapidly and reached levels where thermal inactivation was negligible within about 5–6 s. The effectiveness of CDT was 0.3 s, therefore much smaller as compared with 32.2 s (effectiveness of CUT), nevertheless, it was included in the computation of kinetic data under conventional batch heating condition.
Figure 2 Time-temperature profile (dotted line) and corresponding lethal rate profile (solid line) for milk under isothermal water bath heating condition.
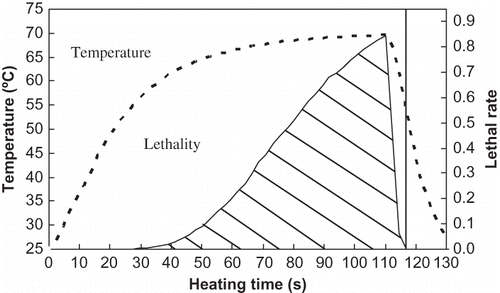
shows typical survival curves of ALP both before and after making come-up and come-down period corrections. As expected, the inactivation behavior indicated characteristic first-order rate kinetics. The inactivation rates increased with increasing temperatures. D-values ranged from 1250 s at 60°C to 8.9 s at 75°C before heating time corrections, 1250 s at 60°C to 1.7 s at 75°C after heating time correction. The difference between uncorrected and corrected D-values increased with an increasing temperature. As compared with lower temperature (60 and 65°C), treatment at higher temperatures (70 and 75°C) required longer come-up periods and shorter heating time, hence, the lag periods became more apparent and significant.
Temperature Sensitivity of D Values
shows the uncorrected and corrected z-values of ALP in milk during the conventional batch heating were 6.88 and 5.18°C, respectively. The results are summarized in . There are some literature data on thermal inactivation kinetics of ALP. Levieux et al.[Citation20] reported a D value of 23 min at 60°C with a z value of 6.8°C while Claeys et al.[Citation21] reported D at 60°C value of 24.6 min with a z value of 5.3°C. These are within a reasonable range from the data obtained in the present study (D60 20 min and a z value of 5.2°C) although using different experimental procedures for heating of milk. The commercial pasteurization procedures include heating of milk at 63°C for 30 min, or at 72°C for 16 s. These conditions would result in about 2–3 D in ALP activity (99–99.9% inactivation). It also shows that at lower temperatures the D-value increases quickly reaching over 1500 min at temperatures below 50°C.
Figure 4 Temperature sensitivity curves for ALP in milk during conventional batch heating treatment showing uncorrected (dotted line) and corrected (solid line) heating times. (Figure provided in color online.)
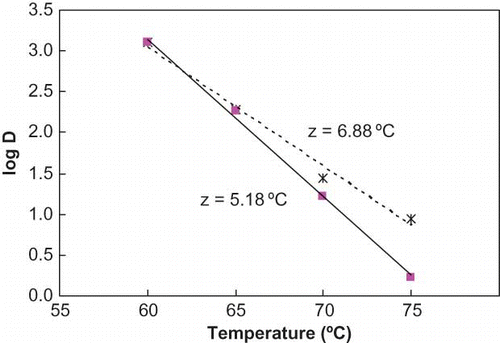
Table 1 Thermal kinetic parameters (D- and z-values) of ALP in milk at various temperatures under conventional isothermal water bath heating condition
Inactivation Kinetics during Continuous Flow Microwave Heating
Raw milk was subjected to continuous-flow microwave heating with initial temperatures and flow rates appropriately adjusted to obtain desired exit temperatures. shows, at initial temperature 20°C (T1) and flow rate 480 ml/min, the outlet temperatures of MW Oven 1 (T2), Oven 2 (T3), and holding coil (T4) stabilized after 20, 60, and 100 s, respectively from the time microwave ovens were turned on. The non-linearity in time-temperature data during early phase of heating (lag period) can easily be explained by the heat sink contributed by the coil and environment within the cavity as explained by Kudra et al.[Citation7] Samples were generally withdrawn only after the stability was achieved. A typical time-temperature profile of test samples inside microwave ovens and their corresponding effective times during continuous-flow microwave heating are illustrated in . As previously described for kinetic data analyses, the effective microwave heating time, te, at the exit temperature were computed based on EquationEq. (1) by taking the effectiveness of CUT into account. The temperature profiles of 3 locations (0.25, 0.5 and 0.75 coil length from entrance) inside each microwave oven were determined by Equationeqn (2), Equation(3), Equation(4) based on temperature profiles gathered from thermocouples outside microwave ovens. A typical cumulative effective heating time curve is also illustrated in giving a come-up period effectiveness of 2.34 s/34.14 s for the CUT period. The D-values and z-values were corrected for microwave heating time as detailed in the methodology and the results are summarized in .
Figure 5 Time-temperature profiles for milk at inlet of oven 1 (T1), outlet of oven 1 (T2), outlet of oven 2 (T3) and outlet of the holding coil (T4) in continuous-flow microwave heating system at initial temperature 20°C and flow rate 480 ml/min. (Figure provided in color online.)
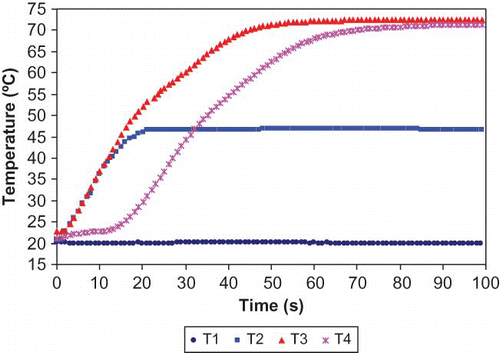
Figure 6 Time-temperature profile of milk during continuous-flow microwave heating (dotted line) and computed effective times (solid line) at exit temperature °C. (Figure provided in color online.)
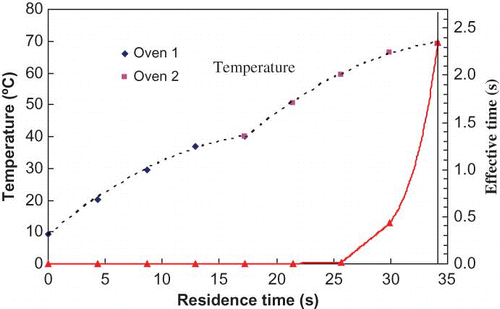
Table 2 Thermal kinetic parameters (D- and z-values) of ALP in milk at various temperatures under continuous-flow microwave heating
illustrates microwave inactivation kinetics of ALP in milk after making the corrections to the heating (residence) times. The corrected D-values ranged from 17.6 s at 65°C to 1.7 s at 70°C with corresponding z-value of 4.9°C. Results again indicated characteristics first-order reaction kinetics during microwave heating. The rate of inactivation generally increased with an increase in temperature.
Inactivation Kinetics during Continuous Flow Thermal Holding
As illustrated in , milk was passed through a glass holding coil after microwave treatment. There was only a small drop in temperature (≤1°C) between the inlet port and the outlet port of holding coil. Since thermal holding is a conventional heating process, the time-temperature profile could be linearly constructed according to inlet and out temperatures, as well as the average residence time. Based on the time-temperature profile, z-value of 5.18°C from conventional batch heating was used for the first calculation of effective heating times at various exit temperatures as per EquationEq. (1).
The D-values and z-values were corrected as detailed in the methodology and the results are summarized in . shows the time corrected survival curves of ALP during isothermal holding. The corrected D-values ranged from 128.2 s at 65°C to 13.5 s at 70°C with corresponding z-value of 5.15°C. Results indicated inactivation kinetics of ALP during continuous flow thermal holding followed conventional first order kinetics.
Figure 8 Survival curves of ALP in milk during continuous-flow thermal holding as a function of corrected heating times. (Figure provided in color online.)
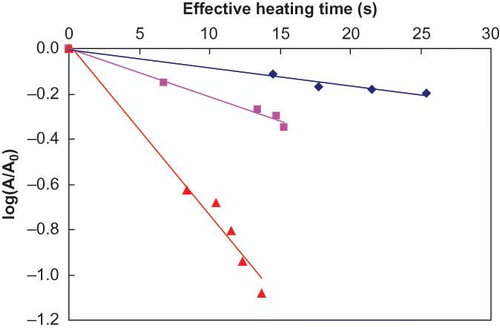
Table 3 Thermal kinetic parameters (D- and z-values) of ALP in milk at various temperatures during continuous-flow isothermal holding (holding coil volume = 100 ml)
Kinetic Comparison: Thermal Batch, Thermal Continuous Flow, and Microwave Continuous Flow
The D-values of ALP varied from 1250 s at 60°C to 1.7 s at 75°C with z-value of 5.2°C for conventional bath heating, 128 s at 65°C to 13.5 s at 70°C with a z-value of 5.2°C for continuous-flow thermal holding, 17.6 s at 67°C to 1.7s at 70°C with z-value of 4.9°C for continuous-flow microwave heating. The results are summarized in . shows the comparison of temperature sensitivity of ALP in milk between the different heating modes. The results indicate that the thermal resistance of ALP under conventional batch heating conditions were similar to those reported in literature. The continuous flow heating conditions provided a sharper D values (about 25 lower) relative to batch systems again as has been observed in earlier studies.[Citation10,Citation18] The continuous flow MW heating provided the most effective condition for ALP inactivation with D values nearly a further order of magnitude lower than under batch heating conditions. Relative to MW heating, the D values under the conventional heating conditions were nearly 7–10 times higher.
Figure 9 Temperature sensitivity curves for inactivation rates of ALP under different conditions. (Figure provided in color online.)
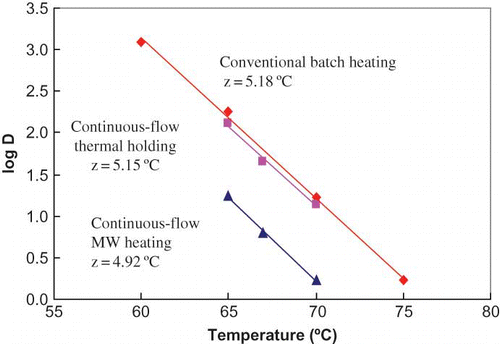
Table 4 Thermal kinetic parameters (D- and z-values) of ALP in milk under conventional isothermal water bath heating, continuous-flow MW heating and continuous-flow thermal holding conditions
D-values associated ALP inactivation under continuous-flow thermal holding was 25% lower than under conventional batch heating, but the nature of ALP inactivation between continuous flow thermal holding and conventional batch heating was similar (with similar z values). Tajchakavit and Ramaswamy[Citation18] reported that continuous-flow thermal holding had higher inactivation rate (lower D-value) for PME in orange juice as compared with the conventional bath heating and suggested (1) there may be some residual effect from the microwave heat treatment causing the inactivation in the isothermal holding tubes to be better than in conventional heating, or (2) it may be due to the inherent variations in the continuous set-up with respect to radial temperature profiles and residence distribution.
No significant ALP inactivation was observed at lower temperatures (≤60°C with short residence times of under 25 s) during continuous flow microwave heating; hence there was no evidence for the existence of non-thermal MW effect in this study. However, as the temperature is elevated to 65–70°C, ALP inactivation under continuous-flow microwave heating occurred about 10 times faster than conventional heating showing there were additional effects associated with microwave heating which were temperature dependent. The effect was described as “enhanced thermal effects of microwave heating” than the traditionally used “non-thermal effects,” which did not exist.[Citation18] This effect has already been demonstrated in several earlier studies for other enzymes and microorganisms,[Citation10] and hence goes to further confirm the trends.
CONCLUSIONS
The application of isothermal batch heating, continuous flow microwave heating and thermal holding for the inactivation of ALP in milk were explored and compared in pasteurization temperature range (60–75°C). Based on gathered time-temperature profiles, CUT and CDT were suitably corrected for obtaining kinetic parameters. Inactivation kinetics of ALP during above heating conditions followed typical first-order kinetics. Within the range of temperatures and samples sizes employed in this study, continuous-flow thermal holding was a little more efficient than conventional batch heating for ALP inactivation. The continuous flow microwave heating inactivated ALP in milk by an order of magnitude faster than conventional thermal heating. Results confirmed the existence of enhanced thermal effects from microwave.
REFERENCES
- Jaynes , H.O. 1975 . Microwave pasteurization of milk . J. Milk Food Technology , 38 : 386 – 397 .
- Chiu , C.P. , Tateishi , K. , Kosikowski , F.V. and Armbruster , G. 1984 . Microwave treatment of pasteurized milk . J. Microwave Power , 19 : 269 – 272 .
- Decareau , R.V. 1984 . Microwaves in food processing . Food Technology, Australia , 36 ( 2 ) : 81 – 86 .
- Decareau , R.V. 1985 . Microwaves in the Food Processing Industry , 234 New York : Academic Press, Inc .
- Knutson , K.M. , Marth , E.H. and Wagner , M.K. 1988 . Use of microwave ovens to pasteurize milk . J. Food Protection , 51 ( 9 ) : 715 – 719 .
- Thompson , J.S. and Thompson , A. 1990 . In-home pasteurization of raw goat's milk by microwave treatment . International Journal of Food Microbiology , 10 : 59 – 64 .
- Kudra , T. , van de Voort , F.R. , Raghavan , G.S.V. and Ramaswamy , H.S. 1991 . Heating characteristics of milk constituents in a microwave pasteurization system . J. Food Science , 56 ( 4 ) : 931 – 934 .
- Villamiel , M. , López-Fandiño , R. , Corzo , N. , Martínez-Castro , I. and Olano , A. 1996 . Effects of continuous flow microwave treatment on chemical and microbiological characteristics of milk . European Food Research and Technology , 202 ( 1 ) : 15 – 18 .
- Anantheswaran , R.C. and Ramaswamy , H.S. 2001 . “ Chemical and Biological Changes Due to Heating. Microbial and biochemical destruction during microwave heating ” . In In Handbook of Microwave Technology For the Food Applications , Edited by: Datta , A.K. and Anantheswaran , R.C. 191 – 213 . New York , NY : Marcell Dekker Inc . Chapter 6
- Ramaswamy , H.S. , Koutchma , T. and Tajchakavit , S. 2002 . “ Enhanced thermal effects under microwave heating conditions ” . In Engineering and Food , Edited by: Welti-Chains , J. , Barbosa-Canóvas , G. and Aguilera , J.M. New York , NY : Technomic Publishing Co . 2002
- Gentry , T.S. and Roberts , J.S. 2005 . Design and evaluation of a continuous flow microwave pasteurization system for apple cider . LWT , 38 : 227 – 238 .
- Tessier , F.J. , Gadonna-Widehem , P. and Laguerre , J.C. 2006 . The fluorometric FAST method, a simple tool for the optimization of microwave pasteurization of milk . Molecular Nutrition & Food Research , 50 ( 9 ) : 793 – 798 .
- Clare , D.A. , Bang , W.S. , Cartwright , G. , Drake , M.A. , Coronel , P. and Simunovic , J. 2005 . Comparison of Sensory, Microbiological, and Biochemical Parameters of Microwave Versus Indirect UHT Fluid Skim Milk During Storage . J. Dairy Science , 88 : 4172 – 4182 .
- Lu , Q. , Piyasena , P. and Mittal , G.S. 2001 . Modeling Alkaline Phosphatase Inactivation in Bovine Milk during High-Temperature Short-Time Pasteurization . Food Science and Technology International , 7 : 479
- Ramaswamy , H.S. and Ranganna , S. 1981 . Thermal inactivation of peroxidase in relation to quality of frozen cauliflower (var. Indian Snowball) . Can. Inst. Food Science. Technology Journal , 14 ( 2 ) : 139 – 143 .
- Awuah , G. , Ramaswamy , H.S. and Simpson , B.K. 1993 . Thermal inactivation kinetics of trypsin inhibitor at aseptic processing temperature . Journal of Food Process Engineering , 16 : 315 – 328 .
- Tajchakavit , S. 1997 . Continuous-flow microwave heating of orange juice: Kinetics of enzyme inactivation/microbial destruction and evaluation of enhanced thermal effects , PhD Thesis, McGill University, Food Science Department .
- Tajchakavit , S. and Ramaswamy , H.S. 1997 . Continuous-flow microwave inactivation kinetics of pectin methyl esterase in orange juice . J. Food Processing and Preservation , 21 : 365 – 378 .
- AOAC . 1995 . Official Method of Analysis of Phosphatase (Residual) in milk , 16th , V.2 Chapter Vol. 33 , 35 – 36 . (AOAC Official Method 979.13); AOAC .
- Levieux , D. , Geneix , N. and Levieux , A. 2007 . Inactivation-denaturation kinetics of bovine milk alkaline phosphatase during mild heating as determined by using a monoclonal antibody-based immunoassay . J. Dairy Research , 74 ( 3 ) : 296 – 301 .
- Claeys , W.L. , Ludikhuyze , L.R. , van Loey , A.M. and Hendrickx , M.E. 2001 . Inactivation kinetics of alkaline phosphatase and lactoperoxidase, and denaturation kinetics of beta-lactoglobulin in raw milk under isothermal and dynamic temperature conditions . J. Dairy Research , 68 ( 1 ) : 95 – 107 .