Abstract
An improved thermal conductivity probe system consisting of a probe heater, control circuits and data test system was developed and described. It has the merits of the short duration of the experiments, simplicity, high accuracy, and relatively small sample requirement. The measurement time is about 20 s. The effective volume of sample is 50 ml. Calibration of the probe with glycerin and ethanol in this work exhibits an accuracy of 1.4% and 1.9%, respectively. The probe system was used to measure the thermal conductivity of orange juice. The measured thermal conductivity values were linearly dependent on the concentration and temperature of the orange juice. A decrease in the concentration of orange juice and an increase in the temperature resulted in an increase in the values of thermal conductivity. However, the influence of concentration on the thermal conductivity of orange juice was stronger than that of temperature. An empirical model for the thermal conductivity of orange juice as a function of concentration and temperature was obtained.
NOMENCLATURE
C | = |
Instrument constant of the probe, Ω/m K s |
E | = |
Electric voltage of power supply, V |
K | = |
Thermal conductivity, W/m K |
L | = |
Effective length of the probe, m |
Q | = |
Heat input per unit length, W/m |
R | = |
Electric resistance values, Ω |
R0 | = |
Electric resistance value of the copper electric resistance at 0°C, Ω |
s | = |
Concentration of the orange juice (°Brix) |
T | = |
Temperature of the copper wire, K |
t | = |
Temperature of the orange juice, °C |
U | = |
Electric voltage, V |
△R | = |
Electric resistance rise of copper wire in probe, Ω |
△V | = |
Output electric voltage of the electric circuit, V |
Greek Symbols | = |
|
α0 | = |
Copper electric resistance's temperature coefficient at 0°C, 1/K |
θ(τ i ) | = |
Initial temperature, K |
θ(τ) | = |
Temperature difference, K |
θ | = |
Temperature of the copper wire of probe, K |
| = |
Average temperature of the copper wire of probe, K |
τ | = |
Heating time, s |
τ i | = |
Initial time, s |
Subindexes | = |
|
s | = |
Resistance box |
p | = |
Thermal probe |
INTRODUCTION
Thermal conductivity of fruit juice is one of the important thermal properties used to estimate the rate of heat transfer during processes, such as preservation, transportation and freezing. Orange juice is one of the most important commodities in the Chinese market; it contains sugar, carotenoid, citric acid, malic acid, pectin, and a complex mixture of vitamins. Most especially, orange juice contains a large amount of vitamin C. Measurement of the thermal conductivity of every product under every conceivable condition would be an enormous task, so the use of models to predict thermal conductivity using other more easily measured properties, such as concentration and temperature, appears to be the best way to assure the availability of data. Mathematical models that express the dependence of thermal conductivity on temperature and concentration are a very appealing alternative to experimentation, and they are a useful tool for the implementation of computer-aided procedures for equipment design and process automation.
Many thermal conductivity values for food are available in the literature.[Citation1–5] Generally, an extensive review of existing methods of measuring thermal conductivity of food has been carried out.[Citation6–11] Some investigators reported the experimental values of thermal conductivity of orange juice as well as the mathematical models for their calculations.[Citation12,Citation13]
Several techniques including steady and non steady state methods have been used to evaluate the conductivity of food systems.[Citation5] The line heat source thermal conductivity probe is recommended for most food applications because it is simple, fast, convenience, low cost and suitable for small sample size.[Citation14] In this work, an improved thermal conductivity probe system was used to determine the thermal conductivity of orange juice. Advantages of this system are the short duration of the experiments, simplicity, high accuracy, and relatively small sample requirement. The measurement time is about 20 s. The effective volume of sample is 50 ml. Calibration of the probe with glycerin and ethanol in this work exhibits an accuracy of 1.4% and 1.9%, respectively. The specific objectives of this research were (1) to elaborate a specifically designed probe system for thermal conductivity of orange juice; and (2) to develop prediction equations for thermal conductivity of orange juice as functions of concentration and temperature.
MATERIALS AND METHODS
Sample Preparation
Orange juice (66°Brix) was supplied by SanMenXia Lake Juice Company, Henan Province, China. All the orange juice samples were mixed with the ultra pure water with an electric resistance rate of 18.2 MΩ cm to obtain various concentrations of 5°Brix, 10°Brix, 20°Brix, 30°Brix, 40°Brix, 50°Brix, and 66°Brix. The concentrations of orange juice were determined using an Abbe Refractometer (2WAJ, Shanghai Precision & Scientific Instrument Company, China) with ±0.1°Brix precision at 20°C. The prepared samples were put into several identical cylindrical glass holders (effective volume 50 ml) with a diameter of 50 mm and height of 60 mm. The samples were equilibrated to the desired temperature by a constant temperature cabinet with ±0.5°C precision.
Thermal Conductivity
In this experiment, a specifically designed thermal conductivity probe system was used to measure thermal conductivities of juice samples by an idealized transient heat conduction model.[Citation11,Citation15,Citation16] In the system, a probe was made of a stainless steel needle; its length was 32 mm, outside diameter of 0.7 mm and inside diameter 0.3 mm. Paint-wrapping copper wire was used to coat the inside of the needle. The probe was full of vacuum silica gel, which had good heat conduction and electricity insulation. Both of the probe ends were sealed with epoxide resin glue ().
During the measurement of thermal conductivity, the probe was inserted into samples that filled the sample holder. The samples were equilibrated to the desired temperature by a constant temperature cabinet. Then the thermal conductivity of the sample (k) was calculated from the slope of average temperature () against logarithmic time (lnt) and power input (q) according to EquationEq. (1) given by Carslaw and Jaeger[Citation17]:
Before the probe was heated, the resistance box's value (RS ) was adjusted to be equal to the electric resistance value of the probe (Rp ). Thus the electric voltage of the probe (Up ) and the resistance box's (Us ) were also equal according to the principle of Wheatstone bridge (EquationEq. (2)). In the course of heating the copper wire of the probe was heated, and the electric resistance had an increment, which was expressed with △R, and then the probe's electric resistance (Rp ) could be expressed in EquationEq. (3).
If ΔV << E, Eqs. (3) and (5) could become EquationEqs. (6) and Equation(7), respectively.
EquationEquations (4) and Equation(6) were differentiated and integrated:
Then the thermal conductivity of the sample could be obtained as EquationEq. (9) after turning EquationEqs. (7) and Equation(8) into (1).
Or
EquationEquation (10) was the basis of the thermal conductivity measurement. In EquationEq. (10), C = α 0R0 /64πL was named instrument constant of the probe, Ω/m K s; which only related with the probe material, length and had nothing with the heating power, testing temperature of the testing system, △V was the output electric voltage of the electric circuit, V; τ was heating time(s). k was the thermal conductivity of sample, W/m K. E was the electric voltage of power supply, V. Rs was the initial electric resistance value of probe, Ω.
Firstly, the probe should be calibrated. The probe was inserted directly into ultra pure water with a cyclindrical glass holder, whose electric resistance rate was 18.2 MΩ cm and thermal conductivity was known in the literature.[Citation19] Secondly, the electric voltage signals tested by the data measurement system and heating time were fed into a computer. Thirdly, the curve of ΔV-ln τ could be drawn. Finally, according to the least-squares method, the slope of d(ΔV)/d(ln τ) could be obtained. And this should be repeated eight times to obtain the slope average. Then the instrument constant of the probe could be obtained by EquationEq. (10). Using this method the thermal conductivities of glycerin, ethanol and orange juice with different concentrations of 5°Brix, 10°Brix, 20°Brix, 30°Brix, 40°Brix, 50°Brix, 60°Brix and 66°Brix under the environment temperature of 0°C, 10°C, 20°C, 30°C, 40°C, 50°C, 60°C, and 70°C could be measured, respectively. According to the obtained instrument constant of the probe, thermal conductivities of 48 samples could be calculated.
RESULTS AND DISCUSSION
Calibration of Instrument Constant Value
The ultra pure water was used to calibrate the probe. The test data for ultra pure water at 20°C is shown in . During the test run, the heating time was no more than 20 seconds and the temperature rise was no more than 1°C, which could weaken convection effects. The linear correlation degree of d(ΔV)/d(ln τ) curve was more than 0.9999 and the relative deviation was less than 2%. The reproducibility was better than 2%. From , the average value of d(ΔV)/d(ln τ) can be calculated. According to EquationEquation (10), the demarcation value of the instrument constant of the probe can be obtained, and it was C = 0.0355 Ω/m K s.
Table 1 Measured Data of ultra pure water at 20°C
Thermal Conductivities of Glycerin and Ethanol
In order to check the accuracy of this method, the thermal conductivities of glycerin (purification degree of more than 99%), ethanol (purification degree of more than 99.7%), were measured in a constant temperature cabinet in the laboratory at 15°C ± 0.5°C. Each sample was measured 6 times under the same conditions and the average was calculated. The experimental results are listed in . The relative errors with reference values[Citation19] were within 2% and every sample's maximal deviation to the mean tested thermal conductivity to be within 2%.
Table 2 Thermal conductivity values of some liquids
Influence of Concentration on the Thermal Conductivity of Orange Juice
The thermal conductivities of orange juice with 5°Brix, 10°Brix, 20°Brix, 30°Brix, 40°Brix, 50°Brix, 60°Brix and 66°Brix were determined at 0°C, 10°C, 20°C, 30°C, 40°C, 50°C, 60°C, and 70°C, respectively. shows that there was a high negative correlation between concentration and thermal conductivity at the same temperature. Using Excel and linear regression analysis, the regression equations ① between concentration (s) and thermal conductivity (k) were established. The six equations had high significance according to the Fisher test (α = 0.01). Therefore, it was suitable to represent the relation between thermal conductivity and concentration at the same temperature. The regression equations are shown in .
Table 3 Regression equations and variance analysis describing relations between concentration, temperature, and thermal conductivity
Influence of Temperature on the Thermal Conductivity of Orange Juice
The thermal conductivities of orange juice with 5°Brix, 10°Brix, 20°Brix, 30°Brix, 40°Brix, 50°Brix, 60°Brix, and 66°Brix were determined at 0°C, 10°C, 20°C, 30°C, 40°C, 50°C, 60°C, and 70°C, respectively. shows that there was a high positive linear correlation between temperature and thermal conductivity of orange juice at the same concentration. Using Excel and linear regression analysis, the regression equations ② between temperature (t) and thermal conductivity (k) were established. They had high significance according to the Fisher test (α = 0.01). Therefore, it was suitable to predict the thermal conductivity of orange juice at the same concentration at different temperature conditions. The regression equations are shown in .
Prediction Equation of Thermal Conductivities of Orange Juice with Temperature and Concentration
Data Processing System software was used to process the experimental data. The double-factors regressive equation of thermal conductivities of orange juice with temperature and concentration could be obtained:
Figure 6 Comparison of thermal conductivity calculated from EquationEq. (11) and in literature at 10°C.
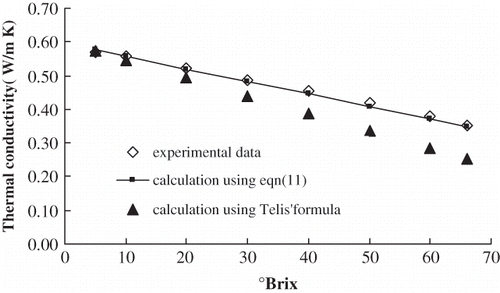
Figure 7 Comparison of thermal conductivity calculated from EquationEq. (11) and in literature at 10°Brix.
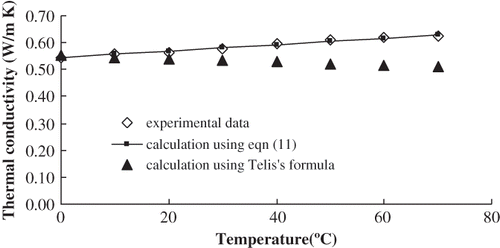
CONCLUSIONS
The effects of concentration in the range of 5–66°Brix and temperature in the range of 0–70°C on the thermal conductivity of orange juice were determined in this study. The thermal conductivity probe system was proposed, which needs short duration of the experiments, simplicity, high accuracy, and relatively small sample requirement. The system worked satisfactorily and its application could be extended to measure thermal conductivities of other solid and liquid foods. The thermal conductivity of orange juice decreased with an increase in concentration, while it increased with an increase in temperature. In addition, the influence of concentration on the thermal conductivity of orange juice was stronger than that of temperature. An empirical correlation for the thermal conductivity of orange juice as a function of concentration and temperature was obtained. The results of this work have direct application as predictive equations for fruit juice processes of heat transfer.
ACKNOWLEDGMENTS
This work is supported by the Natural Science Foundation of China, Grant No 30771245.
REFERENCES
- Sweat , V.E. and Haugh , C.G. 1974 . Thermal conductivity probe for small food samples . Transactions on ASAE , 17 ( 1 ) : 56 – 58 .
- Moresi , M. and Spinosi , M. 1980 . Engineering factors in the production of concentrated fruit juices. 1. Fluid physical properties of orange juices . J. Food Technol. , 15 : 265 – 276 .
- Rahman , M.S. 2009 . “ Thermal conductivity of foods ” . In Food properties handbook , 645 – 680 . Boca Raton : CRC Press .
- Delgado , A.E. , Gallo , A. , Piante , D.D. and Rubiolo , A. 1997 . Thermal conductivity of unfrozen and frozen strawberry and spinach . Journal of Food Engineering. , 13 ( 12 ) : 137 – 146 .
- Ali , S.D. , Ramaswamy , H.S. and Awuah , G.B. 2002 . Thermophysical properties of selected vegetables as influenced by temperature and moisture content . Journal of Food Process Engineering , 25 : 417 – 433 .
- Reidy , G.A. and Rippen , A.L. 1971 . Methods for determining thermal conductivity in foods . Trans. ASAE , 14 : 248 – 254 .
- Mohsenin , N.N. 1980 . Thermal properties foods and agricultural materials , New York : Gordon and Breach Science Publishers .
- Singh , R.P. 1982 . Thermal diffusivity in food processing . Food Technology , 2 : 87 – 91 .
- Choi , Y. and Okos , M.R. 1983 . The thermal properties of tomato juice concentrates . Transaction of ASAE , 26 : 305 – 311 .
- Liang , X.G. , Ge , X.S. , Zhang , Y.P. and Wang , G.J. 1991 . A convenient method of measuring the thermal conductivity of biological tissue . Phys. Med. Biol. , 36 ( 12 ) : 1599 – 1605 .
- Cheng , S.X. , Jiang , Y.F. and Liang , X.G. 1994 . A tiny probe for measuring the thermal conductivities of non-rigid materials . Measurement Science Technology , 5 : 1339 – 1344 .
- Telis-Romero , T. , Telis , V.R.N. , Gabas , A.L. and Yamashita , F. 1998 . Thermophysical properties of Brazilian orange juice as affected by temperature and water content . Journal of Food Engineering , 38 : 27 – 40 .
- Angela , F. and Dane , B. 1999 . Thermal properties of fruit juices as a function of concentration and temperature determined using the photopyroelectric method . Journal of the Science of Food and Agriculture , 79 : 1361 – 1366 .
- Sweat , V.E. 1995 . Engineering properties of foods , 2nd , Edited by: Rao , M.A. and Rizvi , S.S.H. 87 – 99 . New York : Marcel Dekker .
- Healy , J.J. , de Groot , J.J. and Kestin , J. 1976 . The theory of the transient hot-wire method for measuring thermal conductivity . Physica , 82C : 392 – 408 .
- Zhang , M. , Zhang , L.J. and Zhang , J. 2007 . Influence of temperature and soluble solids concentration on thermal conductivity of orange juice . Journal of Northwest A & F University , 35 ( 12 ) : 177 – 180 .
- Carslaw , H.S. and Jaeger , J.C. 1959 . Conduction of Heat in Solids , 1 – 52 . Oxford : Clarendon .
- Zhang , M. , Zhao , H.Z. and Xie , J. 2006 . Study on Measurement System of Thermal Conductivity of Vegetables and Fruits . Transactions of the Chinese Society of Agricultural Machinery , 37 ( 1 ) : 90 – 93 .
- Chen , Z.S. , Ge , X.S. and Gu , Y.Q. 1990 . Heat measuring technology and thermal properties measurement , 171 – 185 . Beijing : Chinese Science Technique Press .