Abstract
The influence of polysaccharide annealing temperature on interactions in acid-induced sodium caseinate (CAS), κ-carrageenan (CAR), and sucrose gels were studied. Gels were formed by glucone-δ-lactone (GDL) addition to mixed systems leading to a slow acidification towards the protein isoelectric point. The system properties were a result of phase separation between the biopolymers, simultaneously with gelation, resulting in a heterogeneous network with particulate structure. The results showed that gels with an increased whiteness index and water entrapped were obtained at higher caseinate concentration. Increasing caseinate and carrageenan concentration resulted in more brittle and elastic gels, whereas stress in rupture behaviour depended on the relative concentration of biopolymers. The depletion–flocculation phenomenon became evident at higher biopolymer concentration, resulting in network weakening and lower water release, although this effect was reduced with sucrose addition.
INTRODUCTION
Protein-polysaccharide mixtures are widely used in the food industry as they play an essential role in the structure, texture and stability of many foodstuffs.[Citation1] These quality attributes are of great interest in food formulation design and are closely related to protein-polysaccharide interactions. However, these biopolymer interactions are strongly influenced by composition and process parameters such as biopolymer concentration, dissolution temperature and also addition of co-solutes, such as salts or other lower molecular weight solutes like sugars.[Citation2] Therefore, in order to develop desirable properties in food products, knowledge of the mechanisms of biopolymer interactions in formulated foods and its relation with macroscopic attributes, such as texture or water retention capacity, is of great importance. Casein–carrageenan interactions have been widely studied because of their applicability in acidified milk beverages and milk desserts.
Sodium caseinate (Na-CN) is manufactured by adding acid to milk in order to precipitate the casein at its isoelectric point. The acid-coagulated casein is washed and then redissolved by adding an appropriate amount of sodium hydroxide to restore neutrality.[Citation3] Sodium caseinate is widely used as an ingredient in the food industry: its functional properties include emulsification, water and fat-binding, thickening, gelation, and whipping. Carrageenans are sulphated linear polymers, with a backbone of alternating α-1 and β-1,3 linked galactose residues and varying proportions and positions of sulphate groups.[Citation4] They can be classified into several basic types; one of them is κ-carrageenan. At high temperatures (>50°C, depending on salt concentration) κ-carrageenan exists in solution as a random coil. However, when the temperature is lowered sufficiently the polymer undergoes a transition from coil to helix. Formation of the helix and the subsequent aggregation of neighboring helices facilitates gelation.[Citation5] κ-carrageenan gel is strong, brittle and thermoreversible, but is subject to syneresis.[Citation6] Carrageenan was added in meat[Citation7] or cheese[Citation8] formulation in order to achieve fat reduction with no damage of texture attributes. The carrageenan addition was also associated with an increase in moisture retention in meat products.[Citation7] These effects were mainly attributed to electrostatic interaction and conformational transition temperature of carrageenan.
In biopolymer mixtures, two concomitant phenomena may occur and contribute to the structure of the system. The first is phase separation, with the classical distinction between associative and segregative phase separation. In associative phase separation one of the separating phases is enriched in both polymers, whilst in segregative phase separation, each phase is enriched in one of the polymers.[Citation9,Citation10] The second phenomenon is gelation of polymers, which depends on the mechanism and type of interaction involved between them. Thus, the structure and properties of the mixed systems are dependent on the balance between these two phenomena.[Citation11]
Acid gelation of milk proteins is of importance during the processing of dairy products, such as yoghurt-like desserts. Milk can be acidified by bacterial cultures (lactic fermentation) or by the addition of glucono-δ-lactone (GDL), which hydrolyzes to gluconic acid with a resulting reduction in pH. On lowering the pH from neutrality towards pI, the carboxylic acid groups of the sodium caseinate submicelles are neutralised, and the net attractive interactions (hydrophobic, unlike electrostatic, van der Waals, hydrogen bonding) cause strengthening of the protein association in an aqueous medium.[Citation12] The presence of sugar may modify the process of gelation,[Citation13] mainly by enhancing the degree of protein aggregation,[Citation14] since the direct contact between protein and water is considered thermodynamically unfavorable in the presence of sugars and this may be directly correlated with an enhancement of hydrophobic interactions.[Citation15]
To the best of authors' knowledge, no studies have been performed focusing on the temperature of carrageenan dissolution as a possible parameter affecting the characteristics of the gel formed. The mechanical properties of acidified sodium caseinate gels with the addition of xanthan and sucrose were studied, and the effects of molecular transitions on xanthan, caused by annealing at different temperatures were also evaluated by oscillatory rheology.[Citation16] Depending on the annealing temperature, xanthan transitions produced different structural characteristics in mixed gels, as observed by their relaxation time and residual stress. However, mechanical properties at equilibrium were affected by the strength of the interactions between caseinate and xanthan, while the amount of sucrose played an important role in the network formation of gels and on their final elasticity. Intermediate concentrations of sucrose yielded gels that were finer than those obtained with a low sucrose content, whereas with high amounts of this co-solute, the gels became more fragile. Therefore, the aim of this work was to understand the interactions between sodium–caseinate, sucrose, κ-carrageenan at different annealing temperatures of polysaccharide in acid-induced milk gels using uniaxial compression, water release and whiteness measurements. A central composite rotatable design (CCRD) was used to study the effect of variables on the material properties.
MATERIALS AND METHODS
Materials
The ingredients used to prepare the gels were casein (total protein 89.7 g/100 g powder, moisture 7.2 g/100 g powder wet basis), κ-carrageenan (moisture 8.4 g/100 g powder) and glucono-δ-lactone (GDL) purchased from Sigma-Aldrich Corporation (St. Louis, USA). Analysis of the material by atomic absorption gave the following ion composition (g/100g powder): 0.80 Na+, 0.40 Ca2+, and 0.40 K+ for sodium caseinate and 0.10 Na+, 0.70 Ca2+, and 2.70 K+ for κ-carrageenan. The analytical degree sucrose was purchased from Synth (São Paulo, Brazil). Double-distilled water was used for preparation of the solutions.
Preparation of the Gels
Sodium caseinate dispersions were prepared by dissolution of the casein in water with mild stirring at 25°C for 2 h. The caseinate powder was dissolved in 55% of the total water of the system and the pH was periodically adjusted to 7.0 with NaOH 40% up to formation of sodium caseinate. Carrageenan was dissolved in the remaining 45% water by mechanical stirring at different temperatures for 30 minutes. The caseinate or carrageenan stock solutions were then mixed by mechanical stirring at the same temperature as carrageenan dissolution. Sucrose was added just after the mixture of the stock solutions. The temperature was decreased to 35°C and GDL powder was gently added to the casein-carrageenan solutions, then rapidly homogenised by mechanical stirring before being cooled with an ice bath down to 10°C. The relation of [GDL]/[casein] used was 0.16. The formulations were made according to a quadratic fully factorial design. shows the range and levels of caseinate (CAS), carrageenan (CAR) and sucrose (SUC) concentration, as well as annealing temperature (T), studied in this work. This design called for a total of 27 experiments, including 24 factorial points, three central points and eight extra axial points. The mixed solutions were poured into cylinder plastic tubes (30 × 30 mm) for compression tests, into centrifuge tubes for water holding analysis, or into Petri plates (φ = 90 mm) for gel whiteness measurements. Samples were stored at 10°C for 48 h before the analyses in order to reach approximately casein pI. The mechanical properties (σrup, εrup, and E), whiteness index (WI) and the value of water release (WR) were taken as the results to develop response surfaces using a quadratic model for statistically significant variables. Analysis of variance was used to detect significant effects at p < 0.10. The Statistica software package v. 6.0 (Statisoft Inc., Tulsa, USA) was used to analyze the results.
Table 1 Range of independent variables (g/100 g of gel) used in the experimental design
Mechanical Properties
Gels were compressed to 80% deformation using a Universal Testing Machine - TA.XT2 Texture Analyzer (Stable Micro Systems, England) with an acrylic cylindrical plate (35 mm in diameter) lubricated with silicon oil to avoid friction between sample and geometry. The crosshead speed was 1 mm/s. Tests were carried out in triplicate at 10 ± 1°C. Stress (σ) and Hencky strain (εH) were calculated from the force-deformation data according to EquationEqs. (1) and Equation(2), respectively.
Water Released (WR) from Gels
The water released from the gels was expressed as the relation between the volume of the liquid released from the gel by the volume of the solid phase after centrifuging. Greater WR values mean more liquid, or serum was released from the gels.[Citation13] The centrifuge utilized was a DuPont model Sorvalle RC 26 Plus, rotor SLA1500 (Du Pont, UK). Samples of 50 g at 10°C were centrifuged from 400 to 10,000 g-force at 5 steps of 10 min each (400, 1500, 3500, 6200 e 10,000 g). Measurements were made in duplicates.
Whiteness Measurement
The reflective surface of gels was evaluated instrumentally with a Color Quest II – Hunter Lab (USA) spectrophotometer with a D65 illuminant as the reference, at an observation angle of 10°. The equipment was calibrated with white (C6299; 03/96; X = 77.46; Y = 82.08; Z = 88.38) and grey (C6299G; 03/96; X = 47.71; Y = 50.83; Z = 54.94) standards. The parameters L* (lightness), a* (redness) and b* (yellowness) were obtained based on the system developed by the Commission Internationale de l'Eclairage (CIE). Measurements were made in triplicate at 10°C. The L*, a* and b* parameters were then converted to whiteness index (WI) values[Citation18,Citation19] according to EquationEq. (3):
RESULTS
Water release, whiteness index, elasticity modulus, stress and strain at rupture were obtained for acid gels prepared with different concentrations of ingredients and annealing temperature of the polysaccharide. shows the results obtained as a function of the independent variables. In general the increase of sodium caseinate concentration yields brittle gels (minor strain at rupture values), with greater liquid exudation (increase in WR values). The increase in carrageenan concentration led to gels, which were also less deformable and more translucid, but more elastic (increase in elasticity modulus). Such a result could be correlated to an increase in biopolymer interactions in the acidified conditions, which led to a strengthening of the gel structure. Stress at rupture behaviour did not show a clear trend as to biopolymer concentration as observed in .
Table 2 Coded variables for the experimental design 2(4 ) and responses obtained for mechanical properties, color parameters and water release WR
Ribeiro et al.[Citation2] studied similar gels, but by a different method: the carrageenan was dissolved at a single temperature (45°C) and the gels were added with a different ratio of sucrose and sucralose. In this study, the increase in biopolymer concentrations of pure caseinate or carrageenan gels led to synergistic effects on mechanical properties, although for mixed biopolymer systems the increase in biopolymer concentration led to a reduction in gel hardness. Such behaviour was attributed to repulsive interactions amongst biopolymers due to excluded volume effects. Similar gels, also containing whey proteins were studied by Sabadini et al.[Citation20] In such systems, the protein and carrageenan mixtures were subjected to different temperatures (30 to 80°C) prior to acidification with the addition of GDL. Sodium caseinate and carrageenan concentration exerted the most important effects on rheological properties with gels with and without sucrose. Heat treatment temperature also exerted an important positive effect on mechanical properties of the gels, especially for systems with no addition of sucrose.
The multivariate analysis allowed us to obtain the regression coefficients of the mathematical models used to describe the behaviour of the gel properties. EquationEquations (4) Equation–Equation(6) show the mathematical models of the mechanical properties (elasticity modulus, stress and strain at rupture, respectively). EquationEquations (7) and Equation(8) show the mathematical models obtained for water release and whiteness index, respectively.
The correlation coefficients (R2) were not so good. Such values correspond to 0.62 for elasticity modulus, 0.70 and 0.73 for stress and strain at rupture and 0.68 and 0.90 for water release and whiteness index. However, the F-test showed that the models were reliable (FCalc/FTab > 1) allowing us to obtain surface responses to describe the gels' properties.
Failure stress of acid κ-carrageenan-sodium caseinate gels showed a complex behaviour since such mechanical property was dependent on biopolymer concentration and also on the dissolution temperature of polysaccharide (EquationEq. (5) and ). This latter variable could be contributing to a structural modification of mixed gels even though the carrageenan network is formed as a result of helical aggregation only if the temperature is sufficiently lowered so that the polymer undergoes a transition from coil to helix.[Citation21] In , it can be observed that at annealing temperature of 40°C harder gels were obtained in two different conditions of biopolymer pairs of concentration: at the lowest carrageenan concentration (0.3 g/100 g gel) with 10 g/100 g gel of caseinate (highest protein concentration) or the lowest caseinate concentration (2 g/100 g gel) and the highest carrageenan content. A similar tendency was observed at an increased temperature of polysaccharide annealing ( and C), although the harder gels were observed only at the highest polysaccharide concentration and smallest content of sodium caseinate. Aside from the strong influence of polysaccharide dissolution temperature on failure stress, this process condition property did not significantly influence elasticity modulus or failure strain (EquationEqs. (4) and Equation(6), respectively).
Figure 1 Response surfaces of stress at rupture (σR) as a function of κ-carrageenan (CAR) and sodium caseinate (CAS) concentration. (A) T: 40°C; (B) T: 65°C; and (C) T: 90°C.
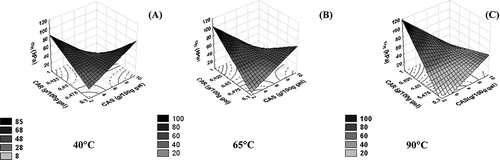
The response surface plots for failure strain and elasticity modulus as a function of carrageenan and sodium caseinate concentration are shown in and B, respectively. More deformable gels (strain at failure about 70%) were obtained at lower protein concentration (2 g/100 g gel of sodium caseinate), showing the relevance of polysaccharide network on the deformability or strengthening of gels. However, an increase in concentration of both biopolymers led to a strong decrease of failure strain, reaching values near 15%, which is characteristic of brittle and particle gels (). In a way different from that observed for failure stress, greater values of elasticity modulus were observed at increased carrageenan and sodium caseinate concentration (B). These could be a result of the increase of protein-polysaccharide interactions at acidic conditions, or that carrageenan was more relevant to gel strength (EquationEq. 4) probably due to a prevailing polysaccharide network. Such behavior was also previously observed concerning the viscoelastic properties of kappa-carrageenan casein micelles aggregates formed in skimmed milk under shear.[Citation22] In this way, the differences observed between elasticity modulus and stress at rupture attributes could be related to the fact that the elasticity modulus was measured under small deformations and would thus be more related to biopolymers interactions. However, the stress and strain at fracture (large deformation properties), would be more related to resistance of the gels' structure.
Figure 2 Response surface of (A) strain at rupture (εR) and (B) elasticity modulus (E) as a function of κ-carrageenan (CAR) and sodium caseinate (CAS) concentration.
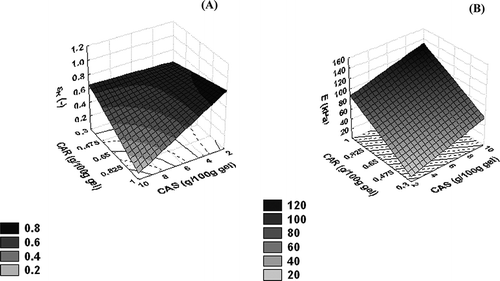
shows the surface responses of water release (WR) as a function of carrageenan-caseinate concentration at different sucrose concentrations. The gels that displayed more liquid release were obtained at a higher sodium caseinate concentration. It was also observed that the gels released more liquid at lower carrageenan concentration if the sucrose concentration was low (), but the increase in sucrose concentration shifted this pattern to the highest carrageenan concentration ().
Figure 3 Response surfaces of water release (WR) as a function of κ-carrageenan (CAR) and sodium caseinate (CAS) concentration at (A) 5 g/100 g gel, (B) 10 g/100 g gel, and (C) 15 g/100 g gel of sucrose (w/w%) concentration.
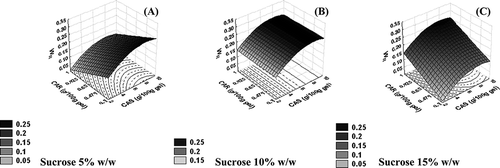
The significant regression coefficients on the whiteness index of gels are shown in EquationEqu. (8). It is observed that such a property was more influenced by the casein concentration, with the only positive linear effect observed. shows the whiteness index as a function of carrageenan and caseinate concentration. It is possible to observe that gel exhibited more whiteness (around 80 WI values) at the highest caseinate concentration (10 g/100 g gel). Even though the carrageenan exerted some effect on gel whiteness, the influence of the polysaccharide was minor on gel colour.
Figure 4 Surface response of whiteness index (WI) as a function of sodium caseinate (CAS) and carrageenan (CAR) concentration.
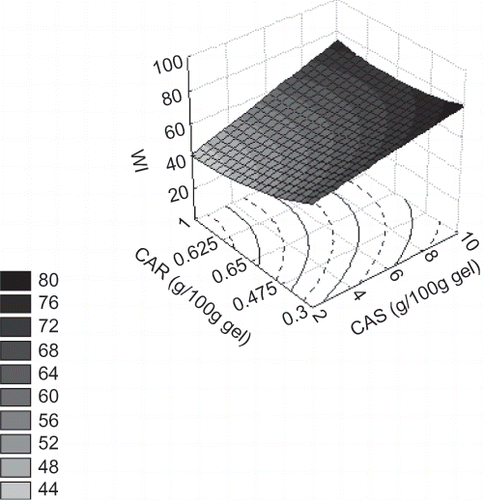
In , it is possible to observe the influence of the other variables on whiteness of the gels. Whiteness was related linearly with caseinate concentration in relation to polysaccharide dissolution temperature or sucrose ( and C), while this relation was non-linear for carrageenan concentration ( and D). A higher whiteness index was observed at higher caseinate concentration (10 g/100 g gel) and lower carrageenan concentration (0.3 g/100 g gel) and both at lower annealing temperature and lower sucrose content (40°C and 5 g/100 g gel of sugar).
DISCUSSION
The properties of the systems were a consequence of the interactions between the several variables used for the gel composition, modifying the process of network formation and its stability. In this way, the systems' properties can be analysed in terms of simultaneous factors occurring: 1-phase separation attributed to thermodynamic incompatibility among the biopolymers at initial acidification pH far from the pI and 2-gelation process. The polysaccharide annealing temperature and the presence of other components such as sucrose seem to have a great influence on the balance of the kinetic processes (phase separation and gelation), since this could change the kind of biopolymer interactions with each other and with water in the system.[Citation12,Citation23–25 However, the system properties could also be distinguished in terms of three parameters associated with the gelation: (A) slow acidification as result of the GDL hydrolysis at low temperature, (B) carrageenan gelation as a consequence of temperature reduction below the helix-coil transition and (C) an interaction between carrageenan and caseins as a result of specific electrostatic interactions.
In a primary analysis, the gelation of the systems was a consequence of GDL addition, which led to a gradual pH decrease, causing protein aggregation and also possible protein-polysaccharide electrostatic interactions near the pI. However, despite the fact that both carrageenans and caseins are globally negatively charged at neutral pH (beginning of the process), a specific interaction was suggested between the sulphate groups of the carrageenan and a very short, positively-charged region situated between the residues 97 and 112 of κ-casein, at the surface of the casein micelle.[Citation26,Citation27] Moreover, an acidification process occurred at 10°C or below the coil-to-helix transition temperature, which also promoted the gelation of κ-carrageenan through the interactions between helices formed from both free and bound (to sodium caseinate) polysaccharide molecules. However, caseinate and carrageenan were mixed at the annealing temperature of polysaccharide, which could also lead to interactions among the biopolymers facilitated by the carrageenan in the coil conformation. Thus, two gelation processes occurred simultaneously at different rates: one associated with protein aggregation promoted by acidification and the other related to the carrageenan gelation. In addition, the pH decrease led to a modification of the protein's net charge, which could be related to an increase of the interaction between protein (less negatively charged at lower pHs) and the anionic polysaccharide, forming complex coacervates that have little affinity with water.[Citation28] On the other hand, at the beginning of the process the system stayed for a certain period of time at higher pH values, far above the casein isoelectric point, which was higher with a decreased GDL concentration added.[Citation29,Citation30] As a result, phase separation between the caseinate (mainly negatively charged in such a pH condition) and the anionic carrageenan occurred,[Citation5,Citation31] with protein and polysaccharide rich domains formed before the system's gelation.[Citation10,Citation28] Moreover, after the biopolymer mixture κ-carrageenan was probably at coil conformation (T ≥ 40°C) and in such a condition carrageenan would not be able to stabilize sodium caseinate from polysaccharide phase separation,[Citation25] as a result of the greater molecular mobility or lower viscosity prior to the polysaccharide gelation. Therefore, the mixed systems were probably formed by an acid caseinate gel surrounded by a self-associated carrageenan network,[Citation5] which is a characteristic of a heterogeneous interconnected or phase-separated network,[Citation11] which could explain the stress at rupture results. A strong synergism between these two biopolymers occurred at small concentrations of protein, but incompatibility between the carrageenan and the protein was evident with an increased sodium caseinate concentration[Citation2] resulting in weaker gels (), but with a higher whiteness index (), since this was mainly associated with the protein component. A higher temperature of polysaccharide annealing promoted the formation of a strong and more interconnected carrageenan network, possibly involving the caseinate domains, especially at lower concentrations of sodium caseinate. As a result, stronger gels () with opaque appearance (reduced whiteness index) were formed () especially at higher carrageenan concentrations.
Synergism at lower protein concentrations could be explained by the formation of a protein-protein network promoted by acidification, polysaccharide-polysaccharide aggregation caused by helix-coil transition, and specific interactions between κ-casein and κ-carrageenan,[Citation32] especially favoured at higher annealing temperatures. At higher casein concentrations, phase separation was favored and casein did not tend to form a continuous network, resulting in a weaker gel[Citation33] with more water released (). However, the increase in liquid release (increase in WR values) could also be a result of the complex coacervation (stronger interactions amongst the biopolymers) favored at higher temperatures and at pH values near the isoelectric point. This is evident in , because stress at rupture values was higher at higher annealing temperatures. On the other hand, enhanced casein concentration forms gels with junction zones of higher density, resulting in higher elasticity modulus (), which was responsible for a diminished capacity of network mobility, leading to less deformable gels ().
CONCLUSIONS
Multivariate analysis was a valuable tool in studying systems with different compositions. The main contribution of multivariate analysis was to allow the separation of the main effects of the system components and process of gel formation on each property evaluated. The system properties were a result of the combination between different simultaneous processes correlated to phase separation attributed to thermodynamic incompatibility between the biopolymers and system gelation. The latter could also be distinguished in terms of slow acidification due to GDL addition and a composite network formation (casein or carrageenan) due to electrostatic interactions favoured by carrageenan in the coil state. Increasing casein concentration resulted in more brittle and less elastic gels, whereas the increase in carrageenan concentration led to harder but less elastic gels. The enhancement in biopolymer concentrations could be associated to the depletion–flocculation phenomenon, which resulted in weaker and less elastic gels, leading also to a higher liquid release.
REFERENCES
- Hemar , Y. , Hall , C.E. , Munro , P.A. and Singh , H. 2002 . Small and large deformation rheology and microstructure of κ - carrageenan gels containing commercial milk protein products . International Dairy Journal , 12 : 371 – 381 .
- Ribeiro , K.O. , Rodrigues , M.I. , Sabadini , E. and Cunha , R.L. 2004 . Mechanical properties of acid sodium caseinate-κ-carrageenan gels: effect of co-solute addition . Food Hydrocolloids , 18 : 71 – 79 .
- Kinsella , J.E. 1984 . Milk proteins: Physicochemical and functional properties . Critical Reviews in Food Science and Nutrition , 3 : 197 – 262 .
- Imeson , A. Carrageenan . 2000 . Handbook of hydrocolloids , Edited by: Phillips , G.O. and Williams , P.A. 105 – 121 . Boca Raton : CRC Press .
- Spagnuolo , P.A. , Dalgleish , D.G. , Goff , H.D. and Morris , E.R. 2005 . Kappa-carrageenan interactions in systems containing casein micelles and polysaccharide stabilizers . Food Hydrocolloids , 19 : 371 – 377 .
- Yuguchi , Y. , Thuy , T.T.T. , Urakawa , H. and Kajiwara , K. 2002 . Structural characteristics of carrageenan gels: temperature and concentration dependence . Food Hydrocolloids , 16 : 515 – 522 .
- Modi , V.K. , Yashoda , K.P. and Naveen , S.K. 2009 . Effect of Carrageenan and Oat Flour on Quality Characteristics of Meat Kofta . International Journal of Food Properties , 12 : 228 – 242 .
- Totosaus , A. and Guemes-Vera , N. 2008 . Effect of κ- and l-carrageenans as fat-replacers in low-fat oaxaca cheese . International Journal of Food Properties , 11 : 656 – 668 .
- Burey , P. , Bhandari , B.R. , Rutgers , R.P.G. , Halley , P.J. and Torley , P.J. 2009 . Confectionery Gels: A Review on Formulation, Rheological and Structural Aspects . International Journal of Food Properties , 20 : 176 – 210 .
- Syrbe , A. , Bauer , W.J. and Klostermeyer , N. 1998 . Polymer science concepts in dairy systems - An overview of milk protein and food hydrocolloid interaction . International Dairy Journal. , 8 : 179 – 193 .
- Morris , V.J. 1995 . “ Synergistic interactions with galactomannans and glucomanans ” . In Biopolymer Mixtures , Edited by: Harding , S.E. , Hill , S.E. and Mitchell , J.R. 289 – 314 . Nottinghan, , UK : Nottingan University Press .
- Belyakova , L.E. , Antipova , A.S. , Semenova , M.G. , Dickinson , E. , Merino , L.M. and Tsapkina , E.N. 2003 . Effect of sucrose on molecular and interaction parameters of sodium caseinate in aqueous solution: relationship to protein gelation . Colloids and Surfaces B: Biointerfaces , 31 : 31 – 46 .
- Schkoda , P. , Hechler , A. and Kessler , H.G. 1999 . Effect of minerals and pH on the rheological properties and syneresis of milk-based acid gels . International Dairy Journal , 9 : 269 – 273 .
- Kulmyrzaev , A. , Bryant , C. and McClements , D.J. 2000 . Influence of sucrose on thermal denaturation, gelation and emulsion stabilization of whey proteins . Journal of Agricultural and Food Chemistry , 48 : 1593 – 1597 .
- Dickinson , E. and Merino , L.M. 2002 . Effect of sugars on the rheological properties of acid caseinate—stabilized emulsion gels . Food Hydrocolloids , 16 : 321 – 331 .
- Braga , A.L.M. and Cunha , R.L. 2004 . The effects of xanthan conformation and sucrose concentration on the rheological properties of acidified sodium caseinate-xanthan gels . Food Hydrocolloids , 18 : 977 – 986 .
- Kohyama , K. and Nishinari , K. 1993 . Rheological studies on gelation process of soybean 7S and 11S proteins in the presence of glucono - delta - lactone . Journal of Agricultural and Food Chemistry , 41 : 8 – 14 .
- Joiner , A. , Hopkinson , I. , Deng , Y. and Westland , S. 2008 . A review of tooth colour and whiteness . Journal of Dentistry , 36s : s2 – s7 .
- Briones , V. and Aguilera , J.M. 2005 . Image analysis of changes in surface color of chocolate . Food Research International , 38 : 87 – 94 .
- Sabadini , E. , Hubinger , M.D. and Cunha , R.L. 2006 . The effects of sucrose on the mechanical properties of acidic milk proteins-κ-carrageenan gels . Brazilian Journal of Chemical Engineering , 23 : 55 – 65 .
- Rochas , C. and Rinaudo , M. 1984 . Mechanism of gel formation in κ-carrageenan . Biopolymers , 23 : 735 – 745 .
- Ji , S. , Corredig , M. and Goff , H.D. 2008 . Aggregation of casein micelles and kappa-carrageenan in reconstituted skim milk . Food Hydrocolloids , 22 : 56 – 64 .
- Penroj , P. , Mitchell , J.R. , Hill , S.E. and Ganjanagunchorn , W. 2005 . Effect of konjac glucomannan deacetylation on the properties of gels formed from mixtures of kappa carrageenan and konjac glucomannan . Carbohydrate Polymers , 59 : 367 – 376 .
- Bayarri , S. , Durán , L. and Costell , E. 2004 . Influence of sweeteners on the viscoelasticity of hydrocolloids gelled systems . Food Hydrocolloids , 18 : 611 – 619 .
- Vega , C. , Dalgleish , D.G. and Goff , H.D. 2005 . Effect of k-carrageenan addition to dairy emulsions containing sodium caseinate and locust bean gum . Food Hydrocolloids , 19 : 187 – 195 .
- Dalgleish , D.G. and Morris , E.R. 1988 . Interactions between carrageenans and casein micelles: electrophoretic and hydrodynamic properties of the particles . Food Hydrocolloids , 2 : 311 – 320 .
- Snoeren , T.H.M. , Payens , T.A.J. , Jeunink , J. and Both , P. 1975 . Electrostatic interaction between κ-carrageenan and κ-casein . Milchwissenschaft , 30 : 393 – 396 .
- de Jong , S. and van de Velde , F. 2007 . Charge density of polysaccharides controls microestucture and large deformation properties of mixed gels . Food Hydrocolloids. , 21 : 1172 – 1187 .
- Cavallieri , A.L.F. and Da Cunha , R.L. 2008 . The effects of acidification rate, pH and ageing time on the acidic cold set gelation of whey proteins . Food Hydrocolloids , 22 : 439 – 448 .
- Braga , A.L.M. , Menossi , M. and Cunha , R.L. 2006 . The effect of the glucono-delta-lactone/caseinate ratio on sodium caseinate gelation . International Dairy Journal , 16 : 389 – 398 .
- Bourriot , S. , Garnier , C. and Doublier , J.L. 1999 . Micellar-casein- κ-carrageenan mixtures. I. Phase separation and ultrastructure . Carbohydrate Polymers , 40 : 145 – 157 .
- Oakenfull , D. , Miyoshi , E. , Nishinari , K. and Scott , A. 1999 . Rheological and thermal properties of milk gels formed with κ - carrageenan. I. Sodium caseinate . Food Hydrocolloids , 13 : 525 – 533 .
- Capron , I. , Nicolai , T. and Smith , C. 1999 . Effect of addition of κ-carrageenan on the mechanical and structural properties of b - lactoglobulin gels . Carbohydrate Polymers , 40 : 233 – 238 .