Abstract
This work presents the compressive strength properties of sponge gourd (Luffa aegyptica) seeds to facilitate the design or adaptation of an appropriate dehuller. The sizes and shape indices of the seed and kernel, and the clearance of the kernel from the seed coat were determined. The seed samples were subjected to uni-axial quasi-static compression tests at 1.0 mm min−1 along the minor and the major axes. The fracture resistance, stiffness modulus, modulus of elasticity, toughness, and maximum elastic deformation of the seed were obtained from the force-deformation curve. The geometric mean diameters varied from 4.0 to 4.5 mm for the seed and 1.6 to 3.9 mm for the embedded kernel; while their corresponding sphericities were 0.64 and 0.62, respectively. The compressive strength of the seed varied with loading orientation. The seed exhibited larger deformation but lower stiffness along the major axis than the minor axis. The force required for rupturing the hull were 95 N along the major axis and 81 N along the minor axis; while the corresponding energy required were 95 and 40 mJ.
INTRODUCTION
Sponge gourd (Luffa aegyptiaca) fruit is a composite biomaterial consisting of a fibrous mesh of seed-bearing vascular bundle, inside an oblong pod. The mature pod is covered by a thin crispy exocarp. The encased fibrous mesh is often used as domestic biodegradable sponge.[Citation1] The encased fibrous mesh is often used as domestic biodegradable sponge[1] and as filler material in the manufacture of padded packaging, sound and thermal insulator, and water and oil filters.[Citation2] In some places in Africa and Asia, the whole unripe pods are used in traditional medicine.[Citation3] At maturity, the dry fruit breaks at the distal end, creating an aperture through which the loose miniature seeds (ranging from 30 to 250 or more seeds per fruit) are shaken out.[Citation4] The hull, which comprises about 50% of the seed weight, contains mostly crude fiber and an insignificant quantity of fat.[Citation5] The embedded kernel contains about 39, 33, 2.7, 14 and 5 g/100 g kernel of crude protein; crude fat; crude fiber; carbohydrate and ash, respectively.[Citation4] Although, sponge gourd seed is rich in essential ingredients for human and livestock feeds and some industrial uses, it is hitherto, usually discarded. The miniature size of the seed might have been part of the reasons for the apparent lack of interest in its processing. As the domestication of lesser known and underutilized species of plants for the diversification of agriculture keeps increasing, it is expected that the development of an appropriate dehuller would complement the utilization and economic value of sponge gourd seeds.
The principle of elastic deformation in shearing the seed coat between compression surfaces has been employed in the design of many existing dehullers, crackers and decorticators.[Citation16–10] For some cucurbitaceae seeds, mechanical oil expression from the whole seed causes rapid wear of machine parts, decreases the oil yield potential and reduces the quality of oil and the de-fatted meal; whereas, oil expression from dehulled seeds reduces wax, improves oil colour and yield and increases the protein content of the defatted meal.[Citation11] Furthermore, the knowledge of the deformation and fracture of both the hull and the kernel under compressive loading plays a very significant role in the dehulling of agricultural seeds. Important mechanical properties, which are vital for the design of an effective and efficient dehuller, to minimize mechanical losses, can be estimated from the force-deformation curve. For example, the average deformation of groundnut pod under uni-axial compression was identified as an important parameter in the specification of concave clearance, in the development of a decorticator.[Citation9] The fracture resistance of whole soybeans, indicating seed coat rupture, was estimated from the force-deformation curve for soybean under quasi-static compressive loading.[Citation12] For roasted cashew nuts, cracking force was estimated from the force-deformation curve using that as a basis for estimating wholesomeness.[Citation13] It was therefore expected that the deformation characteristics of sponge gourd seeds would provide significant information on its dehulling.
It is also well known that the fracture resistance of a wide range of agricultural seeds and nuts depend on their orientations against the applied compressive loads and the case is not different for most cucurbitaceae seeds due to their broadly similar shape indices.[Citation14] The orientation of the seed inside the dehuller during impact can affect the magnitude of the pericarp breakage during fracture. The ability of the pericarp to break and to separate from the seed, defined as hullability is higher, if impact is longitudinal or transverse rather than being radial, on the convex sides.[Citation14] The magnitude mainly depends on the structural properties of the seed such as thickness of the pericarp,[Citation15,Citation16] size[Citation5] and oil content of the embedded kernel.[Citation17,Citation18] For melon seeds, it was observed that the load required for opening the different types of hull was lowest near the sharp tip.[Citation19] The hull breaking load was lowest for the long melon and highest for the watermelon seeds. For pumpkin seeds, no appreciable variation was observed in energy absorbed during rupture failure in the horizontal and vertical loading orientations.[Citation20] When sunflower seeds were loaded in the horizontal direction hull cracks were developed at a lower force than those loaded in the vertical direction..[21] Similarly, for karingda (Citrullus lanatus) seeds, higher energy was required to rupture the hull when the seed was compressed in the vertical (45–67 mJ) than in the horizontal (12–36 mJ) orientation.[Citation8] For almonds, size and loading orientation had significant effect on cracking force; the highest rupture strength occurred when the nuts were loaded along the horizontal axis.[Citation22] Cumin seeds loaded in the vertical orientation required more energy for rupture than that in the horizontal orientation. Maximum energy absorbed was found to be 15 and 20 mJ in the horizontal and vertical orientations respectively.[Citation23] For pine nuts, cracking force for loading on the minor, major and intermediate axis were 430, 64, and 470 N, respectively.[Citation24] Cashew nut was reported to have exhibited the least fracture force when compressed parallel to the minor axis, along the natural line of cleavage.[Citation13] Similar patterns were observed in the fracture behavior of dika nuts,[Citation25] filbert nuts[Citation26] and palm nut, which has no natural cleavage.[Citation27] In contrast, the fracture loads for macadamia nut were roughly the same, regardless of the loading direction, because the structure of the nutshell is reasonably isotropic and uniform.[Citation28,Citation29] When soybeans were loaded in the vertical hilum position a consistent seed coat cracks was observed at lower force than when loading was in the horizontal hilum position.[Citation12] The objectives of this work were to determine the average compressive force, deformation, and toughness at seed coat rupture, of sponge gourd seeds under quasi-static loading, as a function of loading orientation. This was with the view to provide insight into the mechanics of dehulling of sponge gourd seeds, with minimal kernel breakage.
MATERIALS AND METHODS
Twenty matured and sufficiently dried sponge gourd fruits were harvested from the wild, on the Teaching and Research Farm, Obafemi Awolowo University, Ile-Ife, Nigeria. The embedded seeds were extracted manually, sun-dried and sorted, to remove foreign matter, along with broken and immature seeds.
At the time of the experiments, the moisture content of the seeds was determined using the AOAC official method.[Citation30] The three principal dimensions of 100 randomly selected seeds were measured using a vernier caliper. The seed coats were then carefully removed manually, to extract the kernels for similar measurements. Seed hull ratio was estimated as a ratio of the weight of kernel to the hull. The clearance representing the shrinkage of the kernel from the seed coat was estimated as the difference between the diameters of the seed and the embedded kernel. The geometric mean diameters and the sphericities of the seeds and the kernels were determined from the principal dimensions. Sphericity (Sp ) is defined[Citation31] as:
Figure 1 The Instron Universal Testing Machine (Model 3369) used for compression tests. (Figure provided in color online.)
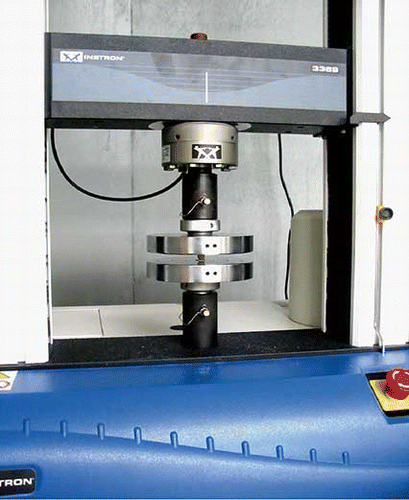
Figure 2 Sponge Gourd Seed Placement during Quasi-static compression tests (a) along minor axis and (b) along major axis.
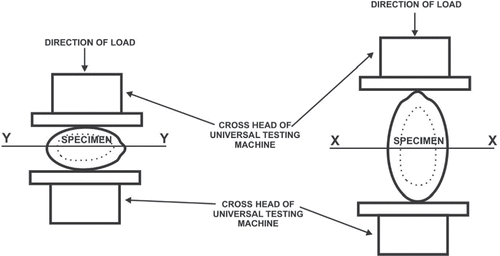
The stiffness modulus (S) is the slope of the force-deformation curve, in the apparent elastic region measured in kN m−2. It was assumed that the deformation of the seed coat alone prevailed up to when the kernel was contacted. Thereafter, provided the seed coat was still intact, the seed under compression behaved as a composite biomaterial with two elastic bodies in parallel compression. This change would result in a deviation of the slope of the force-deformation curve and, this point was taken as the limit for estimating the stiffness modulus for the seed coat.
Toughness is defined as the energy absorbed per unit of seed volume, prior to seed coat rupture in mJ m−3.[Citation27,Citation31,Citation33,Citation34] The energy absorbed per unit volume was estimated by the area under the curve up to the rupture point. Assuming that the change in contact area (Ao ) is proportional to the change in applied force (F), based on Hertz theory,[Citation31,Citation35] the Young modulus of elasticity (E) was calculated from:
The contact area was approximated as the cross-section through an equivalent ellipsoid at a distance of maximum elastic deformation (D) of the seed from its surface. When the seed is compressed along the major axis, the area is given by:
RESULTS AND DISCUSSION
Geometric Characteristics
The moisture content of the seeds and the embedded kernels at the time of the experiment were 8.4 and 9.8 g/100 g sample respectively. The mean geometric diameters of the seeds and the kernel were 4.5 and 2.8 mm, respectively. It was observed that the kernel moved with discernible sound in the seed coat when the seed was shaken. This implies that, the kernel is clearly separated from the seed coat when the seed is properly dried. As shown in , the diametric clearance, between the kernel and the wall of the seed coat was greatest along the major axis, with a mean value of 2.6 mm; while, it was least, 0.8 mm, along the minor axis. The minimum clearance between the tapered end to the compression surface needed to dehull the seed in a dehuller equals the minor diameter minus the deformation at seed coat rupture. The shrinkage of the embedded kernel from the seed coat enhances seed coat rupture with minimal kernel breakage. Similar features were reported for dika nut[Citation25] and palm kernel.[Citation27] Sponge gourd seed and the embedded kernel exhibited sphericities of 0.64 and 0.62, respectively; thus, appearing more like ellipsoids than being spheres.
Table 1 Geometric characteristics of sponge gourd seed and kernel.Footnote*
Compressive Strength Properties
The quasi-static deformation characteristics of sponge gourd seeds are shown in , for compressions along the minor and major axes of the seeds. The mechanical properties of the seeds, including the force and deformation at the rupture point, stiffness, modulus of elasticity, and the energy used for rupture (toughness) presented in , were extracted from .
Table 2 Mechanical properties of sponge gourd seed under uni-axial compression test.Footnote*
Figure 3 Average* Force-Deformation Curves of Sponge Gourd Seeds under Quasi-Static Compressions at 1.00 mm/min along the minor and major axes. *Plotted values are averages of ten determinations. (Figure provided in color online.)
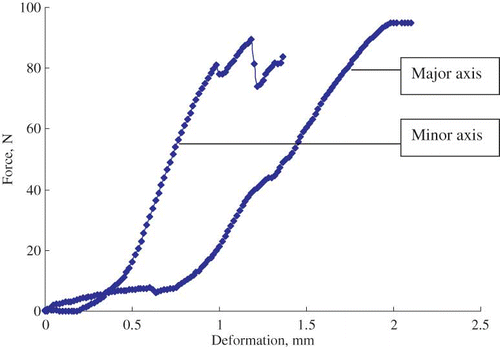
The force needed for seed coat rupture along the major axis, 95 N is greater than 81 N along the minor axis. This may be due to the resistance of the spongy thickened end of the point of attachment of the seed at the root of the major axis. Similar trends have been reported for sunflower seeds with 59 and 50 N along the vertical and horizontal axis, respectively.[Citation21] Furthermore, the magnitude of the forces in both loading directions are comparable with the values reported for soybeans under uni-axial compressive loading.[Citation12]
The deformation of the seed at rupture is higher along the major axis than along the minor axis, and it is less than the clearance between the kernel and the seed coat, in the same orientation. Thus, the seed coat ruptured before the kernel was contacted, when loaded along the major axis. Such feature enhances minimal kernel breakage. In contrast, compression along the minor axis shows that the kernel may be compressed before the seed coat fails. Although the deformation at seed coat rupture may exceed the clearance when compressed along the minor axis, the energy required for rupturing the seed coat, 40 mJ is less than 95 mJ required along the major axis. However, the energy is less than 120 N mm reported for Jatropha nut[Citation36] and it is higher than 0.4 mJ required for soybean.[Citation12] Consequently, the design of an appropriate dehuller, or a modification of an existing machine is necessary.
It is interesting to note that the stiffness modulus is higher along the minor axis than along the major axis. It is likely that the resistance is enhanced by the added resistance of the oil inside the kernel, due to the incompressibility of fluid; whilst, resistance along the major axis is due to the stiffness of the seed coat mainly. This observation further strengthens the indication of lower fracture energy along the minor axis, because the stress that caused the seed coat fracture was due to the externally applied load and the internal pressure due to the compressed oil. Along the major axis, only the external compressive load prevailed. The incompressibility of the embedded oil greatly reduced elastic deformation along the minor axis, hence, the low modulus of elasticity. This suggests the need to avoid excessive pressure when dehulling the seed in its natural rest position, to prevent simultaneous oil expression.
CONCLUSION
Compressive strength properties of sponge gourd seeds were determined from its deformation characteristics under quasi-static loading between two parallel plates. The results showed that orientation of the seed during compression had strong influence on resistance to seed coat rupture. Loading either along the major or minor axis showed appreciable elastic deformation from which significant parameters for machine design were derived. Sponge gourd seeds compressed along the minor axis, which is in the natural rest position, required less energy for seed coat rupture than the seeds compressed along the major axis.
REFERENCES
- Dairo , F.A.S. , Aye , P.A. and Oluwasola , T.A. 2007 . Some Functional Properties of Sponge Gourd (Luffa cylindrical L, M.J. Roem) . Journal of Agriculture, Food & Environment , 5 ( 1 ) : 97 – 101 .
- Christman, S. Luffa aegyptiaca. 2001. http://www. Floridata.com/lists/vine.cfm (http://www. Floridata.com/lists/vine.cfm) (Accessed: 23 April 2009 ).
- Martin, F.W. Cucurbit Seeds as Possible Oil and Protein Sources. Echo Technical Note. 1984. http://www.echonet.org (http://www.echonet.org) (Accessed: 25 March 2009 ).
- Ogunsina , B.S. , Adegbenjo , A.O. and Opeyemi , O. 2009 . Compositional, Mass-Volume Area Related and Mechanical Properties of Sponge Gourd (Luffa aegyptiaca) Seeds . International Journal of Food Properties , 13 : 864 – 876 .
- Tranchino , L. , Melle , F. and Sodini , G. 1984 . Almost Complete Dehulling of High Oil Sunflower Seeds . Journal of the American Oil Chemists Society , 61 : 1261 – 1265 .
- Engleson , J.A. and Fulcher , R.G. 2002 . Mechanical Behavior of Oats . Specific Groat Characteristics and Relation to Groat Damage during Impact Dehulling, Cereal Chemistry , 79 ( 6 ) : 790 – 797 .
- Omobuwajo , T.O. , Ikegwuoha , H.C. , Koya , O.A. and Ige , M.T. 1999 . Design, Construction and Testing of a Dehuller for African Breadfruit (Treculia africana) Seeds . Journal of Food Engineering , 42 : 173 – 176 .
- Suthar , S.H. and Das , S.K. 1996 . Some Physical Properties of Karingda (Citrullus lanatus (Thumb) mansf.) Seeds . Journal of Agricultural Engineering Research , 34 : 77 – 90 .
- Singh , G. 1993 . Development of a Unique Groundnut Decorticator. Agricultural Mechanization in Asia, Africa & Latin America , 24 ( 1 ) : 55 – 59 . 64
- Araullo , E.V. , De Padua , D.B. and Graham , M. 1976 . Rice: Post-Harvest Technology , 207 – 277 . Ottawa, , Canada : International Development Research Centre .
- Subramanian , R. , Sastry , M.C.S. and Venkateshmurthy , K. 1990 . Impact Dehulling of Sunflower Seeds: Effect of Operating Conditions and Seed Characteristics . Journal of Food Engineering , 12 : 83 – 94 .
- Paulsen , G.R. 1978 . Fracture Resistance of Soybeans to Compressive Loading . Transactions of the American Society of Agricultural Engineers , 21 ( 8 ) : 1210 – 1216 .
- Oloso , A.O. and Clarke , B. 1993 . Some Aspects of Strength Properties of Cashew Nut . Journal of Agricultural Engineering Research , 55 ( 1 ) : 27 – 43 .
- Emadi , B. , Kosse , V. and Yarlagadda , K.O.V. 2005 . Mechanical Properties of Pumpkin. International . Journal of Food Properties , 8 ( 2 ) : 277 – 287 .
- Dedio , W. 1993 . Regression Model Relating Decortication of Oilseed Sunflower Hybrids with Achene Characteristics . Canadian Journal of Plant Science , 73 : 825 – 828 .
- Dedio , W. and Dorrell , D.G. 1998 . Factors Affecting the Hullability and Physical Characteristics of Sunflower Achenes . Canadian Institute Food Science Technology Journal , 22 : 143 – 146 .
- Denis , L. and Vear , F. 1996 . Variation of Hullability and other Seed Characteristics Among Sunflower Lines and Hybrids . Euphytica , 87 : 177 – 187 .
- Fick , G.N. and Miller , J.F. 1997 . “ Sunflower Breeding ” . In Sunflower Technology and Production , 2nd , Edited by: Schneiter , A.A. 395 – 439 . Madison, WI : American Society of Agronomy .
- Ramakrishna , P. 1986 . Melon Seeds-Evaluation of Physical Characteristics . Journal of Food Science & Technology , 23 ( 3 ) : 158 – 160 .
- Joshi , D.C. 1993 . Mechanical Dehulling of Melon Seeds , Kharagpur, , India : Unpublished Ph.D Thesis, IIT .
- Gupta , R.K. and Das , S.K. 2000 . Fracture Resistance of Sunflower Seed and Kernel to Compressive Loading . Journal of Food Engineering , 46 : 1 – 8 .
- Aydin , C. 2002 . Physical Properties of Hazelnuts . Biosystems Engineering , 82 : 297 – 303 .
- Singh , K.K. and Goswami , T.K. 1996 . Physical Properties of Cumin Seeds . Journal of Agricultural Engineering Research , 64 : 93 – 98 .
- Vursavus , K. and Ozguven , F. 2005 . Some Physical, Mechanical and Aerodynamic Properties of Pine (Pinus pinea) Nuts . Journal of Food Engineering , 68 : 191 – 196 .
- Ogunsina , B.S. , Koya , O.A. and Adeosun , O.O. 2008 . Deformation and Fracture of Dika Nut (Irvingia gabonensis) under Uni-axial Compressive Loading . International Agrophysics , 22 ( 3 ) : 249 – 253 .
- Pliestic , S. , Dobricevic , N. , Filipovic , D. and Gospodaric , Z. 2006 . Physical Properties of Filbert Nut and Kernel . Biosystems Engineering , 93 ( 2 ) : 173 – 178 .
- Koya , O.A. and Faborode , M.O. 2005 . Mathematical Modeling of Palm Nut Cracking based on Hertz Theory . Biosystems Engineering , 95 ( 3 ) : 405 – 412 .
- Wang , C.H. and Mai , Y.W. 1994 . Deformation and Fracture of Macadamia Nuts—Part2: Microstructure and Fracture Mechanics Analysis of Nutshell . International Journal Fracture , 69 : 67 – 85 .
- Liu , R. , Wang , C.H. and Bathgate , R.G. 1999 . Fracture Analysis of Cracked Macadamia Nutshell under Load between Two Rigid Plates . Journal of Agricultural Engineering Research , 74 : 243 – 250 .
- Association of Official Analytical Chemists (AOAC) . 2000 . Official Methods of Association of Official Analytical Chemists International , 17th , Edited by: Cunnifi , P. Vol. 11 , 1 – 37 . Arlington, VA : AOAC .
- Mohsenin , N.N. 1986 . Physical Properties of Plant and Animals , 2nd , 1 – 28 . New York : Gordon and Breach Science Publishers .
- American Society of Agricultural and Biological Engineers (ASAE). ASAE Standards . 2000 . Compression Test of Food Materials of Convex Shape , St. Joseph, MI : ASAE Standard 368.4; American Society of Agricultural and Biological Engineers .
- Faborode , M.O. and Dirinfo , R. 1994 . R. A Mathematical Model of Cocoa Pod Deformation based on Hertz Theory . International Agrophysics , 8 : 403 – 409 .
- Grotte , M. , Duprat , F. and Loonis , D. 2001 . Mechanical Properties of the Skin and the Flesh of Apples . International Journal of Food Properties , 4 ( 1 ) : 149 – 161 .
- Timoshenko , S.P. and Goodier , J.N. 1970 . Theory of Elasticity , 40 Singapore : McGraw-Hill Book Company .
- Sirisomboon , P. , Kitchaicha , P. , Pholpho , T. and Mahuttanyavanitch , W. 2007 . Physical and Mechanical Properties of Jatropha curcas L . Fruit, Nuts and Kernels. Biosystems Engineering , 97 : 201 – 207 .