Abstract
Retrogradation behavior of waxy and normal corn starch gels stored at 5 and 21°C were investigated for 50 days by differential scanning calorimetry. The extend of retrogradation can be expressed as the ratio of retrogradation and gelatinization enthalpy values and these were 70–78% for normal corn starch and 39–68% for amioca stored at two temperatures. Higher retrogradation rates were observed at 5°C for both waxy and normal corn starch. Kinetic data were evaluated by considering retrogradation as consecutive reactions in series and by Avrami Equation. High R2 values (0.97–0.98) indicated that both models can be used for prediction. The comparison of rate constants obtained by Avrami Equation indicated that both temperature and amylose content of starch affected the recrystallization rate. By the kinetic model based on consecutive reactions in series, it was proven mathematically that the rate limiting step in recrystallization of starch gels is the nucleation.
INTRODUCTION
Amorphous products like starch gels are not at thermodynamic equilibrium and will approach it in time via starch molecular rearrangement and recrystallization. If these processes take place above glass transition temperature, the ageing occurred is called retrogradation. The term retrogradation, in general, is used to describe changes in starch gels during cooling and subsequent storage. Therefore, the retrogradation has a major influence on the quality of starch-based foods, such as acceptability, texture, and shelf-life. Due to these diverse effects and from the economical point of view, the retrogradation has received considerable interest in recent years.[Citation1–4] Starch retrogradation, on the other hand, has received special attention from another point of view. That is the effect of retrogradation on increasing the resistance of starch to digestive enzymes and consequently the reduction of the glycemic index (GI) value.[Citation5–7] Low GI foods have been recommended for the prevention of diseases associated with metabolic disorders.[Citation8,Citation9] Resistant starch is defined as the group of starches not digested by digestive enzymes and consequently transferred into the colon. Among the various types of resistant starches, retrograded starch is known as RS3, which is thermally stable to enable its use in a wide variety of conventional foods.[Citation10]
Retrogradation can be considered as a time and temperature dependent polymer recrystallization process.[Citation1,Citation11] After the pioneering study of McIver et al.,[Citation12] the retrogradation kinetics of starch gels were often analyzed by using the Avrami Equation.[Citation11–19] The effect of storage temperatures on the rate and extent of retrogradation was discussed by using the values of constants obtained from this equation.[Citation13,Citation14,Citation17,Citation20,Citation21] However, in these studies, the theoretical background of the Avrami Equation was overlooked. Additionally, negligible research exists in the literature questioning the applicability of the Avrami Equation on the starch retrogradation.[Citation21] Moreover, in the above-mentioned studies, retrogradation experiments were carried out for short-term durations, such as 2–3 weeks. In addition, not much research exists concerning the effect of amylose content of starch on retrogradation. Therefore, the objectives of this study were: (i) to determine the retrogradation behaviors of two corn starches with different amylose contents (waxy and normal corn starches) at two ambient temperatures (5 and 21°C) for long-term storage (50 days), (ii) to demonstrate and discuss the applicability of Avrami Equation, (iii) to propose a new model equation based on the irreversible reactions in series in order to evaluate the kinetic data, and (iv) to discuss the results from the point of view of classical crystallization theory, temperature, and amylose content.
MATERIALS AND METHODS
Materials
Two kinds of corn starches with different amylose contents were used in this study. Normal corn starch was purchased from Sigma Chemical Co. (St. Louis, MO, USA). Amioca (waxy corn starch) was donated by National Starch & Chemical Limited (Bridgewater, NJ, USA). Amylose contents of starches were approximately 1–2 g/100 g starch for amioca and 21–24 g/100 g starch for normal corn starch, as declared by their producers. Moisture contents of starches were determined using a standard AOAC[Citation22] method and were found to be 11.4 ± 0.1 and 11.2 ± 0.1 (g/100 g starch) for amioca and normal corn, respectively.
Differential Scanning Calorimetry
Differential scanning calorimetry technique was used to follow gelatinization, glass transition, and retrogradation of starch samples. Experiments were carried out using TA Q20 model differential scanning calorimeter (DSC) apparatus (TA Instruments, New Castle, USA) with the cooling system. The calorimeter was calibrated with indium (melting point = 156.6°C, ΔH = 28.5 J/g). The DSC runs were operated under nitrogen gas atmosphere (30 mL/min) and an empty pan was used as the reference. The scanning rate was 10°C/min for all experiments.
The samples were prepared as follows: about 3 mg starch was weighed in an aluminum pan and distilled water was subsequently added. Then the content of the pan was controlled periodically by weighing until the desired moisture content was attained. Afterwards, the pan was sealed hermetically and allowed to stabilize at room temperature overnight before heating in the calorimeter. The weight of samples was determined by using an analytical balance (Gec Avery VA/WA, accuracy = ±0.0001 g). The amount of water added to the starch was adjusted accordingly to obtain the water:starch ratio of 3:1 (w/w). This is the adequate amount of water required for complete gelatinization of both amioca and normal corn starch, as determined experimentally. The original moisture content of the starch sample was also taken into account in calculating the water-starch ratio.
During a DSC run, the pans were heated from room temperature (21 ± 3°C) to 130°C. The thermogram was evaluated to define the gelatinization properties (onset, peak completion temperatures, and the gelatinization enthalpy). In order to determine the glass transition temperatures of both hydrated and gelatinized starches, the hydrated sample prepared as described above was first cooled down −50°C, maintained at that temperature for 30 min, and then heated to 90°C. Then a second run was conducted with the same sample (the one gelatinized during the first run). The sample procedure mentioned above was followed also with this sample. In this way, the glass transition temperatures () of both hydrated and gelatinized starches were determined by taking the first derivative of the thermogram representing the glass transition.
Retrogradation Experiments
Each sample pan to be used in the retrogradation experiment contained 75% moisture by weight and was prepared according to the procedure mentioned in the previous section. The gelatinization of starch was accomplished (first scan) and controlled (second scan) by DSC. Then the gelatinized sample pans were stored at room temperature (21 ± 3°C) and in a refrigerator (5 ± 2°C) for 50 days. At certain time intervals (5, 10, 15, 20, 25, 30, 40, and 50 days of storage), one sample pan was removed from the batch and rescanned for retrogradation analysis (third scan). For pans stored at 21°C, the scans were conducted immediately, while for those stored at 5°C, the scans were conducted after maintaining at room temperature for 30 min. For each storage time and temperature, two independent replicates were used. The endothermic peak obtained in the second scan was analyzed quantitatively. The onset (To ), peak (Tp ), and completion (Tc ) temperatures were measured from the thermogram. The enthalpy associated with retrogradation (ΔHr ) was measured and expressed as J/g dry starch. The data collected by DSC experiments were analyzed by the instrument's software.
Statistical Analysis
Experimental data were subjected to one-way analysis of variance (ANOVA), using SPSS version 11.5 (SPSS Inc., Westchester, NY, USA). Treatment means were tested separately for the least significant difference test. Nonlinear curve fitting was performed by using Origin 7.0 software (OriginLab, Northampton, MA, USA).
RESULTS AND DISCUSSION
Glass Transition Temperatures
As stated before, ageing of starch called retrogradation occurs above Tg
. In order to observe retrogradation, Tg
s of hydrated and gelatinized starches were determined experimentally, following the procedure discussed in the Materials and Methods section, and the storage temperatures were selected accordingly. When the sample is cooled, ice formation often causes the solid concentration to increase in unfrozen water. Therefore, the transition preceeding apparent (initial point of ice melting) corresponds to the glass transition of solid matrix (
) (mixture of solid and unfreezable water) in the frozen sample. This temperature is named as apparent
.[Citation23,Citation24] The
s of hydrated amioca and normal corn starch were determined as −0.8 and −10.1°C, respectively.
s were found to be −4.5 and −5.4°C for their gelatinized counterparts ( and ).
Figure 1 DSC thermograms for glass transition temperatures of (a) amioca and (b) normal corn starch in hydrated and gelatinized forms (dashed lines represent the first derivative of heat flow).
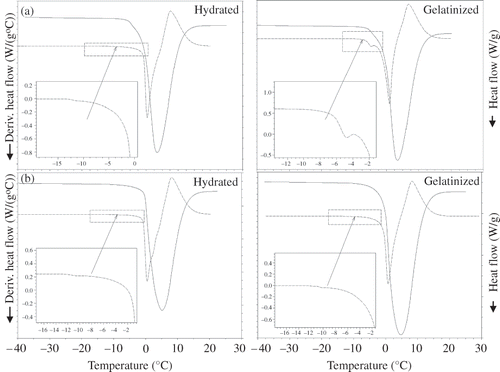
Table 1 Apparent glass transition temperatures (
) and apparent initial point of ice melting (
) for hydrated and gelatinized amioca and normal corn starch
It is obvious that was higher in gelatinized starches. The difference between
s of hydrated and gelatinized starches may be attributed to the plasticizing effect of water. It is known that plasticization by water results in a depression of Tg
in a completely amorphous and partially crystalline polymer. At this point, the water binding in hydrated and gelatinized starches should be taken into account because the water binding in those matrices are different. The hydration of starch granules involves the reversible adsorption of small amounts of water into amorphous regions of starch. But gelatinized starch holds moisture as an integral part of solid structure via hydrogen bonding and, moreover, a greater proportion of amorphous chains in the gelatinized starch interact with water. Therefore, it was concluded that the structural differences outcoming by gelatinization resulted in a decrease in available water content of gelatinized starch, as a result of which its plasticizing effect was decreased and higher temperatures were required for glass transition.
In the literature, glass transition temperature for fully water-plasticized wheat starch gel has been shown to be −5°C by Slade and Levine.[Citation11] Therefore, the results obtained in this research were in accordance with those noted in the literature (). Chung et al.[Citation25] reported that not much data existed for glass transition temperature of hydrated native starches, and their values obtained for hydrated rice starch were in the range of −6.8 and −6°C for moisture contents of 40 and 60%. A comparison of glass transition temperatures of hydrated amioca and normal corn starch with those in literature showed that the values obtained in this research were lower than those obtained in the literature. This difference may be attributed to the types of starch as well as the plasticizing effect of water, since the samples used in this research contained 75% moisture by weight.
Retrogradation at Different Storage Temperatures
The retrogradation is due to molecular interactions between starch chains via hydrogen bonding. The process has been described as a non-equilibrium polymer recrystallization process in a completely amorphous (in the case of waxy starches) starch-water melts. In normal starches, retrogradation consists of two separable processes involving amylose and amylopectin. These are the fast gelation of amylose solubilized during gelatinization, and slow recrystallization of amylopectin within the gelatinized granules. While the amylose crystallization is dominant at the initial stages of retrogradation, long term changes in starch gels are generally due to amylopectin.[Citation26]
On DSC thermograms, retrogradation of starch gels can be followed by the characteristic melting endotherm of recrystallized amylopectin. This endotherm is absent in fresh gels immediately after gelatinization and appears at around 40–70°C on aging. illustrates the DSC thermograms of starch gels studied at different storage temperatures. A typical endotherm for the melting of retrograded amylopectin was observed for amioca at 40–72°C and for normal corn starch at 40–71°C ( and ). These values are in accordance with the results reported in the literature.[Citation27–29]
Figure 2 DSC thermograms of (a) amioca and (b) normal corn starch and their gels stored at stored at 5 and 21°C (Storage time = 25 days. For amioca gel stored at 21°C, storage time = 50 days).
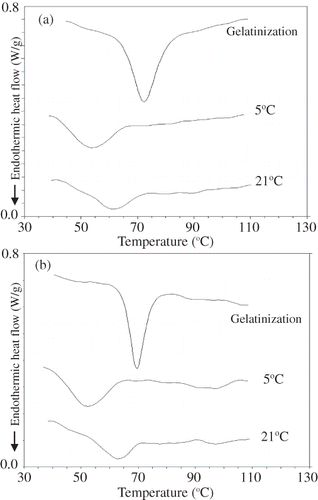
The retrogradation enthalpy versus time data at 5 and 21°C were presented for amioca in and for normal corn starch in . The enthalpy versus time curves of amioca and normal corn starch at 5°C were similar, showing that the retrogradation rate was high at the initial stages of retrogradation, and then the enthalpy values reached to a plateau value as the time elapsed. The retrogradation curve of amioca increased tremendously up to the 10 days, reaching to the enthalpy value of 9.1 ± 0.2 J/g dry starch and after the 15 days of storage, they fluctuated about a mean. The average value representing this second period (15 to 50 days) was found to be 10.7 ± 0.2 J/g dry starch. The retrogradation curve of normal corn starch increased in a similar manner in the first 5 days, reaching up to an enthalpy value of 6.1 ± 0.6 J/g dry starch. Then up to the 20th day, it increased steadily and from that point on, values again fluctuated about a mean. This mean value (20 to 50 days) was found to be 9.8 ± 0.5 J/g dry starch. For storage at 21°C, a completely different behavior was noted in either amioca and normal corn starch ( and ). In amioca gels, for example, no detectable retrogradation occurred during 25 days and an enthalpy value of 6.1 J/g dry starch was observed at the end of the storage. In normal corn starch, retrogradation proceeded slowly, reaching an enthalpy value of 8.7 J/g dry starch on the 50th day.
Figure 3 Retogradation enthalpy values of (a) amioca and (b) normal corn strach gels as a function of time at different storage temperatures.
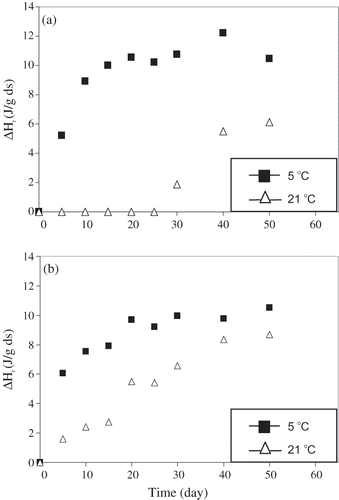
A similar study was carried out by Jane et al.[Citation27] at a temperature of 4°C, using normal and waxy corn starches. The enthalpy values reported were lower than those obtained in this research. This was attributed to the short-time retrogradation experiments, which were only 7 days. Long-duration retrogradation (30 days) was investigated by Nakazawa et al.[Citation30] using normal potato starch at 5 and 23°C. The values reported by these authors at the end of 30 days were 10.8 J/g at 5°C and 9.3 J/g at 23°C. The results obtained for normal corn starch in this research were in accordance with those noted in the literature.[Citation30]
and showed that retrograded starch gels had very different characteristics from their normal counterparts. For example, the retrogradation endotherm was broader than the gelatinization one ( and ). The gelatinization peak temperature for amioca was determined as 72.1 ± 0.4°C and for normal corn starch as 69.4 ± 0.2°C. The gelatinization enthalpies (ΔHg ) were 15.7 J/g dry starch for amioca and 12.5 J/g dry starch for normal corn starch. The endotherm parameters for retrograded starches were presented in and their enthalpy values were given in and . A comparison of gelatinization and retrogradation enthalpy values indicated that retrogradation enthalpies dropped significantly. When the comparison was based on the average values representing the fluctuation period at 5°C and enthalpy values at the 50th day at 21°C. The retrogradation enthalpies for amioca gel stored at different temperatures were determined to be 39–68% smaller than its gelatinization enthalpy. The same values for normal corn starch were 70–78% smaller. The lower extent of decrease (39%) was observed in amioca gels at 21°C. This was due to fact that in this sample the retrogradation was observed after 25 days of storage. The retrogradation enthalpies are reported to be 60–80% smaller than gelatinization enthalpies.[Citation31] Except amioca gels at 21°C, our values were in accordance with what has been stated in the literature. The decrease in peak temperature was calculated as the difference between the gelatinization and retrogradation peak temperatures. The decrease in peak temperatures were 11–20°C for amioca and 10–18°C for normal corn starch, depending on storage temperature. The reported values are 16–26°C.[Citation31] Therefore, our values also fell within the ranges stated in the literature.
Table 2 Effect of storage temperature on retrogradation endotherm parameters
That the retrograded starches showed lower enthalpy and transition temperatures than their normal counterparts is an indication of their weaker crystallinity and difference in their crystalline forms from those present in their normal granules. This was in agreement with the observations of Chang and Liu[Citation32] for rice starch and was also consistent with the results of the X-ray diffraction (XRD) studies.[Citation33] The extent of decrease in enthalpy was higher in normal corn starch gels than in amioca. A greater amount of amylose has been linked to a greater retrogradation tendency in starches.[Citation34] From this point of view, our results were in good accordance with those stated in the literature.
The thermal characteristics of retrogradation endotherm were also affected by storage temperature (). The onset (To ), peak (Tp ), and conclusion (Tc ) temperatures significantly increased as the storage temperature increased (P < 0.05) (). The dependency of thermal characteristics on storage temperature raised the questions concerning that different crystal types were being formed in gels stored at different temperatures. But according to X-ray diffraction study of corn starch gels, Jouppila et al.[Citation20] reported that the crystal type produced during recrystallization was independent of storage temperature. They also indicated that the peaks in XRD patterns were broader and flatter at lower storage temperatures, suggesting that smaller and/or less perfect crystalline regions were formed at low temperatures. This fact was also supported by the study carried out by Zeleznak and Hoseney[Citation35] in which the researchers investigated and compared the retrogradation endotherms and XRD patterns of bread stored at different temperatures. According to our experimental results, as the storage temperature decreased, the endotherm shifted towards to lower temperatures, and this fact indicated that smaller and/or less perfect crystals were formed at lower temperatures. On the other hand, no significant difference was noted between the thermal characteristics of retrogradation endotherms of amioca and normal corn gels stored at the same temperature (P < 0.05).
Modeling of Retrogradation
Retrogradation considered as consecutive reactions in series
The model that has received the greatest application to eludiciate the starch retrogradation is the Avrami Equation. As reported in the literature, the Avrami Equation was originally developed to describe the crystallization kinetics of synthetic polymers.[Citation20,Citation36] But this equation seems to be lacking because it does not include the basic steps of crystallization. According to the classical polymer crystallization theory, crystallization proceeds via a three step mechanism: nucleation → propagation → maturation. Nucleation is the first step of crystallization and requires nucleus formation. The propagation and maturation steps stand for crystal growth. The basic requirement for crystallization is that the nucleus formation must first occur before crystal growth starts. New nuclei may continue to form while the nuclei present are growing.
This mechanism may very likely be considered to irreversible reactions in series. Assuming that the crystallization is considered as consecutive unimolecular type first-order reactions, the following scheme can be written for the recrystallization of starch:
If the rate equations for three components are written and the differential equation obtained is solved by using the stoichiometric relations between the concentrations of reacting components, the following equation was obtained (the solution of the differential equation was presented by Levenspiel[Citation37]):
The equation shows the ratio of retrogradation enthalpy (ΔHr ) to gelatinization enthaply (ΔHg ). The gelatinization enthaply for each starch at the experimental conditions has a constant value and was determined by DSC in the scope of this research. As stated before, it has the value of 15.7 J/g dry starch for amioca, and for normal corn starch it is equal to 12.5 J/g dry starch. The right side of the equation contains two constants, k 1 and k 2. These are the first order constants for the first and second reactions and have the units of day−1. The applicability of EquationEq. (1) was studied analyzing the experimental data (ΔHr /ΔHg versus t) by nonlinear regression program (Origin 7.0). indicated that no retrogradation up to the 30th day was observed in amioca samples stored at 21°C. In this case, in the evaluation of experimental data, this lag time was taken into consideration and the experimental points obtained at t < 25 days were not taken into evaluation. Only ΔHr values at t ≥ 25 days were evaluated by assigning the 25th day as the start of retrogradation (t = 0) and following days accordingly. The same procedure was followed in the modeling of data by the Avrami Equation. The k 1 and k 2 values thus obtained for each starch at the different temperatures were presented in . Rate constants in this table indicated that k 2 >> k 1, in other words, the overall rate of recrystallization was determined by k 1 or the first step of the two-step reaction. For processes in series, it is the slowest step that has the greatest influence on the overall reaction. Therefore, it was proven mathematically that the formation of nuclei is the process that controls the overall rate of retrogradation.
Table 3 Kinetic parameters for corn starch gels
Avrami Equation
In the literature, starch retrogradation data are generally analyzed by means of the Avrami Equation.[Citation12,Citation13,Citation19,Citation20,Citation36,Citation38–42] The general form of the Avrami Equation is expressed as follows:
There are two important parameters in EquationEq. (3): the rate constant (k) and the Avrami exponent (n). The rate constant depends on the crystal nucleation and growth rate.[Citation36] The Avrami exponent depends on two factors: type of nucleation (homogeneous and heterogeneous) and the dimensions in which growth takes place. If the crystal growth is in one dimension: rod-like crystals are formed; two dimensions: disc-like crystals are formed; three dimensions: spherulitic crystals are formed.[Citation12,Citation36,Citation43] When the Avrami Equation is applied to the case of starch retrogradation, in some studies Avrami exponent is assumed as 1 (heterogeneous nucleation and one dimensional crystal growth).[12,13,36,40,41] Then EquationEq. (3) becomes:
Referring back to EquationEq. (1) and if k 2 >> k 1, then the equation reduces to:
This equation is identical to the Avrami Equation with n = 1. In the analysis of experimental data by the Avrami Equation, the usual practice is to use ΔH ∞ instead of ΔHg . EquationEq. (4) indicates that ΔHr varies exponentially with time and reaches to a constant value of ΔH ∞ as t → ∞. Theoretically, ΔH ∞ values obtained by modeling should have the value of ΔHg at most, since starch gels approach thermodynamic equilibrium in time via starch recrystallization. Equilibrium implies a situation in which there is no change in the properties of that material with time and the rate of change becomes very slow as the equilibrium is approached. Actually, equilibrium is assumed in scientific studies when changes can no longer be detected by the available methods. At this point, mathematical modeling of experimental data becomes a useful tool to estimate the property as t → ∞.
The applicability of EquationEq. (4) was studied by a nonlinear regression program and k and ΔH ∞ values obtained were presented in . Low R 2 values ranging from 0.88 to 0.98 indicated that Avrami Equation with n = 1 is not a good fit for the mathemathical modeling of experimental data. As a third step in the modeling, the general form of Avrami Equation was used. Three unknown parameters (ΔH ∞, k, n) in EquationEq. (2) were estimated by the nonlinear regression analysis program (). The fit of the models was assessed by correlation coefficient (R 2). Values obtained ranging from 0.97–0.99 showed that the general form of the Avrami Equation gave a reasonable description of starch retrogradation at different temperatures.
Dependency of Crystallization Rate on Temperature
The rate constant (k) decreased as the storage temperature increased (). This relationship between rate and temperature can be interpreted in terms of polymer crystallization theory.[Citation35] As stated before, the crystallization process proceeds via a three-step mechanism: nucleation → propagation → maturation. Total rate of crystallization depends on both rates of nucleation and propagation steps. But in this process, the rate-limiting step is nucleation (which is enhanced at lower temperatures) rather than propagation (which is enhanced at higher temperatures). Therefore, in starch gels, higher nucleation and lower propagation rates would be expected at 5°C, compared to those at 21°C. This was evidenced by substantially higher ΔHr values at the beginning of storage at 5°C for both amioca and normal corn starch. But the enthalpy values of normal corn starch gels stored at 5 and 21°C were found to be converging after 50 days of storage because of higher propagation rate at 21°C. Also, the lack of retrogradation endotherm in amioca during 25 days of storage at room temperature, in contrast to normal corn starch, should not be overlooked. This could be attributed to the contribution of amylose fraction to the nucleation step in normal corn starch. Temperature affects both the formation of the nuclei and propagation steps. From the well-known thermodynamic relationship,
as ΔG < 0 (recrystallization is a spontaneous process) and ΔS < 0 (the crystalline form has less freedom), then ΔH < 0, where ΔG, ΔH, and ΔS are changes in free energy, enthalpy, and entropy, respectively. Therefore, an increase of temperature does not favor crystallization. In our experiments, retrogradation data for amioca and normal corn starch at 5 and 21°C showed the similar trend ( and ).
While discussing the temperature dependency of the retrogradation rate, the rate constants obtained by use of the general form of Avrami Equation were taken into consideration. A comparison of rate constants in indicated that both temperature and amylose content of starch affected the crystallization rate. But the effect of temperature was more evident. Since the decrease in temperature resulted in a nearly 20-fold increase in rate constants for both starches while the increase in amylose content created an ∼3-fold increase. The temperature dependency of the rate constant can be given by the following exponential function:
where T is the temperature, K and B is a constant with unit of K −1. The value of B shows the sensitivity of k against the temperature. The greater the value of B, the more sensitivity of k to the temperature. Using k values available at two different temperatures, B values were determined for amioca and normal corn starch. Very close values (B = 0.186 K −1 for amioca and B = 0.191 K −1 for normal corn starch) were obtained. The closeness of B values suggests that the temperature affects the retrogradation ability of amioca or normal corn starch to the same extent. Here, it should be kept in mind that k value for retrogradation of amioca at 21°C has been calculated after retrogradation has started (t ≥ 25 days).
CONCLUSIONS
The following conclusions were derived from this study: (i) The retrograded starches showed lower enthalpy and transition temperatures than their native counterparts. This can be taken as an indication of their weaker crystallinity and difference in their crystalline forms from those present in their native granules. Also, as the storage temperature is decreased, endotherm shifted to lower temperatures, indicated that smaller and/or less perfect crystals are formed at lower temperatures. (ii) In the modeling of retrogradation data by considering crystallization process as consecutive reactions in series, high k 2 values having the order of magnitude of 1013 day−1 were obtained. This proves mathematically that the formation of nuclei is the process that controls the overall rate of crystallization. (iii) High R 2 values obtained in modeling of experimental data by considering the process as consecutive reactions in series or by using the general form of the Avrami Equation suggest that these equations can be applicable for modeling the starch retrogradation. (iv) Both temperature and amylose content of starch gels were effective in the rate and extent of retrogradation.
ACKNOWLEDGMENT
The authors are thankful to the Scientific and Technical Research Council of Turkey for providing funds in the form of a research project (Project number: TOGTAG-3181).
REFERENCES
- Biliaderis , C.G. 1990 . “ Thermal analysis of food carbohydrates ” . In Thermal Analysis of Foods , Edited by: Harwalkar , V.R. and Ma , C.Y. 168 – 220 . New York : Elsevier Applied Science Publishers .
- Eliasson , A. C. and Tatham , A. 2001 . “ Cereal starches and proteins ” . In Cereal and Cereal Products Chemistry and Technology , Edited by: Dendy , D.A.V. and Dobraszczyk , B.J. 68 – 89 . Gaithersburg, Maryland : Aspen Publishers Inc .
- Gudmundsson , M. 1994 . Retrogradation of starch and role of its components . Thermochimica Acta , 246 : 329 – 341 .
- Karim , A.A. , Norziah , M.H. and Seow , C.C. 2000 . Methods for the study of starch retrogradation . Food Chemistry , 71 : 9 – 39 .
- Eerlingen , R.C. , Jacobs , H. and Delcour , J.A. 1994 . Enzyme-resistant starch. V. Effect of retrogradation of waxy maize starch on enzyme susceptibility . Cereal Chemistry , 71 : 351 – 355 .
- Fredriksson , H. , Bjorck , I. , Andersson , R. , Liljeberg , H. , Silverio , J. , Elliasson , A.C. and Aman , P. 2000 . Studies on α-amylase degradation of retrograded starch gels from waxy maize and high-amylopectin potato . Carbohydrate Polymers , 43 : 81 – 87 .
- Frei , M. , Siddhuraju , P. and Becker , K. 2003 . Studies on the in vivo starch digestibility and the glycemic index of six different indigenous rice cultivars from the Philippines . Food Chemistry , 3 : 395 – 402 .
- Jenkins , D.J. , Wolever , T.M. and Taylor , R.H. 1981 . Glycemic index of foods: A physiological basis for carbohydrate exchange . American Journal of Clinical Nutrition , 34 : 362 – 366 .
- Ludwig , D.S. 2000 . Dietary glycemic index and obesity . Journal of Nutrition , 130 : 280 – 283 .
- Haralampu , S.G. 2000 . Resistant starch: A review of the physical properties and biological impact of RS3 . Carbohydrate Polymers , 41 : 285 – 292 .
- Slade , L. and Levine , H. 1991 . Beyond water activity: Recent advences based on an altenative approach to the assessment of food quality and safety . Critical Reviews in Food Science and Nutrition , 30 ( 2–3 ) : 115 – 360 .
- McIver , R.G. , Axford , D.W.E. , Colwell , K.H. and Elton , G.A.H. 1968 . Kinetic study of the retrogradation of gelatinized starch . Journal of the Science of Food and Agriculture , 19 : 560 – 563 .
- Colwell , K.H. , Axford , D.W.E. , Chambelain , N. and Elton , G.A.H. 1969 . Effect of storage temperature on the ageing of concentrated wheat starch gels . Journal of the Science of Food and Agriculture , 20 : 550 – 555 .
- Longton , J. and Legrys , G.A. 1981 . Differential scanning calorimetry studies on the crystallinity of aging wheat-starch gels . Stärke , 33 ( 12 ) : 410 – 414 .
- Eliasson , A.C. 1983 . Differential scanning calorimetry studies on wheat-starch gluten mixtures. 2. Effect of gluten and sodium stearoyl lactylate on starch crystallization during aging of wheat-starch gels . Journal of Cereal Science , 1 ( 3 ) : 207 – 213 .
- Germani , R. , Ciacco , C.F. and Rodriguez-Amaya , D.B. 1983 . Effect of sugars, lipids and type of starch on the mode and kinetics of retrogradation of concentrated corn starch gels . Starch/Stärke , 35 : 377 – 381 .
- del Rosario , R.R. and Pontiveros , C.R. 1983 . Retrogradation of some starch mixtures . Starch/Starke , 35 : 86 – 92 .
- Bulkin , B.J. , Kwak , Y. and Dea , I.C.M. 1987 . Retrogradation kinetics of waxy-corn and potato starches: A rapid, Raman-spectroscopic study . Carbohydrate Research , 160 : 95 – 112 .
- Russell , P.L. 1987 . The ageing of gels from starches of different amylose/amylopectin content studied by differential scanning calorimetry . Journal of Cereal Science , 6 : 147 – 158 .
- Jouppila , K. , Kansikas , J. and Roos , Y.H. 1998 . Factors affecting crystallization kinetics in amorphous corn starch . Carbohydrate Polymers , 36 : 143 – 149 .
- Marsh , R.D.L. and Blanshard , J.M.V. 1988 . The application of polymer crystal growth theory to the kinetics of formation of the N-amylose polymorph in a 50% wheat-starch gel . Carbohydrate Polymers , 9 : 301 – 317 .
- AOAC . 1990 . Official Methods of Analysis of the Association of Official Analytical Chemists, Inc , Virginia : AOAC .
- Rahman , M.S. , Sablani , S.S. , Al-Habsi , N. , Al-Maskri , S. and Al-Belushi , R. 2005 . State diagram of freeze-dried garlic powder by differential scanning calorimetry and cooling curve methods . Journal of Food Science , 70 ( 2 ) : E135 – E141 .
- Rahman , M.S. 2004 . State diagram of date flesh using differential scanning calorimetry (DSC) . International Journal of Food Properties , 7 ( 3 ) : 407 – 428 .
- Chung , H-J. , Lee , E-J. and Lim , S-T. 2002 . Comparison in glass transition and enthalpy relaxation between native and gelatinized rice starch . Carbohydrate Polymers , 48 : 287 – 298 .
- Sathaporn , S. and Jane , J.L. 2007 . Physicochemical properties of starch affected by molecular composition and structures: A review . Food Science and Biotechnology , 16 : 663 – 674 .
- Jane , J. , Chen , Y.Y. , Lee , L.F. , McPherson , A.E. , Wong , K.S. , Radosavljevic , M. and Kasemsuwan , T. 1999 . Effects of amylopectin branch chain length and amylose content on the gelatinization and pasting properties of starch . Cereal Chemistry , 76 : 629 – 637 .
- Farahnaky , A. and Majzoobi , M. 2008 . Physicochemical properties of part baked breads . International Journal of Food Properties , 11 : 186 – 195 .
- Thirathumthavorn , D. and Trisuth , T. 2008 . Gelatinization and retrogradation properties of native and hydroxypropylated crosslinked tapioca starches with added sucrose and sodium chloride . International Journal of Food Properties , 11 : 858 – 864 .
- Nakazawa , F. , Noguchi , S. , Takahashi , J. and Takada , M. 1985 . Retrogradation of gelatinized potato starch studied by differential scanning calorimetry . Agricultural Biology and Chemistry , 49 ( 4 ) : 953 – 957 .
- Singh , N. , Singh , J. , Kaur , L. , Soghi , N.S. and Gill , B.S. 2003 . Morphological, thermal and rheological properties of starches from different botanical sources . Food Chemistry , 81 : 219 – 231 .
- Chang , S.M. and Liu , L.C. 1991 . Retrogradation of rice starches studied by differential scanning calorimetry and influence of sugars, NaCl and lipids . Journal of Food Science , 56 ( 2 ) : 564 – 570 .
- Kim , J.O. , Kim , W.S. and Shin , M.S. 1997 . A comparative study on retrogradation of rice starch gels by DSC, X-ray and α-amylase methods . Starch/Stärke , 49 ( 2 ) : 71 – 75 .
- Whistler , R.L. and BeMiller , J.N. 1996 . “ Starch ” . In Carbohydrate Chemistry for Food Scientists , Edited by: Whistler , R.L. and BeMiller , J.N. 117 – 151 . St. Paul, MN : Eagan Press .
- Zeleznak , K.J. and Hoseney , R.C. 1987 . Characterization of starch from bread aged at different temperatures . Starch/Stärke , 39 ( 7 ) : 231 – 233 .
- Nobile , M.A.D. , Martoriello , T. , Mocci , G. and Notte , E.L. 2003 . Modeling the starch retrogradation kinetic of durum wheat bread . Journal of Food Engineering , 59 : 123 – 128 .
- Levenspiel , O. 1999 . Chemical Reaction Engineering , 3rd , 668 New York : John Wiley and Sons Inc .
- Fearn , T. and Russell , P.L. 1982 . A kinetic study of bread staling by differential scanning calorimetry. The effect of loaf specific volume . Journal of Science and Food Agriculture , 33 : 537 – 548 .
- Russell , P.L. 1989 . The ageing of gels from starches of different amylose/amylopectin content studied by differential scanning calorimetry . Journal of Cereal Science , 6 : 147 – 158 .
- Roulet , P. , MacInnes , W.M. , Gumy , D. and Würsch , P. 1990 . Retrogradation kinetics of eight starches . Starch/Stärke , 42 ( 3 ) : 99 – 101 .
- Baker , L.A. and Duarte , P.R. 1998 . Retrogradation of amaranth starch at different storage temperatures and effect of salt and sugars . Cereal Chemistry , 75 ( 3 ) : 308 – 314 .
- Farhat , I.A. , Blanshard , J.M.V. and Mitchell , J.R. 2000 . The retrogradation of waxy maize starch extrudates: Effects of storage temperature and water content . Biopolymers , 53 : 411 – 422 .
- Stein , R.S. and Tobolsky , A.V. 1971 . “ Crystallinity in polymers ” . In Polymer Science and Materials , Edited by: Tobolsky , A.V. and Mark , H.F. 161 – 187 . New York, USA : John Wiley & Sons Inc .