Abstract
One fraction enriched with flavonols and phenolic acids; and the fraction enriched with anthocyanins, were isolated from red raspberry, blackberry, sour cherry, strawberry, chokeberry, elderberry, and blueberry to study their antiradical activity using the free radical, 2,2-diphenyl-1-picrylhydrazyl, test. The phenol content was determined using high-performance liquid chromatography. The antiradical activity of anthocyanins was 7 to 25 times higher than the antiradical activity of flavonols and phenolic acids. High correlations were found between the content of anthocyanins, flavonols, phenolic acids, quercetin derivatives, caffeic acid derivatives, and the antiradical activity (r = 0.99, 0.93, 0.83, 0.93, 0.94, respectively). Among the phenols studied in this work, anthocyanins showed the highest influence on the antiradical activity in all fruits. Other phenolics with relatively strong influence on the antiradical activity of fruits were quercetin derivatives and caffeic acid derivatives (chlorogenic acid) in chokeberries and blueberries, quercetin derivatives in blackberries, quercetin derivatives and caffeic acid derivatives (chlorogenic acid) in elderberries, and hydroxycinnamic acids in sour cherries.
INTRODUCTION
A wide number of studies have shown that fruits have protective effects on human health. This especially refers to small fruits and berries. Fruits inhibit the proliferation of some human carcinoma cells in vitro[Citation1,Citation2] and the intake of fruits and fruit juices improves the antioxidant status in humans.[Citation3] Components of fruits with positive effects are various antioxidants like vitamin C or different phenolic compounds.[Citation1] Phenolic compounds are a large group of secondary metabolites that are widespread in various plants.[Citation4–6] Phenols were reported to exhibit anti-inflammatory, anti-carcinogenic, anti-arthritic activities in vitro,[Citation7,Citation8] and may even have a positive role in decreasing the risk of some chronic diseases, such as cancer.[Citation9] One of the reasons for such positive effects of phenols is their ability to scavenge free radicals (antiradical activity).[Citation10] The effects of plant phenols in vivo are not yet completely explained. Phenols are absorbed in the human body, although rarely completely. Some of them are metabolized and products of metabolism have different effects in vivo. These effects are not yet completely understood.[Citation10] Besides, plant phenols are used in the food industry to improve the quality and safety of foods.[Citation11] Because of all these reasons, the interest in studying the plant phenols is still growing.
Fruits contain a mixture of various phenols. Anthocyanidins and flavonols occur as glycosides, flavan-3-ols as monomers and as structural units in proanthocyanidin chains.[Citation12–15] Soluble hydroxycinnamic acids are found as glycosides and as esters with sugars or quinic acid. Ellagic acid is found free in methoxylated and glycosylated forms and as part of unknown polymers.[Citation12,Citation13] These compounds all contribute to some extent to the total antiradical activity of fruits. An earlier study[Citation16] showed that anthocyanins and caffeic acid derivatives have a significant portion in the antiradical activity of chokeberries and blueberries. But, still little information exists about the real contribution of individual phenols or phenolic classes to the total antiradical activity of many other berries and fruits. Since fruits and their phenols showed positive effects, it is important to find phenolic compounds or classes of phenols with the highest influence on the antiradical activity of fruits.
The aim of this study was to investigate the influence of various classes of phenols on the antiradical activity of seven red fruits: chokeberry, elderberry, blueberry, strawberry, blackberry, red raspberry, and sour cherry. Phenols were fractionated into two fractions. The first fraction was enriched with flavonols and phenolic acids and the other with anthocyanins. The radical scavenging activity of phenolic fractions was studied using the 2,2-diphenyl-1-picrylhydrazyl (DPPH) test. The phenol content in fractions was determined by high-performance liquid chromatography (HPLC) analysis. Additionally, the relationship between the phenol content and the antiradical activity was investigated by using the correlation analysis.
MATERIALS AND METHODS
Fruit Samples
Seven species of berries and fruits were harvested at maturity in 2007 [red raspberry (Rubus idaeus), blackberry (Rubus fruticosus), sour cherry (Prunus cerasus), strawberry (Fragaria anannassa), chokeberry (Aronia melanocarpa), elderberry (Sambucus nigra), and blueberry (Vaccinium myrtillus)]. Immediately after harvesting, berries were frozen and stored at −20°C for two months until analyzed for this study.
Chemicals
The anthocyanin standard cyanidin-3-O-glucoside chloride (kuromanin chloride 0915 S) was purchased from Extrasynthese (Genay, France) and prepared in 0.1% methanolic HCl solution in a concentration range of 1–100 mg l−1. Other phenolic standards were obtained from Sigma-Aldrich (St. Louis, MO, USA) (ellagic acid (E2250), (+)-catechin hydrate (C1251), (−)-epicatechin (E1753), chlorogenic acid (C3878), caffeic acid (C0625), ferulic acid (F3500), p-coumaric acid (C9008), quercetin dihydrate (Q0125), kaempferol (K0133), rutin hydrate (quercetin-3-rutinoside hydrate) (R5143)). Calibration curves were made by diluting stock standards in methanol. The 2,2-diphenyl-1-picrylhydrazyl radical (DPPH·) (D9132) and Trolox ((±)-6-hydroxy-2,5,7,8-tetramethylchromane-2-carboxylic acid) (56510) were purchased from Sigma-Aldrich (St. Louis, MO, USA).
Sample Extraction
Two phenol fractions were isolated from fruits: the first fraction with ethyl acetate to extract free and conjugated phenolic acids and flavonol glycosides, and the other with acidified methanol to extract anthocyanins.[Citation12,Citation13] The frozen fruits were homogenized, weighed (3 g) into centrifuge tubes, and were extracted with ethyl acetate (4 × 5 ml) by repeated vortexing. The ethyl acetate extracts were combined; one portion (10 ml) was evaporated to dryness (35°C, rotary evaporator) and dissolved in 2 ml of methanol (first phenol fraction). The fraction was subjected to direct antiradical activity determination using the DPPH test, and afterwards was directly injected into the HPLC system. Since some phenolic compounds from the first fraction were tentatively identified, this fraction was acidified to 0.6 M with concentrated HCl and heated for 5 min in a boiling water bath (70–80°C) to release the aglycones from flavonol and phenolic acid glycosides (hydrolyzed fraction). The hydrolyzed fraction was analyzed on the HPLC system to confirm the phenolic compound identification and to quantify flavonols in the aglycon forms.
The HPLC analysis of the ethyl acetate extract (first fraction) revealed a small amount of free monomeric flavan-3-ols extracted within ethyl acetate.[Citation12,Citation13] The flavan-3-ols in ethyl acetate extracts coeluted with the peaks of hydroxycinnamic acids. Therefore, liquid-liquid extraction of the second part of the ethyl acetate extract was applied to eliminate phenolic acids from extract before the analysis of flavan-3-ols. The second part of ethyl acetate extract (5 ml) was extracted with sodium acetate buffer (2 × 5 ml, 0.1 M, pH 7.0) and with 5 ml of water to remove phenolic acids into the water phase. This purified ethyl acetate phase was then evaporated to dryness, dissolved in 1 ml of methanol, and was used for the determination of flavan-3-ols ((+)-catechin and (−)-epicatechin) by HPLC.
After the ethyl acetate extraction, the berry residue was acidified with HCl (2 M, 1 ml) and extracted with 5 ml of methanol (4 to 8 times).[Citation12,Citation13] The combined extracts contained anthocyanins in the flavylium cation forms. An aliquot of the methanol extract (10 ml) was evaporated to dryness and dissolved in 2 ml of methanol (second phenol fraction). In this fraction, the antiradical activity of anthocyanins was measured using the DPPH test. The anthocyanin content was determined by HPLC analysis. All extracts were prepared in duplicate and filtered through a 0.45-μm syringe filter before injection into the HPLC system.
Chromatographic Analyses
The chromatographic analyses were conducted on a Varian HPLC system (Palo Alto, CA, USA) consisting of ProStar 230 solvent delivery module, ProStar 330 PDA detector, and OmniSpher C18 column (250 × 4.6 mm inner diameter, 5 μm, Varian, USA) (guard column ChromSep 1 cm × 3 mm, Varian, USA). The quantification was conducted by HPLC methods validated earlier in our laboratory.[Citation17,Citation18] The flavonols, flavan-3-ols, and phenolic acids were separated using 0.1% phosphoric acid as solvent A and 100% HPLC grade methanol as solvent B (elution conditions: 0–30 min from 5% B to 80% B; 30–33 min 80% B; 33–35 min from 80% B to 5% B; flow rate = 0.8 ml min−1; injection volumes 20 μl). Phosphoric acid (0.5%) was used as solvent A and 100% HPLC grade methanol was used as solvent B for separation of anthocyanins (elution conditions: 0–38 min from 3% B to 65% B; from 38–45 min, 65% B; flow rate = 1 ml min−1, injection volumes were 20 μl).
UV-Vis spectra were recorded in a wavelength range from 190–600 nm (the detection wavelength was 280 nm for (+)-catechin and (−)-epicatechin; 320 nm for ellagic, chlorogenic, caffeic, p-coumaric, and ferulic acid; 360 nm for quercetin, kaempferol, quercetin-3-rutinsoide; 520 nm for anthocyanins). The phenol identification was based on the comparison of their retention times and spectral data (190–600 nm) with those of the authentic standards. Additional identification was carried out by spiking the fractions with phenolic standards. The identified compounds were quantified using the calibration curves of the authentic standards. Ellagic acid-, p-coumaric acid-, quercetin-, and kaempferol derivatives were tentatively identified by comparison of their spectra features with those found in the literature.[Citation2,Citation12] The quercetin- and kaempferol derivative contents were determined in hydrolyzed ethyl acetate fractions using quercetin and kaempferol calibration curves. The total anthocyanin content was calculated by plotting the total peak area at 520 nm against the cyanidin-3-glucoside calibration curve. The data presented are mean ± standard deviation (SD) (n = 4).
Antiradical Activity
The antiradical activity of two phenol fractions was measured by using the DPPH test.[Citation19] Five dilutions of phenol fraction were prepared, each containing increasing aliquots of phenol fraction, 200 μl of methanolic DPPH• solution (1 mmol dm−3) and methanol (final volume 3 ml). The dilutions were kept in the dark at room temperature for 15 min. The absorbance (A extract) was read against the prepared blank (200 μl of methanol instead of DPPH• solution) at 517 nm. A DPPH• blank sample was prepared each day (200 μl of 1mmol dm−3 DPPH•, 2.8 ml of methanol) and absorbance (A DPPH) was measured daily. The percent inhibition of DPPH• radical caused by each dilution was calculated using the following formula:
A Trolox standard of final concentration 400 μmol dm−3 was prepared in methanol, assayed under the same condition, and the Trolox calibration curve was used to calculate the antiradical activity of all dilutions of phenol fractions (μmol of Trolox equivalent (TE) per gram of fruits). The final results were expressed in μmol of TE per gram of fruits needed to reduce DPPH· radicals by 50% (EC50).
Statistical Analysis
The data are the results of four measurements expressed as mean value ± SD (standard deviation). The correlations between the phenolic compound content in ethyl acetate extract or in methanol extract and the antiradical activity of the same extract were observed. The correlations were expressed by calculating the correlation coefficient (r) (significant at P ≤ 0.05) (Statistica 7.1 [Statsoft, Tulsa, OK, USA]).
RESULTS AND DISCUSSION
shows the HPLC chromatograms of ethyl acetate fractions with identified phenolic acids and flavonols. All of the dominant compounds were identified by comparison of their spectra with those of standards and the identification agrees with literature data.[Citation12–16,Citation20,Citation21] UV/Vis spectra of ellagic acid derivatives (peak 7′, λmax = 245–249, 341–350 nm) and p-coumaric acid derivatives (peak 4′, λmax = 207–227, 299–304 nm) matched to those found in literature.[Citation2,Citation12] UV/Vis spectra of peaks 8′ and 9′ (peak 8′ λmax = 248–250 nm and 338–342 nm; peak 9′ λmax = 260 nm and 333–337 nm) indicated that these compounds are probably quercetin and kaempferol derivatives, respectively.[Citation2,Citation12] The ethyl acetate fractions were hydrolyzed to confirm the presence of these compounds. Hydrolyzation reactions released aglycons from phenolic glycosides (). After analyzing the hydrolyzed fraction on HPLC ellagic acid-, p-coumaric acid-, quercetin-, and kaempferol derivative existence was confirmed ().
Figure 1 HPLC chromatograms of phenol fractions extracted with ethyl acetate and recorded at 320 nm. Peak identification: 1, neochlorogenic acid; 2, chlorogenic acid; 3, caffeic acid; 4, p-coumaric acid; 4′, p-coumaric acid derivative; 5, ferulic acid; 6, quercetin-3-rutinsoide; 7′, ellagic acid derivative; 8, quercetin; 8′, quercetin derivative; 9′, kaempferol derivative.
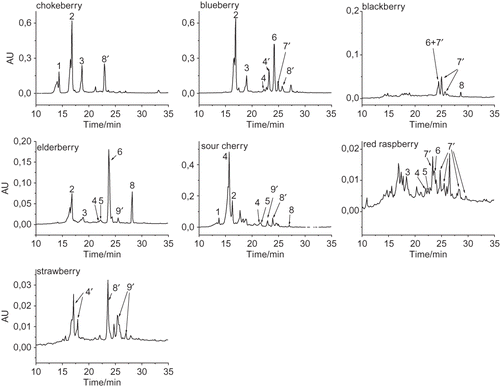
Figure 2 Examples of HPLC chromatograms of hydrolyzed ethyl acetate fractions recorded at 320 and 360 nm. Peak identification: 3, caffeic acid; 4, p-coumaric acid; 7, ellagic acid; 8, quercetin; 9, kaempferol.
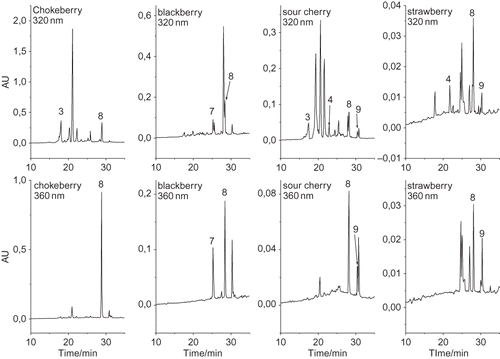
The phenol content is shown in . The dominant phenolic acids were hydroxycinnamic acids (from 3.2 to 267 mg kg−1 FW), and their content was especially high in chokeberries (267 mg kg−1 FW), blueberries (244 mg kg−1 FW), and sour cherries (145 mg kg−1 FW). Earlier studies found higher hydroxycinnamic acid content in chokeberries (892–2617 mg kg−1).[Citation13,Citation16] The hydroxycinnamic acid content in blueberries (163 to 646 mg kg−1)[Citation13,Citation16,Citation22] and in sour cherries (166–336 mg kg−1)[Citation20] is similar to the results found in this work. The dominant hydroxycinnamic acids were caffeic acid derivatives, including neochlorogenic acid, chlorogenic acid, and caffeic acid (chokeberries (267 mg kg−1 FW), blueberries (208 mg kg−1 FW), and sour cherries (66 mg kg−1 FW)). Earlier studies found similar caffeic acid derivative content in blueberries (95–106 mg kg−1;[Citation23] 645 mg kg−1;[Citation16] 111 mg kg−1)[Citation13] and in sour cherries (125–287 mg kg−1)[Citation20] and higher caffeic acid derivative content in chokeberries (832 mg kg−1;[Citation13] 750 mg kg−1;[Citation23] 2,617 mg kg−1).[Citation16] Furthermore, fruits contained relatively high flavonol derivative content (from 6.5 to 438 mg kg−1 FW). Among them quercetin derivatives were the dominant compounds (from 6.5 to 438 mg kg−1 FW). The quercetin derivative content found in the literature (chokeberries 348–576 mg kg−1;[Citation13,Citation16] blueberries 81–249 mg kg−1;[Citation13,Citation16] elderberries 331 mg kg−1;[Citation13] sour cherries 12–48 mg kg−1;[Citation20] red raspberries 1–8 mg kg−1;[Citation12] strawberries 0–11 mg kg−1)[Citation12,Citation21] is similar to the results in this study. A small amount of free monomeric flavan-3-ols were extracted in the ethyl acetate extract as well (). Their content (from 0 to 38 mg kg−1 FW) was lower in comparison to the hydroxycinnamic acid and flavonol content.
Table 1 ContentFootnote a of phenolic acids, flavonols, and flavan-3-ols in first phenol fraction extracted with ethyl acetate, and contentFootnote a of anthocyanins in second phenol fraction extracted with acidified methanol
Methanolic fractions extracted from the berry residue after the extraction of flavonols and phenolic acids, contained anthocyanins (). The anthocyanin content in chokeberries, blueberries, and elderberries (14,733, 13,012, and 10,927 mg kg−1 FW, respectively) was much higher than in other investigated fruit species (from 166 mg kg−1 FW in strawberry to 1,242 mg kg−1 FW in blackberry). The anthocyanin contents are in accordance with literature data (3,167–14,800 mg kg−1 in chokeberries;[Citation14,Citation16] 1,222–8,080 in mg kg−1 in blueberries;[Citation13,Citation16] 2,450 mg kg−1 in blackberries;[Citation14] 3,316–13,750 mg kg−1 in elderberries;[Citation13,Citation14] 0–921 mg kg−1 in red raspberries;[Citation12,Citation14] 78–417 mg kg−1 in strawberries[Citation12,Citation14,Citation21,Citation24,Citation25]). Furthermore, the anthocyanin content in all fruit species was 5 to 53 times higher in comparison to other investigated classes of phenols.
The radical scavenging activity of phenol fractions determined by using the DPPH test is shown in . A higher EC50 value refers to a higher antiradical activity. The antiradical activity of anthocyanins from all fruits (4–26 μmol TE/g) was higher considerably in comparison to the antiradical activity of flavonols and phenolic acids (0.5–2.7 μmol TE/g). This suggested that anthocyanins had much higher portion in the total antiradical activity of investigated fruits in comparison to flavonols and phenolic acids and also a stronger influence on the antiradical activity. The highest difference in the antiradical activity was noticed in elderberry where the antiradical activity of anthocyanins was 25 times higher in comparison to the antiradical activity of flavonols and phenolic acids. The antiradical activity of anthocyanins in other fruits was also considerably higher in comparison to the antiradical activity of flavonols and phenolic acids (15 times in blueberry; 10 times in red raspberry, sour cherry, strawberry, and chokeberry; and 7 times in blackberry). Earlier studies[Citation16,Citation26] also reported a high portion of anthocyanins in the antioxidant activity of fruits. Zheng et al.[Citation16] found that anthocyanins contributed more than 50% to the antioxidant activity of blueberries and chokeberries.
Table 2 Antioxidant activity of phenolic fractions expressed as μmol Trolox equivalent/g of fruit needed to reduce DPPH• radical by 50% in 15 min (EC50) and ratio of EC50 values
The correlations between phenolic compounds and the antiradical activity are shown in . A strong correlation existed between the total anthocyanin, total flavonol, total phenolic acid content, and the antiradical activity of fruits (r = 0.99***; 0.93**, 0.83*, respectively) suggesting a significant influence of these specific classes of phenols on the antiradical activity of investigated fruits. Furthermore, a strong correlation of hydroxycinnamic acid contents with the antiradical activity (r = 0.85*) emphasized hydroxycinnamic acids as compounds that have significant influence on the antiradical activity within the phenolic acid class. These results are in accordance with selected literature data.[Citation18,Citation27,Citation28] The negative correlation coefficient between the flavan-3-ol content and the antiradical activity (r = −0.32) indicated that these compounds had no influence (or small influence) on the antiradical activity of fruits. The reason for the negative correlation is probably the low flavan-3-ol content in fruits. Moreover, to reveal individual phenols with higher influence on the antiradical activity of fruits, the correlations between the content of some individual phenolic compounds and the antiradical activity were observed. Quercetin derivatives and caffeic acid derivatives correlated highly with the antiradical activity (r = 0.93**; r = 0.94*, respectively). This suggested that quercetin derivatives, neochlorogenic acid, chlorogenic acid, and caffeic acid as individual phenolic compounds influenced strongly the antiradical activity of fruits. The strong antiradical activity of these compounds was shown earlier.[Citation29] It is clear that, since these compounds are present in higher amounts in investigated fruits, their relatively high concentrations contributed to the influence on the antiradical activity of fruits. Furthermore, fruit species studied in this work differed significantly in the phenol content. Anthocyanins were the dominant phenols in all fruits contributing with the highest portion to the antiradical activity. Besides anthocyanins, fruits were characterized by relatively higher content of some other phenolic compounds. Chokeberries, blueberries, blackberries, and elderberries were abundant in quercetin derivatives and caffeic acid derivatives. Hydroxycinnamic acids were present in relatively high concentrations in sour cherries, red raspberries, and strawberries. To see the influence of these phenols on the antiradical activity of these specific fruits, the correlations between the content of phenols in these fruits and the antiradical activity were observed. Total hydroxycinnamic acid content from sour cherries, red raspberries and strawberries correlated highly with the antiradical activity (r = 0.98). The quercetin derivative content and caffeic acid derivative content from chokeberries, blueberries, blackberries, and elderberries also correlated strongly with the antiradical activity (r = 0.94, r = 0.92, respectively). This suggested the strong influence of hydroxycinnamic acids on the antiradical activity of sour cherries, red raspberries and strawberries. The antiradical activity of chokeberries, blueberries, blackberries, and elderberries was influenced by quercetin and caffeic acid derivatives.
Table 3 Correlation coefficients (r) between the antioxidant activity (EC50) and contents of phenolic compounds in fruits (mg kg−1 FW)
The antiradical activity of fruits has a complex mechanism because of various phenolic compounds present in fruits and their interactions occurring in the fruits. In this study, various classes of phenols were isolated from fruits and their antiradical activity was screened and estimated independently. The results emphasized the anthocyanins as the compounds with the highest influence on the antiradical activity of investigated fruits. The anthocyanin concentrations in fruits were higher and, because of that, anthocyanins had higher influence on the antiradical activity in comparison to other investigated phenolic compounds. Moreover, the existence of high correlations suggested a strong influence of some other individual compounds. These compounds were caffeic acid derivatives (chlorogenic acid and neochlorogenic acid) and quercetin derivatives. The portion of these compounds in the antiradical activity was not as high as a portion of anthocyanins, but the presence of correlations suggested the influence exist. The influence of all phenols was concentration dependent. The higher the content of a certain phenol, the higher was the influence on the total antiradical activity of fruits. Furthermore, the compounds with the highest influence on the antiradical activity were different in different fruits. The total antiradical activity of chokeberries and blueberries was influenced mostly by anthocyanins but quercetin derivatives and caffeic acid derivatives (chlorogenic acid) also had a strong influence on the antiradical activity of these fruits. The antiradical activity of blackberries was influenced by anthocyanins and quercetin derivatives. Anthocyanins, caffeic acid derivatives (chlorogenic acid) and quercetin derivatives influenced the antiradical activity of elderberries. Anthocyanins and phenolic acids, especially p-coumaric acid derivatives, were responsible for the antiradical activity of sour cherries. Anthocyanins had a high influence on the antiradical activity of strawberries and red raspberries. These fruits were reported to have a significant amount of ellagitanins.[Citation12] Ellagitanins probably have a high portion in the total antiradical activity of strawberries. Apart from mentioned compounds, the antiradical activity of fruits is influenced by some other phenols not determined in this study. Chokeberries, blueberries, and elderberries were reported to contain proanthocyanidins[Citation13,Citation15] the compounds even stronger scavengers of DPPH radicals in comparison to flavonols and flavan-3-ols.[Citation29] Proanthocyanidins contribute probably a great deal to the antiradical capacity of these fruits. The basic structural units in proanthocyanidin chains are flavan-3-ols. In this study, only free monomeric flavan-3-ols were extracted from fruits and they had no influence on the antiradical activity (). If we look at these compounds as a part of proanthocyanidins, their influence could probably be higher. Fruits have also been reported to contain vitamin C. The content of vitamin C was 131 mg kg −1 in chokeberry,[Citation19] 124–131 mg kg−1 in blackberry,[Citation19] 60–250 mg kg−1 in elderberry,[Citation30] 155–163 mg kg−1 in raspberry,[Citation19] 324–847 mg kg−1 in strawberry[Citation31]). Although the antiradical potential of vitamin C was found to be weaker that that of quercetin and similar to that of Trolox,[Citation29] it can still contribute to the antiradical activity of berries and fruits.
CONCLUSION
The results of this study showed that the antiradical activity of investigated fruits was strongly influenced by anthocyanins. Besides anthocyanins, high influence on the antiradical activity had quercetin derivatives and caffeic acid derivatives (chlorogenic acid) in chokeberries and blueberries, quercetin derivatives in blackberries, quercetin- and caffeic acid derivatives (chlorogenic acid) in elderberries, and p-coumaric acid derivatives in sour cherries. The results of this study provide a better insight into the antiradical activity of dark colored berries and fruits and their potential health benefits.
ABBREVIATIONS
DPPH: 2,2-diphenyl-1-picrylhydrazyl radical; EC50: efficient concentration (concentration of antioxidant needed to reduce the DPPH concentration by 50 %); FW: fresh weight; HPLC: high performance liquid chromatography; PDA: photo diode array.
ACKNOWLEDGMENT
This work was supported by The Ministry of Science, Education, and Sports of Republic of Croatia (Project number 113-1130471-0451).
REFERENCES
- Olsson , M.E. , Gustavsson , K.E. , Andersson , S. , Nilsson , A. and Duan , R.D. 2004 . Inhibition of cancer cell proliferation in vitro by fruit and berry extracts and correlations with antioxidant levels . Journal of Agricultural and Food Chemistry , 52 ( 24 ) : 7264 – 7271 .
- Seeram , N.P. , Adams , L.S. , Zhang , Y. , Lee , R. , Sand , D. , Scheuller , H.S. and Heber , D. 2006 . Blackberry, black raspberry, blueberry, cranberry, red raspberry, and strawberry extracts inhibit growth and stimulate apoptosis of human cancer cells in vitro . Journal of Agricultural and Food Chemistry , 54 ( 25 ) : 9329 – 9339 .
- Garcia-Alonso , J. , Ros , G. , Vidal-Guevara , M.L. and Periago , M.J. 2006 . Acute intake of phenolic-rich juice improves, antioxidant status in healthy subjests . Nutrition Research , 26 ( 7 ) : 330 – 339 .
- Gungor , N. and Sengul , M. 2008 . Antioxidant activity, total phenolic content and selected physicochemical properties of white mulberry (Morus Alba L.) fruits . International Journal of Food Properties , 11 ( 1 ) : 44 – 52 .
- Rababah , T.M. , Ereifej , K.I. , Al-Mahasneh , M.A. , Ismaeal , K. , Hidar , A.G. and Yang , W. 2008 . Total phenolics, antioxidant activities, and anthocyanins of different grape seed cultivars grown in Jordan . International Journal of Food Properties , 11 ( 2 ) : 472 – 479 .
- Mandic , A.I. , Đilas , S.M. , Ćetković , G.S. , Čanadanović-Brunet , J.M. and Tumbas , V.T. 2008 . Polyphenolic composition and antioxidant activities of grape seed extract . International Journal of Food Properties , 11 ( 4 ) : 713 – 726 .
- Rotelli , A.E. , Guardia , T. , Juárez , A.O. , de la Rocha , N.E. and Pelzer , L.E. 2003 . Comparative study of flavonoids in experimental models of inflammation . Pharmacological Research , 48 ( 6 ) : 601 – 606 .
- Mamani-Matsuda , M. , Kauss , T. , Al-Kharrat , A. , Rambert , J. , Fawaz , F. , Thiolat , D. , Moynet , D. , Coves , S. , Malvy , D and Mossalayi , M.D. 2006 . Therapeutic and preventive properties of quercetin in experimental arthritis correlate with decrease macrophage inflammatory mediators . Biochemical Pharmacology , 72 ( 10 ) : 1304 – 1310 .
- Garcia-Closas , R. , Gonzalez , C.A. , Agudo , A. and Riboli , E. 1999 . Intake of specific carotenoids and flavonoids and the risk of gastric cancer in Spain . Cancer Causes and Control , 10 ( 1 ) : 71 – 75 .
- 2006 . Halliwell, B. Perspective. Polyphenols: Antioxidant treats for healthy living or covert toxins? . Journal of the Science of Food and Agriculture , 86 : 1992 – 1995 .
- Arabshahi-Delouee , S. and Urooj , A. 2007 . Application of phenolic extracts from selected plants in fruit juice . International Journal of Food Properties , 10 ( 3 ) : 479 – 488 .
- Määttä-Riihinen , K.R. , Kamal-Eldin , A. and Törrönen , A.R. 2004a . Identification and quantification of phenolic compounds in berries of Fragaria and Rubus species (Family Rosaceae) . Journal of Agricultural and Food Chemistry , 52 ( 20 ) : 6178 – 6187 .
- Määttä-Riihinen , K.R. , Kamal-Eldin , A. , Mattila , P.H. , González-Paramás , A.M. and Törrönen , A.R. 2004b . Distribution and content of phenolic compounds in eighteen Scandinavian berry species . Journal of Agricultural and Food Chemistry , 52 ( 14 ) : 4477 – 4486 .
- Wu , X. , Beecher , G.R. , Holden , J.M. , Haytowitz , D.B. , Gebhardt , S.E. and Prior , R.L. 2006 . Concentrations of anthocyanins in common foods in the United States and estimation of normal consumption . Journal of Agricultural and Food Chemistry , 54 ( 11 ) : 4069 – 4075 .
- Wu , X. , Gu , L. , Prior , R.L. and McKay , S. 2004 . Characterization of anthocyanins and proanthocyanidins in some cultivars of Ribes, Aronia, and Sambucus and their antioxidant capacity . Journal of Agricultural and Food Chemistry , 52 ( 26 ) : 7846 – 7856 .
- Zheng , W. and Wang , S.Y. 2003 . Oxygen radical absorbing capacity of phenolics in blueberries, cranberries, chokeberries, and lingonberries . Journal of Agricultural and Food Chemistry , 51 ( 2 ) : 502 – 509 .
- Jakobek , L. , Šeruga , M. , Medvidović-Kosanović , M. and Novak , I. 2007a . Anthocyanin content and antioxidant activity of various red fruit juices . Deutsche Lebensmittel-Rundschau , 103 ( 2 ) : 58 – 64 .
- Jakobek , L. , Šeruga , M. , Novak , I. and Medvidović-Kosanović , M. 2007b . Flavonols, phenolic acids and antioxidant activity of some red fruits . Deutsche Lebensmittel-Rundschau , 103 ( 8 ) : 369 – 378 .
- Benvenuti , S. , Pellati , F. , Melegari , M. and Bertelli , D. 2004 . Polyphenols, anthocyanins, ascorbic acid, and radical scavenging activity of Rubus, Ribes, and Aronia . Journal of Food Science , 69 ( 3 ) : 164 – 169 .
- Kim , D.O. , Heo , H.J. , Kim , Y.J. , Yang , H.S. and Lee , C.Y. 2005 . Sweet and sour cherry phenolics and their protective effects on neuronal cells . Journal of Agricultural and Food Chemistry , 53 ( 26 ) : 9921 – 9927 .
- Hernanz , D. , Recamales , A.F. , Meléndez-Martinez , A.J. , González-Miret , M.L. and Heredia , F.J. 2007 . Assessment of the differences in the phenolic composition of five strawberry cultivars (Fragaria ananassa duch) grown in two different soilless systems . Journal of Agricultural and Food Chemistry , 55 ( 5 ) : 1846 – 1852 .
- Riihinen , K. , Jaakola , L. , Kärenlampi , S. and Hohtola , A. 2008 . Organ-specific distribution of phenolic compounds in bilberry (Vaccinium myrtillus) and “northblue” blueberry (Vaccinium Corymbosum x V. angustifolium) . Food Chemistry , 110 ( 1 ) : 156 – 160 .
- Matilla , P. , Hellström , J. and Törrönen , R. 2006 . Phenolic acids in berries, fruits, and beverages . Journal of Agricultural and. Food Chemistry , 54 ( 19 ) : 7193 – 7199 .
- Voća , S. , Dobričević , N. , Družić , J. , Duralija , B. , Skendrović Babojelić , M. , Dermišek , D. and Čmelik , Z. 2009 . The change of fruit quality parameters in day-neutral strawberries cv. Diamante grown out of season . International Journal of Food Science and Nutrition , 60 ( 3 ) : 248 – 254 .
- Voća , S. , Dobričević , N. , Dragović-Uzelac , V. , Duralija , B. , Družić , J. , Čmelik , Z. and Skendrović Babojelić , M. 2008 . Fruit quality of new early ripening strawberry cultivars in Croatia . Food Technology and Biotechnology , 46 ( 3 ) : 292 – 298 .
- Tulio , A.Z. , Reese , R.N. , Wyzgoski , F.J. , Rinaldi , P.L. , Fu , R. , Scheerens , J.C. and Miller , A.R. 2008 . Cyanidin 3-rutinoside and cyanidin 3-xylosylrutinoside as primary antioxidants in black raspberry . Journal of Agricultural and Food Chemistry , 56 ( 6 ) : 1880 – 1888 .
- Bermúdez-Soto , M. and Tomás-Barberán , F.A. 2004 . Evaluation of commercial red fruit juice concentrates as ingredients for antioxidant functional juices . European Food Research and Technology , 219 ( 2 ) : 133 – 141 .
- Hukkanen , A.T. , Pölönen , S.S. , Kärenlampi , S.O. and Kokko , H.I. 2006 . Antioxidant capacity and phenolic content of sweet rowanberries . Journal of Agricultural and Food Chemistry , 54 ( 1 ) : 112 – 119 .
- Soobrattee , M.A. , Neergheen , V.S. , Luximon-Ramma , A. , Aruoma , O.I. and Bahorun , T. 2005 . Phenolics as potential antioxidant therapeutic agents: Mechanism and actions . Mutation Research , 579 ( 1–2 ) : 200 – 213 .
- Kaack , K. and Austed , T. 1998 . Interaction of vitamin C and flavonoids in elderberry (Sambucus nigra L.) during juice processing . Plant Foods for Human Nutrition , 52 ( 3 ) : 187 – 198 .
- Hakala , M. , Lapveteläinen , A. , Huopalahti , R. , Kallio , H. and Tahvonen , R. 2003 . Effects of varieties and cultivation conditions on the composition of strawberries . Journal of Food Composition and Analysis , 16 ( 1 ) : 67 – 80 .