Abstract
The wide variation of water absorption and water solubility index values of extruded food products makes their utilization difficult in food process applications. The published values of water absorption and water solubility indices of various extruded starchy and proteinaceous food products were classified and analyzed statistically in order to reveal the influence of die temperature, feed moisture content, and screw speed. A power law model relating the water absorption or water solubility index to independent extrusion variables was fitted to all literature data for each food product. The predicted values were carefully screened and successfully correlated to experimental values. In the case of the water absorption index, seven products and seven blends were evaluated and the correlation for most food products and blends was very good. However, in the case of the water solubility index, six products and two blends were evaluated and the correlation for most food products and blends was satisfactory.
INTRODUCTION
Extrusion is a technological process, commonly used in the field of the human and animal feed industry, applied successively on a broad spectrum of commercially manufactured products. Hot extrusion is a thermomechanical processing operation that makes use of high temperatures applied on the processed material for a short time. As a result of this process, a variety of chemical reactions and functional changes occur on the extruded material, which leads to changes of its sensory characteristics, nutritional value, and components and the reduction of the microbiological contamination.[Citation1–6,Citation17,Citation18] Conclusions upon the quality of the derived products can be drawn by measuring several functional properties of the final product. Usually most of the gathered data allow us to make further useful assumptions. In particular, water solubility index (WSI) and water absortion index (WAI) are two indices linked to the estimation of the behavior of the material if further processed for use as a binder, a stabilizer, or a source of protein in beverages, health and nutrition bars, dairy, baked, and emulsified/ground meat food systems.[Citation7–12]
Anderson et al.[Citation13] recorded a method to estimate the amount of material that can be extracted by water from an extruded product. The method involves milling the extruded product and then adding a known weight of powder to distilled water. The sample is then stirred and the suspension is poured into a tare centrifuge tube. After centrifugation, the supernatant is dried and the weight of solids in the supernatant is used to calculate the WSI as a percentage of dry weight of the extrudate. The weight of the sediment in the centrifuge tube is used to calculate the WAI as a percentage of the water bound per 100 g of dry extrudate. All the collected values are measured according to the above method. WSI and WAI values extend in a wide range of prices depending on the specific parameters applied during each extrusion process. Factors affecting the values of indices can be classified into two main groups: the first one consists of the parameters that are linked to the raw material subjected to extrusion, and the second one includes parameters related to the extruder equipment. In particular, the first group refers to the initial composition of the raw material, the raw material formulation, the pre-processing treatments, the initial particle size of milled materials, and the milling procedure.[Citation10–12] The second group is related to the extruder conditions, such as extruder type, last section barrel temperature, or die temperature, feed moisture content, feed rate, screw speed, screw configuration, screw compression ratio, die dimension, and die configuration.[Citation14,Citation15] Many previous studies related the final state of raw materials transformation during extrusion to such processing parameters as barrel temperature, water content, feed rate, or screw speed without examining the influence of the other factors on the final product.
Various models have been proposed based on statistical analysis, in order to correlate the WAI or WSI with the barrel temperature, water content, and screw speed for the extruded food products. These models are generally second- or third-order polynomials and describe the effects of independent variables in the first, second, and third order, as well as the product between them on WAI or WSI. Unfortunately, comprehending the physical values of these second- and third-order polynomials or the products between the independent variables is very difficult. The present study proposes a mathematical model that overcomes the above difficulties in understanding, as it is a simple power law model. The proposed mathematical model investigates the effect of die temperature, feed moisture content, and screw speed on the WAI and WSI properties for some food products. The model is fitted to relevant data available from the literature and the model parameters were estimated for every category of food products.
DATA-MATHEMATICAL MODEL
According to Oikonomou and Krokida[Citation15] a database was developed, which includes experimental values for WAI and WSI from a wide range of extruded food products. Part of this database (63 articles which include 877 and 577 values for WAI and WSI, respectively) was used for statistical analysis and modeling mainly for starchy and proteinaceous extruded food products (). The WAI and WSI can be predicted using a model, which considers power law dependency of all independent variables:
Table 1 Number of papers and literature data which were used for analysis
STATISTICAL ANALYSIS
Curve fitting was performed using the solver function in Microsoft® Office Excel 2003 (XP Edition, Microsoft Corporation, WA, USA) adopting the nonlinear optimization equation to determine the parameters, a, b, c, d, and e in the power law model (EquationEqs. 1 and Equation2). Therefore, the parameters are estimated simultaneously by minimization of the residual sum of squares (SST):[Citation16,Citation17]
A model is considered acceptable if the standard deviation between experimental and calculated values is close to the standard experimental error
. The parameters described above do not have the same affect on the SST. In order to check if the model contains the required number of parameters for predicting the values of WAI and WSI, the following process was adopted. Initially excluding one parameter at a time, the values of
were calculated for all the remaining parameters. The parameter selected for elimination was the one that, when excluded, produced the minimum
. Continuing the former procedure, the minimum values of
were evaluated for (4), 3, 2, and 1 parameters, respectively. Another statistical test that determines the accuracy of the parameter estimates is the calculation of the confidence intervals (95%) for each parameter and for all the products ( and ).
RESULTS
The results of the nonlinear regression analysis of fitting EquationEq. (1) to the experimental points are shown in . In this table, the standard experimental error (EquationEq. 5) and the standard deviation between experimental and calculated values
(EquationEq. 6) are also presented. In most cases the values of the standard experimental error
are a little smaller (or a little bigger in the cases of corn starch: whey protein concentrate (WPC) and rice starch: sou protein isolate (SPI)) than the values of the standard deviation between the experimental and calculated values
indicating that the model (EquationEq. 1) is satisfactory in predicting the WAI of products. In the cases of wheat, wheat: distiller's dried grains (DDG), and potato:DDG, the difference in the values was a little bigger than that in the above cases showing that there is an adequate fit to EquationEq. (1). Similarly, in the results of the nonlinear regression analysis of fitting EquationEq. (2) for WSI are presented. In most cases, the values of the standard experimental error
are relatively close to the values of standard deviation between experimental and calculated values
. Therefore, the model provides a good fit for the various food products.
Table 2 Standard experimental error (
), standard deviation between experimental and calculated values (
), parameters of the model and the experimental limits of die temperature (T), feed moisture content (X), screw speed (S), and the mixture (M) for the WAI prediction
Table 3 Standard experimental error (
), standard deviation between experimental and calculated values (
), parameters of the model and the experimental limits of die temperature (T), feed moisture content (X), screw speed (S), and the mixture (M) for the WSI prediction
The experimental limits of independent variables are shown in and . The same limits are used for the prediction of WAI and WSI. The variation of experimental WAI values ranges from 0.3 to 12.8, while predicted values of WAI varied from 1.3 to 9.5. Experimental WSI values varied from 2.7 to 73.2%, while predicted WAI varied from 4.5 to 61.6% for all extruded food products. The smaller range of predicted values compared to experimental values may be explained by the low ability of the model to predict accurate values at low and high values of independent variables.
The plots, which relate the WAI and WSI, with die temperature of extruded products at X = 15% and S = 150 rpm are presented in a and a, respectively. An increase in die temperature does not have a consistent effect on experimental and predicted WAI and WSI for all raw materials examined. a presents the plot of WAI versus die temperature at X = 15%, S = 150 rpm, and M = 25% for blends. In general, an increase in die temperature leads to a slight increase of WAI, except for starch and the blend of starch:DDG. Similar results were obtained for the other ranges of independent variables (data not shown). b illustrates that the WAI increases sharply with feed moisture content for all of the extruded food products, except for wheat and oat products. An increase in feed moisture content leads to a slight decrease in the WSI for all food products, except beans (b). In the extruded blends, the effect of feed moisture content on WSI has no definite correlation for the food products (b). In c and c the curves do not have a distinct slope as screw speed increases for all the extruded products. As can be seen, the increase in mixture on proteinaceous materials has as a result a slight decrease in WAI (c). Overall, when the extrusion variables are increased, the WAI and WSI are increased only in the cases of corn, rice, beans, starch, corn:lentil, rice, and wheat products, respectively.
Figure 1 Effect of (a) die temperature °C; (b) feed moisture content %; (c) screw speed rpm on WAI for barley (▴), corn (•), oat (■), rice (x), wheat (◊), beans (○), and starch (□). Lines are calculated model values using parameters given in .
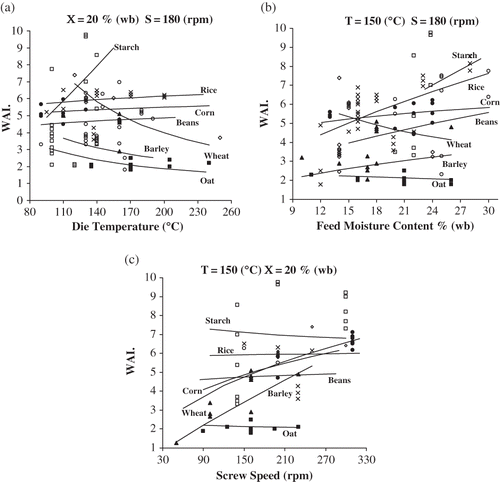
Figure 2 Effect of (a) die temperature °C; (b) feed moisture content %; (c) screw speed rpm on WSI for corn (•), oat (■), rice (x), wheat (◊), beans (○), and starch (□). Lines are calculated model values using parameters given in .
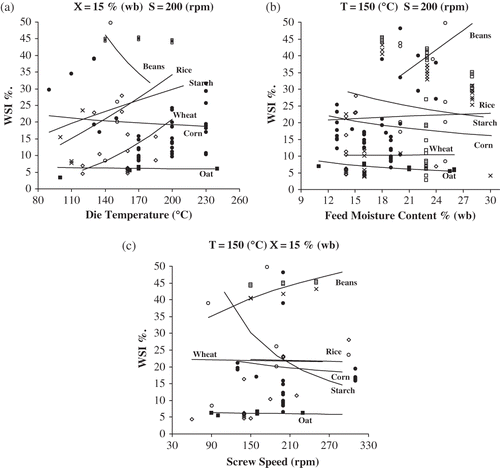
Figure 3 Effect of (a) die temperature °C; (b) feed moisture content %; (c) mix lever % on WAI for: (1) (▲) corn:DDG, (2) (•) corn:lentil, (3) (■) rice:DDG, (4) (x) wheat:DDG, 5 (◊) corn starch:WPC, (6) (○) rice starch:SPI, and (7) (□) potato:DDG. Lines are calculated model values using parameters given in .
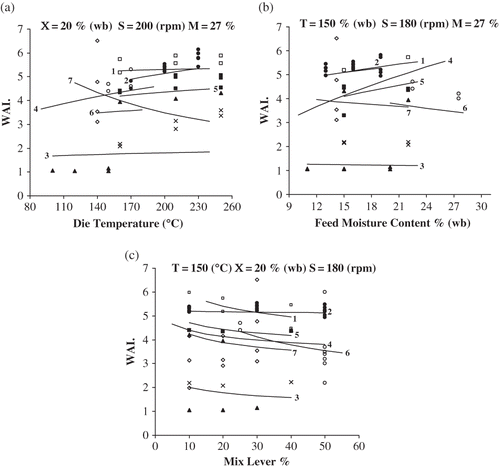
and b present typical charts of and
as a function of the number of parameters for WAI the rice and for WSI the blend corn:lentil. The results presented on the charts indicate that the number of parameters is necessary for the accurate prediction of the model. In and , the confidence intervals (95%) for all parameters for all food products are shown for WAI and WSI. These tables include parameters with confidence intervals that range from negative to positive values, showing that these specific parameters are not required in EquationEqs. (1) and Equation(2). The products with two or more parameters having zero within the range of their confidence intervals are also those products where the differences in the values of
and
was great, such as wheat, beans, and wheat:DDG.
Figure 4 The lack of fit () and standard experimental error (
) as a function of the number of parameters: (a) predicted model for WAI of rice; (b) predicted model for WSI of blend corn:lentil.
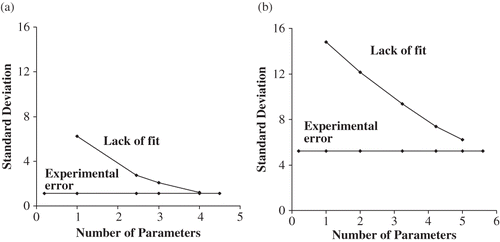
Table 4 Confidence intervals (95%) for the parameters of the model for the WAI prediction
Table 5 Confidence intervals (95%) for the parameters of the model for the WSI prediction
CONCLUSION
The present study proposes a mathematical model that investigates the effects of the main extrusion variables on the WAI and WSI properties for some food products. The model is fitted to literature data and the model parameters were estimated for every category of food products. The method used for curve fitting was a nonlinear regression that was based on the minimization of residual sum of squares. The check of the accuracy of the model and the need for the number of parameters was undertaken with the aid of the standard experimental error , standard deviation between experimental and calculated values
, and the confidence intervals of the parameters, respectively. In most cases, the modeling of the WAI of food products showed that the power law equation is fitted satisfactory to the available experimental values. In contrast, when modeling WSI the fit of the power law equation to experimental data is adequate. Unfortunately, enough data exists only for limited products to carry out a successful regression analysis.
NOMENCLATURE
WAI | = |
Water absorption index |
WSI | = |
Water solubility index |
T | = |
Die or last section temperature of extruder (°C) |
X | = |
Feed moisture content of food product in wet base % |
S | = |
Screw speed of extruder (rpm) |
M | = |
% mixture of blend |
| = |
Standard experimental error |
| = |
Standard deviation between experimental and calculated values |
a, b, c, d, e | = |
Dimensionless parameters |
| = |
Experimental value of WAI or WSI |
| = |
Calculated value of WAI or WSI |
| = |
Arithmetic mean of experimental value of WAI or WSI |
Subscripts | = | |
= |
Reference value of independent variables |
REFERENCES
- Allen , K.E. , Carpenter , C.E. and Walsh , M.K. 2007 . Influence of protein level and starch type on an extrusion-expanded whey product . International Journal of Food Science and Technology , 42 ( 8 ) : 953 – 960 .
- Amaya-Llano , S.L. , Morales Hernández , N. , Castaño Tostado , E. and Martínez Bustos , F. 2007 . Functional characteristics of extruded blends of whey protein concentrate and corn starch . Cereal Chemistry , 84 ( 2 ) : 195 – 201 .
- Fellows , P. 2000 . Food Processing Technology Principles and Practice , 2nd , 294 – 308 . Boca Raton , FL : CRC Press .
- Gajula , H. , Liu , S. , Alavi , S. , Herald , T. , Madl , R. , Bean , S.R. and Tilley , M. 2009 . Pre-cooked fiber-enriched wheat flour obtained by extrusion: Rheological and functional properties . International Journal of Food Properties , 12 ( 1 ) : 27 – 44 .
- Martínez-Bustos , F. , Chang , Y.K. , Bannwart , A.C. , Rodríguez , M.E. , Guedes , P.A. and Gaiotti , E.R. 1998 . Effects of calcium hydroxide and processing conditions on corn meal extrudates . Cereal Chemistry , 75 ( 6 ) : 796 – 801 .
- Sablani , S.S. 2009 . “ Gelatinization of starch ” . In Food Properties Handbook , 2nd , Edited by: Rahman , S.M. 287 – 288 . Boca Raton , FL : CRC Press .
- Kim , C.H. , Maga , J.A. and Martin , J.T. 1989 . Properties of extruded blends of wheat dried distiller grain flour with other flours . International Journal of Food Science and Technology , 24 ( 4 ) : 373 – 384 .
- Matthey , F.P. and Hanna , M.A. 1997 . Physical and functional properties of twin-screw extruded whey protein concentrate–corn starch blends . LWT—Food Science and Technology , 30 ( 4 ) : 359 – 366 .
- Pelembe , L.A.M. , Erasmus , C. and Taylor , J.R.N. 2002 . Development of a protein-rich composite sorghum-cowpea instant porridge by extrusion cooking process . LWT—Food Science and Technology , 35 ( 2 ) : 120 – 127 .
- Sharma , H.R. , Chauhan , G.S. and Agrawal , K. 2004 . Physico-chemical characteristics of rice bran processed by dry heating and extrusion cooking . International Journal of Food Properties , 7 ( 3 ) : 603 – 614 .
- Singh , J. and Singh , N. 2004 . Effect of process variables and sodium alginate on extrusion behavior of nixtamalized corn grit . International Journal of Food Properties , 7 ( 2 ) : 329 – 340 .
- Sopade , P.A. and Le Grys , G.A. 1991 . Effect of added sucrose on extrusion cooking of maize starch . Food Control , 2 ( 2 ) : 103 – 109 .
- Anderson , R.A. , Conway , H.F. , Pfeifer , V.F. and Griffin , E.L. 1969 . gelatinisation of corn grits by roll and extrusion cooking . Cereal Science Today , 14 ( 1 ) : 4 – 12 .
- Balandran-Quintana , R.R. , Barbosa-Cánovas , G.V. , Zazueta-Morales , J.J. , Anzaldύa-Morales , A. and Quintero-Ramos , A. 1998 . Functional and nutritional properties of extruded whole pinto bean meal (Phaseolus vulgaris L.) . Journal of Food Science , 63 ( 1 ) : 113 – 116 .
- Oikonomou , N.A. and Krokida , M.K. 2011 . literature data of WAI and WSI of extrudate food products . International Journal of Food Properties , 14 : 199 – 240 .
- Draper , N.R. and Smith , H. 1998 . Applied Regression Analysis , 3rd , 47 – 54 . NY , , USA : John Wiley and Sons .
- Kiranoudis , C.T. , Maroulis , Z.B. , Tsami , E. and Marinos-Kouris , D. 1993 . Equilibrium moisture content and heat of desorption of some vegetables . Journal of Food Engineering , 20 : 55 – 74 .
- Maroulis , Z.B. and Saravacos , G.D. 2007 . Food Plant Economics , Boca Raton , FL : CRC Press .
- Maroulis , Z.B. and Saravacos , G.D. 2003 . Food Process Design , 2 – 13 . NY , , USA : Marcel Dekker .
- Saravacos , G.D. and Maroulis , Z.B. 2001 . Transport Properties of Foods , 283 – 284 . NY , , USA : Marcel Dekker .