Abstract
Vigna unguiculata protein concentrate was enzymatically hydrolyzed with Alcalase® and Flavourzyme® and the resulting hydrolysates, with the highest respective degrees of hydrolysis, functional, and nutritional properties, were evaluated. Hydrolysis was conducted for 5, 10, 15, and 60 min. Degree of hydrolysis values increased from 10.56 and 3.26%, respectively, at 5 min to 23.61 and 7.27%, respectively, at 60 min. The Flavourzyme® hydrolysate exhibited higher solubility and surface hydrophobicity than the Alcalase® hydrolysate. A large number of low molecular weight bands in the Alcalase® hydrolysate suggested that hydrolysis was extensive (DH = 23.6%). Both hydrolysates exhibited digestibility and intestinal absorption levels comparable to a casein control.
INTRODUCTION
Animal proteins, such as meat, milk, and eggs, are generally expensive and relatively difficult to acquire, which has led to a worldwide increase in research into vegetable protein sources. Because of their very high protein content, legumes have formed an important focus of this search for cheaper, alternative protein sources.Citation[1] As occurs in many tropical regions, a wide variety of legume crops, including cowpea Vigna unguiculata, are grown on the Yucatan Peninsula, Mexico. Cowpea seeds are an excellent carbohydrate (50–60 g/100 g) and protein (18–35 g/100 g) source, making it a promising, non-conventional alternative source for these nutrients.Citation[2] Incorporation of cowpea seed flour into most foods raises protein content, although the advantages of this supplementation are often limited by the presence of restricting antinutritional factors, such as phytic acid, protease inhibitors, and flatulence-causing sugars.Citation[3] In response, researchers have turned their attention to the use of legume seed protein concentrates and hydrolysates, rather than flours, since they have superior functional properties and are free of toxic factors and indigestible carbohydrates.Citation[4] Hydrolyzed proteins may, therefore, prove beneficial for patients suffering from specific digestive disorders, such as cystic fibrosis or short bowel syndrome, or prove to be useful in high protein diets to treat malnutrition.Citation[5]
Chemical processes, including acidic or alkaline media, have been used to hydrolyze proteins, although these can reduce protein quality through oxidation, destruction, or modification of some amino acids.Citation[6] Enzymatic hydrolysis is, therefore, preferred because it can improve native protein physicochemical, functional, and sensory properties without affecting nutritional value.Citation[7] These protein hydrolysates' physicochemical properties are intrinsically linked to parameters, such as: (1) protein substrate purity; (2) protein substrate pretreatment; (3) specificity of the enzyme used for proteolysis; (4) physicochemical conditions during hydrolysis (e.g., pH, temperature, ionic strength, activator); (5) degree of hydrolysis; (6) enzyme inactivation technique (e.g., heat treatment, acidification, or membrane filtration); and (7) use of post-hydrolysis treatments (e.g., free amino acid adsorbents, membrane separation).Citation[8]
Industrial hydrolysates are currently classified into three groups: (1) low (<10%) degree of hydrolysis (DH), with improved functional properties; (2) variable (usually high) DH, used for taste enhancement; and (3) high (>10%) DH, also called extensive, for use in nutritional supplements and medical diets. The latter includes hydrolysates aimed at improving the source protein's nutritional characteristics, and their high solubility and optimal intestinal absorption have brought them wide use in diets for athletes, the elderly, and those with special dietary requirements.Citation[9]
Hydrolytic enzymes enhance the functional properties of protein foods without deteriorating their nutritional value.Citation[10] Indeed, if done properly, enzymatic protein hydrolysis can improve the physical, chemical, and organoleptic properties of the source food.Citation[11] Alcalase® and Flavourzyme® are industrially produced enzymes with distinct advantages and disadvantages. Produced by Novo Nordisk A/S, Alcalase® is a nonspecific serine-type protease derived from Bacillus licheniformis with subtilisin as its main enzymatic component. It is a promising candidate for industrial hydrolysate production because of its broad specificity endoprotease activity, low cost, and high tolerance for alkaline pHs.Citation[12] Flavourzyme® is an exopeptidase-endoprotease complex generated by Aspergillus orizae, which can generate very high DH and diminish the bitter off-taste produced in some products.Citation[13] The objective of the present study was to evaluate the effect of enzymatic hydrolysis of cowpea Vigna unguiculata protein concentrates on the solubility, hydrophobicity, and in vivo bioavailability of the resulting hydrolysates and to record their molecular weight profiles.
MATERIALS AND METHODS
Seeds and Chemicals
V. unguiculata seeds were obtained from the February 2004 harvest in the state of Yucatan, Mexico. After sun-drying for 8 h, the seeds were ground in a disk mill (model 4-E Quaker, Mill Straub Co., Philadelphia, PA, USA) and sifted through 4.76 and 2.38 mm screens. Hulls were removed with a fluidizing air bed. The dehulled flour was milled in a Cyclotec mill (Tecator, Hoganas, Sweden) until passing through a 0.841 mm screen. All chemicals were reagent grade and purchased from J.T. Baker (Phillipsburg, NJ, USA).
V. unguiculata Protein Concentrate
Protein concentrate was extracted following Betancur-Ancona et al.Citation[14] A flour/water (1:6 w/v) dispersion was prepared, its pH adjusted to 11 with NaOH 1N, and the suspension stirred for 1 h at 400 rpm with a homogenizer (Heidolph, Silent Crusher RZ-1, Schwabach, Germany). The suspension was then poured through 80-mesh (0.19 mm pore size) and 100-mesh (0.14 mm pore size) screens to separate the solid fraction (fiber) from the liquid fraction (protein and starch). Residual solids were washed five times at a 1:1 solid to distilled water ratio. The wash water was mixed with the supernatants from the initial suspension and allowed to sediment for 30 min to recover the starch and separate the solubilized protein. Solubilized protein pH was adjusted to its isoelectric point (4.5) with HCl 1N, and the suspension centrifuged at 1317× g for 12 min with a Mistral 3000i (Curtin Matheson Sci., Houston, TX, USA) centrifuge. The supernatants were discarded and the precipitates freeze-dried at −47°C and 13 × 10−3 mbar.
Enzymatic Hydrolysis
Enzymatic hydrolysis was done according to Hamada et al.,Citation[15] using a random block design. The blocks were the enzymes (Alcalase® or Flavourzyme®), the factor to evaluate was reaction time (5, 15, 30, and 60 min), and the response variable was degree of hydrolysis. The protein concentrate (5 g) was dispersed in 250 mL of deionized water, pH adjusted to 8 with NaOH 1N, and 25 mg of enzyme added. The reaction was run at 50°C with constant stirring for 1 h; 25 mL samples were taken at 5, 15, 30, and 60 min to measure degree of hydrolysis. The hydrolysis reactions were run in a beaker equipped with a stirrer, thermometer, and pH electrode, and stopped by heating to 85°C for 15 min. The dissolved protein was centrifuged at 10,000 rpm for 20 min to extract the soluble fraction.
Degree of Hydrolysis
Degree of hydrolysis (% DH) was determined using the method of Kim et al.Citation[16] in which the value is estimated by measuring soluble nitrogen content in 10% trichloroacetic acid (TCA). A 10-mL sample of hydrolysate was mixed with 10 mL of 20% TCA and centrifuged at 12,100 g for 15 min. Soluble nitrogen in the supernatant was assayed by the Kjeldahl method,Citation[17] and percent DH was expressed as:Equation
Solubility, Hydrophobicity, and In Vivo Digestibility
The Alcalase® (AH) and Flavourzyme® (FH) hydrolysates exhibiting the highest DH on the curve (i.e., those produced at 60 min reaction time) were selected to evaluate solubility, hydrophobicity, and in vivo digestibility, as well as for electrophoretic characterization. Nitrogen solubility was determined by the method of Were et al.Citation[18] Either AH, FH, or protein concentrate (0.5%) was dispersed in 25 mL of deionized water and adjusted to pH 2.0, 4.0, 6.0, 8.0, or 10.0 with either 0.01 or 1.0 N NaOH or HCl. The dispersions were shaken for 30 min at room temperature and centrifuged at 43,503 g for 30 min. Nitrogen content in the supernatant was determined by the Kjeldahl method,Citation[17] and the percent soluble protein was calculated as:Equation
Surface hydrophobicity was measured with a hydrophobic fluorescence probe method using 1-anilino-8-naphthalene sulfonate (ANS).Citation[19] Protein hydrolysate samples at concentrations of 0.0, 0.05, 0.1, 0.2, 0.3, 0.4, and 0.5% were prepared by serial dilution with 0.1 M phosphate buffer (pH 7), and 25 μL ANS (8 mM in 0.1 M phosphate buffer) added to these protein solutions. The fluorescence intensities of ANS-protein conjugates were measured using a Perkin-Elmer 2000 fluorometer (Perkin-Elmer Corp., Norwalk, CT, USA) at a 364-nm excitation wavelength and 484-nm emission. Fluorescence intensity slope vs. percent protein concentration was calculated by linear regression, and this was the figure used as the protein surface hydrophobicity index.
Electrophoretic Characterization
Sodium dodecyl sulphate polyacrylamide gel electrophoresis of protein hydrolysates was performed following the method of Schägger and Jagow.Citation[20] All gels were run in a Mini-PROTEAN 2 System (Bio-Rad), with 1 μg of each hydrolysate diluted in 200 μl of sample buffer and 4 μl of each solution placed on each lane. Molecular weight standard (BioRad 161–0305, Hercules, CA, USA) protein composition was: phosphorylase (103 kDa); bovine serum albumin (77 kDa); ovalbumin (50 kDa); carbonic anhydrase (34.3 kDa); trypsin inhibitor (28.8 kDa); and lysozyme (20.7 kDa). Runs were done at a constant amperage (20 mA/gel) for 1.15 h. Gels were fixed and stained with Coomassie Brilliant Blue stain 0.10% for 30 min under constant shaking and destained with water:acetic acid:methanol mixture (5:1:2 v/v/v ratio) for 24 h.
In Vivo Digestibility
To evaluate in vivo digestibility, diets were prepared containing casein, Alcalase® hydrolysate, and Flavourzyme® hydrolysate (), and tested according to Calderón de la Barca et al.Citation[21] Experimental animals were 24 male Sprague Dawley rats of 24 or 31 days of age housed in individual cages and kept at 25°C, a 12 h:12 h light-dark cycle, and 50–65% relative humidity. The animals were acclimated for 7 days with ad libitum access to the casein diet, then randomly assigned to one of the three diets (three groups of six rats each). Animal weight and protein ingested and excreted (in feces) were measured daily in order to calculate apparent digestibility (AD) (Citation22):Equation
Table 1 Composition (%) of experimental diets containing casein (C), Alcalase® hydrolysate (AH), or Flavourzyme® hydrolysate (FM) as a protein source
Statistical Analysis
Degree of hydrolysis results were analyzed in triplicate using a two-way analysis of variance (ANOVA) with random blocks and a least significant difference (LSD) test. A student t analysis was used to identify differences in hydrolysate solubility. A one-way ANOVA was applied to evaluate in vivo digestibility and a LSD test to identify differences between treatments, according to Montgomery.Citation[23] All statistical analyses were done with the Statgraphics plus version 5.1 software (Statpoint Technologies Inc., Warrenton, VA, USA).
RESULTS AND DISCUSSION
Enzymatic Hydrolysis
Hydrolysis reaction progress was monitored by measuring the TCA index as estimation of the degree of hydrolysis (). This differed (P < 0.05) depending on the enzyme used (i.e., Alcalase® [endoprotease] or Flavourzyme® [protease complex]) and reaction time. The hydrolysis curves for Alcalase® and Flavourzyme® exhibited behavior characteristic of enzyme/protein systems, which can be linked to saturation and/or inhibition phenomena in the substrate or product.Citation[24] Initial DH values were 10.55% for AH and 3.25% for FH. Hydrolysis with Alcalase® preceded quickly during the first 5 min and increased steadily thereafter, reaching 23.6% DH at 60 min. Flavourzyme®, in contrast, produced only minimal increases in DH values, with a value of 7.27% at 60 min. Hydrolysis with Flavourzyme® was clearly not as extensive as with Alcalase®.
Figure 1 Changes in degree of hydrolysis (%) over time of V. unguiculata protein concentrates treated with Alcalase® and Flavourzyme®. a–dDifferent letters in the same reaction time and in the same enzyme indicate statistical difference (P < 0.05).
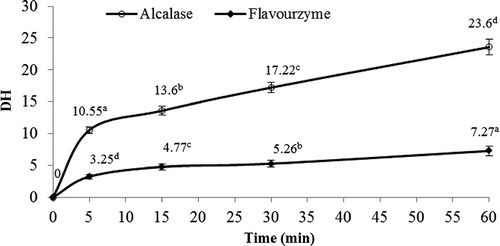
The DHs observed in the present study were lower than those reported for other vegetable protein sources. Using the same enzymes with sunflower proteins and a reaction time of 180 min, Villanueva et al.Citation[25] reported DH values ranging from 34.7 to 42.2%. Vioque et al. Citation[26] reported a DH of 60% using a mixture of Alcalase® and Flavourzyme® to digest rapeseed protein for 180 min. When hydrolyzing chickpea protein sequentially with Alcalase® and Flavourzyme® for 150 min, Pedroche et al.Citation[27] recorded a DH of 65%, while Hong et al.Citation[28] observed a 22% DH after hydrolyzing mung bean (Phaseolus vulgaris L.) protein concentrate with Alcalase® for 10 h. However, the present results are similar to those reported by Betancur-Ancona et al. Citation[29] after hydrolyzation of P. lunatus protein with Alcalase® or Flavourzyme® for 5, 10, 15, and 60 min. In that study, Alcalase® hydrolysis produced a DH of 11% at 5 min, was asymptotic after 10 min, and reached a maximum of 16.9% at 60 min; in other words, the enzymatic preparation produced a rapid initial reaction and then increased only gradually. Hydrolysis with Flavourzyme® was even less effective since it reached 2.8% DH at 5 min and only 7.7% DH at 60 min. A similar behavior was reported by Wasswa et al.Citation[30] after hydrolyzation of Nile perch, Grass carp, and Nile tilaphia with Alcalase® for 85 min and to observe that all curves exhibited an initial fast reaction followed by a slowdown.
Functional and nutritional characterizations were done using the V. unguiculata protein hydrolysates produced at 60 min reaction time with Alcalase® (AH) and Flavourzyme® (FH) because they exhibited the highest DH, estimated by measuring soluble nitrogen content in 10% TCA.
Nitrogen Solubility, Hydrophobicity, and In Vivo Digestibility
Solubility is the most important functional property of a protein since much of its functional behavior depends on its capacity to initially enter into solution. Comparison of the nitrogen solubility profiles for the AH and FH showed them to be enzyme- and pH-dependent (P < 0.05), with ranges of 29.05 to 34.35% (AH) and 27.18 to 93.67% (FH) (). Solubility values were higher, and variation among them was much more pronounced and pH-dependent in the FH than in the AH. The highest nitrogen solubility was observed with the FH (93.67%) at pH 8, which was different (p < 0.05) than values for AH (34.36%) and the protein concentrate (45.13%). At pH values near the isoelectric point (pH 4–6), enzymatic hydrolysis with Alcalase® and Flavourzyme® raised nitrogen solubility of the protein concentrate. At acid and alkaline pH values, the FH exhibited much higher solubility, producing a typical “V” pattern, whereas the AH exhibited no significant changes in solubility over the tested pH spectrum. This stark contrast in solubility profile between the hydrolysates was caused by the much higher DH in the AH, which generated new peptides with electrical charges differing from those of the unhydrolyzed protein. Similar behavior has been reported in sunflower protein concentrate hydrolysates, where solubility is independent of pH at extensive DHs but pH-dependent at low DHs.Citation[31] A different pattern in solubility profiles has been reported for P. lunatus protein hydrolysates in which nitrogen solubility was highest and dependent of pH at extensive DHs (11.8–16.1% with Alcalase®) but lowest and pH-independent at low DHs (2.8 and 6.3% with Flavourzyme®).[29]
Figure 2 Nitrogen solubility of V. unguiculata protein concentrate and hydrolysates generated with Alcalase® and Flavourzyme®. a–cDifferent letters in the same pH value indicate statistical difference (P < 0.05).
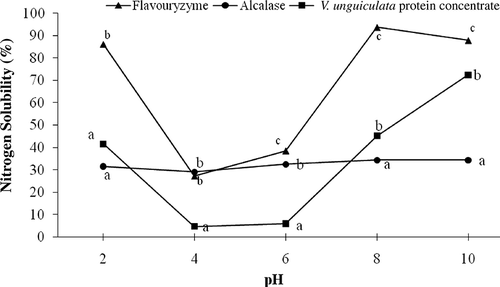
Solubility changes in the protein hydrolysates compared to the protein concentrate may have been caused by a reduction in molecular weight of the enzymatically modified proteins. The increased solubility observed in the FH would, therefore, be due to the smaller peptides generated by hydrolysis with this protease complex, and to exposure of their hydrophilic groups, which would increase interactions between the hydrophilic amino acids and water molecules.Citation[32] This property makes the cowpea FH potentially useful in applications requiring high solubility profiles to impart certain characteristics to a food formulation; for instance, baby food, baked products, carbonated drinks, diet drinks, and desserts.[1]
The AH (23.61% DH) had a substantially lower surface hydrophobicity (So = 30.18) than the FH (7.27% DH) (So = 291.52), probably because Flavourzyme® breaks the protein in very different ways and at different sites than Alcalase®. Flavourzyme® also produces more peptides with low aromatic amino acids contents,Citation[33] which would create hydrophobic areas and lead to higher hydrophilic amino acids content. Similar results have been reported in P. lunatus protein hydrolysates produced with Alcalase® and Flavourzyme® at 5 or 15 min. So, the Flavourzyme®-prepared hydrolysates were higher than in the Alalase®-prepared hydrolysates, which increased from 382 (5 min; 2.8% DH) to 655 (15 min, 6.3% DH) and from 251 (5 min; 11.8% DH) to 279 (15 min, 16.1% DH), respectively.[29]
Hydrolysis of V. unguiculata with Alcalase® or Flavourzyme® unfolded the protein molecule, although the extent of unfolding was probably greater with Flavourzyme®, resulting in different surface hydrophobicities. The higher So in the FH was also at least partially caused by greater flexibility in the modified molecules resulting in increased exposure of hydrophobic groups to the probe. This coincides with higher hydrophobicity (So = 40) reported in soy protein concentrate hydrolyzed with papain.[18] Hettiarachchy and Kalapathy[32] also observed increased hydrophobicity in a soy protein hydrolysate and concluded this behavior was probably due to exposure of the hydrophobic groups caused by unfolding of the protein. Hydrophobic groups are generally buried inside the core of native proteins' folded structure. Partial enzymatic hydrolysis would expose some of the hydrophobic groups, increasing hydrophobicity. This increase in hydrophobic groups could facilitate interactions between them and oils.
Peptide hydrophobicity produced by enzymatic hydrolysis is an important factor in enhancing protein surface properties, and high surface hydrophobicity can contribute to enhanced foaming capacity since foamability is associated with high surface hydrophobicity.Citation[34] The high surface hydrophobicity of the FH compared to the AH could provide better foaming properties because unfolding of the protein molecule exposes the hydrophobic groups, potentially increasing interactions at the air/water interface.
Electrophoretic Characterization
Electrophoresis showed the unhydrolyzed protein concentrate to have a complex protein profile characterized by seven bands between 21 and 103 kDa and one below 20.7 (). The AH exhibited six bands with molecular weights between 21 and 60 kDa and two bands below 20.7 kDa, while the FH exhibited four bands between 22 and 60 kDa and one below 20.7 kDa. The greater number of low molecular weight bands in the AH suggests that hydrolysis was extensive (23.61% DH), consequently producing small peptides. This agrees with reports that protein hydrolysis produces low molecular weight peptidesCitation[35]and that this is associated with enzyme specificity on specific substrates.[7] Given this, the lower number of electrophoretic bands in the FH was caused by its lower DH (7.27%), and was probably due to the globular structure of its globulins, the principal protein in legumes and a limiting factor for action with a single proteolytic enzyme. At this respect, Clemente et al.Citation[36] suggest the use of a sequential hydrolysis with an endoprotease and an exoprotease to solve this problem. The cleavage of peptide bonds by the endopeptidase Alcalase® increases the number of end peptide sites for the action of the exoprotease Flavourzyme®.
In Vivo Digestibility
Apparent digestibility (AD) of the diets containing the AH (99.79%), FH (99.78%), and casein (99.87%) were not statistically different (P < 0.05) (). These values are higher than reported for rice (84–87%), sunflower (78%), corn (83–84%), sesame (78.9%), soybean (91–94.4%), and wheat (75–89.6%). Both hydrolysates clearly had very good nutritional quality in that they were almost completely digested and absorbed; this is a significant property because AD is an essential parameter in evaluating ingredient quality. These hydrolysates' high AD values provide them potential uses in improving protein nutritional qualities in nutritional supplements and medical diets.
Table 2 Ingested and excreted nitrogen and apparent digestibility in diets containing V. unguiculata protein hydrolysates produced with Alcalase® or Flavourzyme® and a control diet containing casein
CONCLUSIONS
Enzymatic hydrolysis of V. unguiculata protein concentrate for 60 min with Alcalase® produced a hydrolysate with a higher DH than when hydrolyzed with Flavourzyme® for the same reaction time. Both hydrolysates exhibited improved solubility at pH values near the isoelectric point, although the Flavourzyme® hydrolysate had the highest nitrogen solubility at acid and alkaline pHs, and the highest surface hydrophobicity. Electrophoresis showed the Alcalase® hydrolysate to have a large number of low molecular weight bands, indicating that hydrolysis was more extensive with this endoprotease. Apparent digestibility of diets containing the Alcalase® or Flavourzyme® hydrolysates showed them to be almost completely digested and absorbed, meaning they are of good nutritional quality. Enzymatic modification of a V. unguiculata protein concentrate with Alcalase® or Flavourzyme® substantially improves its potential functionality, providing possible applications as an additive in nutritional supplements and medical diets.
REFERENCES
- Chel-Guerrero , L. , Pérez-Flores , V. , Betancur-Ancona , D. and Dávila-Ortiz , G. 2002 . Functional properties of flours and protein isolates from Phaseolus lunatus and Canavalia ensiformis seeds . Journal of Agricultural and Food Chemistry , 50 : 584 – 590 .
- Cai , R. and Hettiarachchy , N.S. 2003 . Jalaluddin, M. High-performance liquid chromatography determination of phenolic constituents in 17 varieties of cowpeas . Journal of Agricultural and Food Chemistry , 51 ( 6 ) : 1623 – 1627 .
- Mune Mune , M.A. , Rene Minká , S. and Lape Mbome , I. 2008 . Response surface methodology for optimization of protein concentrate preparation from cowpea [Vigna unguiculata (L.) walp] . Food Chemistry , 110 : 735 – 741 .
- Neto , V.Q. , Narain , N. , Silva , J.B. and Bora , P.S. 2001 . Functional properties of raw and heat processed cashew nut (Anarcardium occidentalis, L.) Kernel protein isolate . Nahrung Food , 45 : 258 – 262 .
- Van der Ven , C. , Gruppen , H. , de Bont , D.B.A. and Voragen , A.G.J. 2001 . Emulsion properties of casein and whey protein hydrolysates and the relation with other hydrolysate characteristics . Journal of Agricultural and Food Chemistry , 49 : 5005 – 5012 .
- Manninen , A. 2004 . Protein hydrolysates in sports and exercise: A brief review . Journal of Sports Science and Medicine , 3 : 60 – 63 .
- Kristinsson , H.G. and Rasco , B.A. 2000 . Biochemical and functional properties of Atlantic salmon (Salmo salar) muscle proteins hydrolyzed with various alkaline proteases . Journal of Agricultural and Food Chemistry , 48 ( 3 ) : 657 – 666 .
- Gauthier , S.F. and Pouliot , Y. 2003 . Functional and biological properties of peptides obtained by enzymatic hydrolysis of whey proteins . Journal of Dairy Science , 86 ( E suppl ) : E78 – E87 .
- Vioque , J. , Pedroche , J. , Yust , M. , Lqari , M. , Megías , C. , Girón-Calle , J. , Alaiz , M. and Millan , F. 2006 . Bioactive peptides in storage plants proteins . Brazilian Journal of Food Technology , 3 : 99 – 102 .
- Tardioli , P.W. , Pedroche , J. , Giordano , R.L.C. , Fernández-Lapuentes , R. and Guisán , J.M. 2003 . Hydrolysis of proteins by immobilized-stabilized alcalase-glycoxyl agarose . Biotechnology Progress , 19 ( 2 ) : 352 – 360 .
- Betancur-Ancona , D. , Matínez-Rosado , R. , Corona-Cruz , A. , Castellanos-Ruelas , A. , Jaramillo-Flores , M.E. and Chel-Guerrero , L. 2009 . Functional properties of hydrolysates from Phaseolus lunatus seeds . International Journal of Food Science and Technology , 44 : 128 – 137 .
- Doucet , D. , Otter , D.E. , Gauthier , S.F. and Foegeding , E.A. 2003 . Enzyme-induced gelation of extensively hydrolyzed whey proteins by Alcalase: Peptide identification and determination of enzyme specificity . Journal of Agricultural and Food Chemistry , 51 : 6300 – 6308 .
- Pedroche , J. , Yust , M.M. , Girón-Calle , J. , Alaiz , M. , Millán , F. and Vioque , J. 2002 . Utilization of chickpea protein isolates for the production of peptides with angiotensina I converting enzyme inhibitory activity . Journal of the Science and Food and Agriculture , 82 : 960 – 965 .
- Betancur-Ancona , D. , Gallegos-Tintoré , S. and Chel-Guerrero , L. 2004 . Wet-fractionation of Phaseolus lunatus seeds: Partial characterization of starch and protein . Journal of the Science and Food and Agriculture , 84 ( 10 ) : 1193 – 1201 .
- Hamada , J.S. 2000 . Characterization and functional properties of rice bran proteins modified by commercial exoproteases and endoproteases . Journal of Food Science , 65 ( 2 ) : 305 – 310 .
- Kim , Y.S. , Park , W.S.P. and Rhee , C.K. 1990 . Functional properties of proteolytic enzyme modified soy protein isolate . Journal of Agricultural and Food Chemistry , 38 ( 3 ) : 651 – 656 .
- Association of Official Analytical Chemists (AOAC) . 1990 . Methods of Analysis of Association of Official Analytical Chemists , 16th , Washington , D.C : Association of Official Analytical Chemists .
- Were , L. , Hettiarachchy , L. and Kalapathy , U. 1997 . Modified soy proteins with improved foaming and water hidratation properties . Journal of Food Science , 62 ( 4 ) : 821 – 824 .
- Kato , A. and Nakai , S. 1980 . Hydrophobicity determined by a fluorescence probe method and its correlation with surface properties of proteins . Biochemistry and Biophysics Acta , 624 : 13 – 20 .
- Schägger , H. and Jagow , G.V. 1987 . Tricine-sodium dodecyl sulfate-polyacrylamide gel electrophoresis for the separation of proteins in the range from 1 to 100 kDa . Analytical Biochemistry , 166 : 368 – 379 .
- Calderón de la Barca , M.A. , Medrano , A.W. , Jara-Marini , E.M. , González , C.A.F. and Ruiz , S.A. 2000 . Enzymatic modification of functional, nutritional and sensory properties of soy for special feeding . Archivos Latinoamericanos de Nutrición , 50 ( 1 ) : 26 – 34 .
- Friedman , M. 1996 . Nutritional value of proteins from different food sources . A review. Journal of Agricultural and Food Chemistry , 44 ( 1 ) : 6 – 29 .
- Montgomery , D. 2004 . Designs and Analysis of Experiments Limusa-Wiley , México, D.F
- Adler-Nissen , J. 1986 . “ Some fundamental aspects of food protein hydrolysis ” . In Enzymatic Hydrolysis of Food Proteins , Edited by: Adler Nissen , J. 9 – 24 . London : Elsevier Applied Science .
- Villanueva , A. , Vioque , J. , Sánchez-Vioque , R. , Clemente , A. , Pedroche , J. , Bautista , J. and Millán , F. 1999 . Peptide characteristics of sunflower protein hydrolysates . Journal of the American Oil Chemists' Society , 76 ( 12 ) : 1455 – 1460 .
- Vioque , J. , Sánchez-Vioque , R. , Clemente , A. , Pedoche , J. , Bautista , J. and Millan , F. 1999 . Production and characterization of an extensive rapeseed protein hydrolysate . Journal of the American Oil Chemists' Society , 76 ( 7 ) : 819 – 823 .
- Pedroche , J. , Yust , M.M. , Girón-Calle , J. , Alaiz , M. , Millán , F. and Vioque , J. 2002 . Utilization of chickpea protein isolates for the production of peptides with angiotensina I converting enzyme inhibitory activity . Journal of the Science and Food and Agriculture , 82 : 960 – 965 .
- Hong , L.G. , Wei , L.G. , Liu , H. and Hui , S.Y. 2005 . Mung-bean protein hydrolysates obtained with alcalase exhibit angiotensin I-converting enzyme inhibitory activity . Food Science Technology International , 11 ( 4 ) : 281 – 287 .
- Betancur-Ancona , D. , Martínez-Rosado , R. , Corona-Cruz , A. , Castellanos-Ruelas , A. , Jaramillo-Flores , M.E. and Chel-Guerrero , L. 2009 . Functional properties of hydrolysates from Phaseolus lunatus sedes . International Journal of Food Science and Technology , 44 : 128 – 137 .
- Wasswa , J. , Tang , J. and Gu , X. 2008 . Functional properties of grass carp (Ctenopharyngodon idella), nile perch (Lates niloticus) and nile tilapia (Oreochromis niloticus) skin hydrolysates . International Journal of Food Properties , 11 : 339 – 350 .
- Miñones , C.J. , Yust , E.M. , Pedroche , J.J. , Millán , R.F. and Rodríguez , P.J.M. 2005 . Effect of enzymatic treatment of extracted sunflower proteins on solubility, amino acid composition, and surface activity . Journal of Agricultural of Food Chemistry , 53 : 8038 – 8045 .
- Hettiarachchy , N.S. and Kalapathy , U. 1997 . Solubility and emulsifying properties of soy protein isolates modified by pancreatin . Journal of Food Science , 62 ( 6 ) : 1110 – 1115 .
- Tardioli , P.W. , Pedroche , J. , Giordano , R.L.C. , Fernández-Lapuentes , R. and Guisán , J.M. 2003 . Hydrolysis of proteins by immobilized-stabilized alcalase-glycoxyl agarose . Biotechnology Progress , 19 ( 2 ) : 352 – 360 .
- Damodaran , S. and Ziegler , G.R. 1994 . “ Structure-function relationship of food proteins ” . In Protein Functionality in Food Systems , Edited by: Hettiarachchy , N.S. 5 – 6 . New York : Marcel Dekker, Inc .
- Bandyopadhyay , K. and Ghosh , S. 2002 . Preparation and characterization of papain-modified sesame (Sesamum indicum L.) protein isolates . Journal of Agricultural and Food Chemistry , 50 ( 23 ) : 6854 – 6857 .
- Clemente , A. , Vioque , J. and Millán , F. 1999 . Vegetable protein hydrolysates. Department of Physiology and Technology of Vegetable Products. Institute of the Fat , 2 ( 6 ) : 25 – 32 .