Abstract
In this study, the drying kinetics and the effective water diffusivity Deff of tomato halves during oven drying were investigated. This study was carried out for three drying temperatures (45, 55, and 65°C) and for salted and non-salted samples. The Logarithmic model was chosen to describe the drying curves among seven single-layer drying models. The estimated drying parameters of the model were correlated with the drying temperatures using an Arrhenius type equation. The relaxation time ratio of the salted to non-salted tomato halves was 0.80 ± 0.08. The Deff values of tomato halves (salted and non-salted) were estimated with the method of slopes adopting an analytical solution of modified Fick's law. The estimated mean Deff values for non-salted tomato halves was 1.1 × 10-10, 1.9 × 10-10, and 3.2 × 10-10 m2 s-1 and for salted tomato halves was 1.3 × 10-10, 1.9 × 10-10, and 4.0 × 10-10 m2 s-1 at 45, 55 and 65°C considering the shrinkage effect. The estimated activation energy Ea of the salted tomato halves (56.3 kJ mol-1) was found to be approximately 20% higher than this of the non-salted tomato halves (46.8 kJ mol-1) and both were higher from cited Ea in the literature.
INTRODUCTION
Dried tomato (Solanum lycopersicum, Solanaceae) is considered as a delicatessen food adopted in many national cuisines. In the last decade, it has been promoted as an ingredient of fresh green salads or recipes. The tested tomato variety has been perfectly adopted in the arid cultivated areas of Santorini Island in Greece and is of small size, bigger than cherry tomatoes but smaller than the common edible tomato (). It is usually consumed as sun dried or as tomato paste and it is considered as appropriate for drying due to low moisture content, high dry matter content, and high total soluble solids (Brix %) compared to organically cultivated tomatoes.
Figure 1 Whole tomatoes (left) and flesh view (right) of the tested variety. (Colour figure available online.)
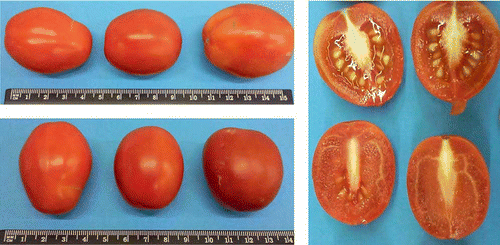
Studies have been carried out in air drying of tomato halves,[Citation1–4] of tomato slices,[Citation5,Citation6] and on sun drying.[Citation7,Citation8] In all studies, the effective diffusion coefficient Deff was estimated employing an analytical solution of Fick's law for a slab geometry without considering the shrinkage of the drying product except in the study of Hossain and Gottschalk[Citation4] who estimated the shrinkage measuring the initial and final thickness of the drying tomato halves.
The aim of this study was to investigate the drying behaviour of tomato. Therefore, three drying temperatures (45, 55, 65°C) and salted against non-salted tomato halves were tested. Seven single-layer drying models were tested for their efficiency to describe the drying curves of the salted and non-salted tomato halves. The parameters of the best model from the tested drying cases were correlated with the three drying temperatures adopting an Arrhenius type equation. Finally, the Deff was estimated considering the volume shrinkage of the samples. For this purpose, a modified Fick's law expression was solved analytically for a slab geometry for the salted and non-salted tomato halves at the three drying temperatures.
THEORY
The moisture ratio MR = Mt/Mo of tomato halves was calculated from the respective experimental drying curves, Mt = f(t) where Mt is the moisture content in dry basis at time t and t is the drying time in h, and fitted to seven single-layer drying models[Citation9–14] tabulated in . The tested drying models have been used in our previous studies[Citation15–18] for other agricultural products. The drying process, as it is demonstrated from the respective drying rate curves in , was taken place in the falling rate period.
Table 1 Single-layer drying models
The drying models in are semi-empirical incorporating the assumption that the resistance of water diffusion occurs mainly in the outer layer of the drying produce.[Citation9,Citation19] These models have been proved to describe well the falling rate period of many foodstuffs during drying.[Citation14,Citation15,Citation18,Citation20,Citation21] Nonlinear regression analysis of the experimental drying data was carried out by Statgraphics Plus 5.1[Citation22] using the Marquardt iterative algorithm. The seven single-layer drying models were assessed for their fitting efficiency based on the adjusted coefficient of determination (), the standard error of estimate (SSE), and the chi-square (χ2). The effect of the three drying temperatures on the drying constant k of the chosen single-layer drying model was determined employing an Arrhenius type equation. The seven tested drying models were also assessed according to four statistical hypotheses concerning the calculated studentized residuals (ei
= MRexp
− MRpred
) of the regressed model.
i. | E(ei ) = 0, an efficient model generates zero residuals, therefore, the smaller the estimated residuals the more efficient the model. The hypothesis can be tested using the mean absolute error (MAE) or other error expressions. | ||||
ii. | var(ei ) = σ2, homoscedasticity hypothesis is the condition where the variance σ2 of the residuals is constant over the entire range of the residuals. This hypothesis can be tested visually plotting the residuals with the predicted values. The residuals must exhibit a non systematic pattern and should be allocated around zero in a narrow value band. | ||||
iii. | cov(ei , ej ) = 0 for every i ≠ j (adjacent measurements), autocorrelation hypothesis is tested either visually, plotting the autocorrelation plot, or numerically, employing Durbin–Watson test. The plot contains a pair of lines (confidence limits) and vertical bars that represent the coefficient for each lag. The distance from the baseline is multiple of the standard error at each lag. Significant autocorrelations extend above or below the confidence limits. | ||||
iv. |
|
The Deff of tomato halves was estimated using the method of slopes,[Citation24,Citation25] which is based on Fick's law of diffusion. The Fick's law in Cartesian coordinates (x-direction diffusion) is given by EquationEq. (1) accompanied by the appropriate initial and boundary conditions:
The analytical solution of EquationEq. (1) is presented in EquationEq. (3).[Citation26] EquationEquation (3) can be used for Deff estimation only if the drying curves MR = f(t) are linear when are plotted in a semi-logarithmic scale. In other cases, the Deff can be estimated either by the method of slopes[Citation24,Citation25,Citation27] or using a numerical scheme to solve EquationEq. (1).
where is the Fourier number, Deff
is the effective diffusivity (m2 s−1), L is the half-thickness of tomato halves (m), and n is the number of terms taken into consideration. In this study, a modified form of EquationEq. (3) derived from Efremov and Kudra,[Citation28] EquationEq. (4), was employed due to accuracy reasons as it was explained in detail by Xanthopoulos et al.:[Citation17]
where a′ = 0.91 for a plane sheet. The characteristic dimension, 2L, was calculated from the volumetric method of water displacement presented by Mohsenin.[Citation29] The estimated thickness of the drying samples (2L) was the radius of the tomato hemispheres whose volume was estimated using the water displacement method. Afterwards, the theoretical MR curves were estimated numerically for a range of Fo numbers using EquationEq. (4). The derived curves for the experimental and the theoretical MR values were plotted against the drying time (t) and Fo numbers, respectively, on a semi-logarithmic plot as it is shown in for the six drying cases (three drying temperatures for non-salted and for salted tomato halves). Finally, the Deff values were estimated from EquationEq. (5) for all moisture contents and drying cases:
The Deff was assumed to remain constant for short successive drying time intervals. From the previous analysis, the Deff values were estimated as function of the drying temperature T employing an Arrhenius type equation.
MATERIALS AND METHODS
Experimental Facility
The drying of the tomato halves was carried out in a laboratory forced convection drying oven, Binder FD 53 (BINDER GmbH, Tuttlingen, Germany). The inner dimensions of the oven are 400 × 400 × 330 mm and its volume is 53 L (). The oven can take up to five racks. At the rear side of the oven, an air fan homogenises the inner drying air temperature field. The latter configuration retains a constant heat transfer rate at produce surface. As it is shown in , two air temperature and humidity sensors, Hobo U10-003 (Onset Computer Corp., Bourne, MA, USA) were used to monitor the inner air temperature and relative humidity, with resolution 0.4°C and 0.5%, and accuracy ±0.7°C and ±3.0% for temperature and relative humidity measurement. The air temperature fluctuation in the drying chamber was <1°C. Openings at the rear side of the oven permit approximately 40–50 changes of air per hour of operation preventing accumulation of air humidity in the drying chamber. The experiments were conducted using fresh tomatoes shipped within 12 h from their harvest site, the island of Santorini in Greece, to the laboratory at Agricultural University of Athens in Greece during the harvest season of summer 2009. Tomatoes were carefully hand-picked based on their uniform physiological state and dimensions. Tomatoes were shipped in two lots, on 7/14/2009 and on 7/29/2009. In order to evaluate the physiological state of the raw material, 30 tomatoes from each lot were randomly picked. In 20 of them, the mass in g, the volume in cm3, and the peel colour in CIELAB scale were measured. In 10 of them, the flesh appearance at their equatorial section, the Brix %, and the acidity % were also measured. The peel colour was measured by a Minolta CR–300 Chromameter (Minolta Co., Tokyo, Japan) in L* (luminance), a* (red-green), and b* (yellow-blue) chromatic coordinates. The Brix degrees (gtss /100 ml) were measured by a temperature-adjusted digital refractometer SR–400 (Erma, Tokyo, Japan) having an accuracy of ±0.25%. The tomato acidity (gacid /100 ml) was measured in terms of citric acid, by the GMK–708 (G–Won Hitech Co., Ltd., Seoul, Korea), with accuracy of ±0.05%.
Figure 4 Forced convection drying oven (BINDER GmbH, Tuttlingen, Germany). In A and B, points were suspended to temperature and relative humidity sensors.
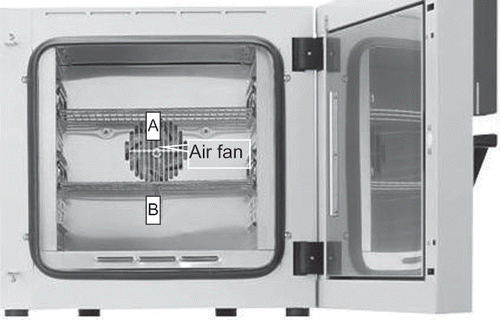
From the mass and volume measurements of whole tomatoes, a simple linear relation was derived (EquationEq. 6), having = 0.987 and SSE = 0.660. The tomato mass with the respective volume from the 20 samples (two lots) are presented in
Figure 5 Volume variation of whole tomatoes with mass (points = experimental values, solid line = estimated values from EquationEq. 6).
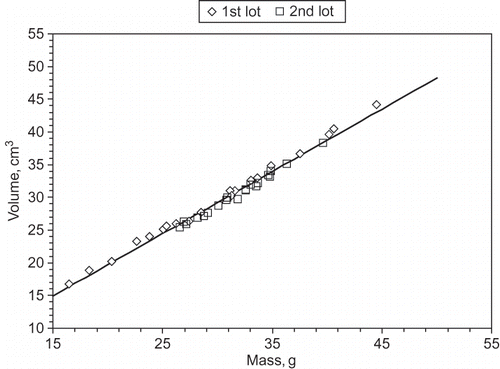
where V is the volume of whole tomatoes in cm3 and Mass is the respective initial weight in g. The tomato flesh appearance was evaluated based on a scale proposed by Kader and Morris.[Citation30] The measured characteristics of the raw material in both lots are presented in . The drying experiments were carried out with tomato halves. Three drying temperatures were tested: 45, 55, and 65°C. In the traditional sun-drying, the tomato halves are treated with salt before drying. The effect of salt on tomato halves drying was evaluated against non-salted tomato halves. For this purpose, 0.3 g of salt (corresponds to a pinch of salt) was spread on each of the 14 samples. In each experiment, 10 tomato halves for weighing and 4 for volume estimation were used. The tomato halves, 28 in total, (14 non-salted and 14 salted tomato halves) were carefully spread on trays prior to their entrance in the drying oven. The initial volume (half) of the four tomato halves was estimated from EquationEq. (6) and during drying with the water displacement method.
Table 2 Measured characteristics of whole tomatoes
The mean initial water content of the tomato halves was 15 ± 2 [93.8 ± 0.6% w.b.] for non-salted tomatoes and 15 ± 3
for salted tomatoes [93.7 ± 1.2% w.b.] (P ≤ 0.05). The drying process was terminated when steady weight of the drying samples was reached [26.1 ± 0.8% w.b. non-salted and 27.5 ± 1.0% w.b. salted tomatoes (P ≤ 0.05)].
The weight of the drying samples was manually measured in regular intervals using an electronic balance of ±0.02 g accuracy, 0.01 g resolution, and 0.4 kg of maximum capacity. The dry matter of the samples was determined at 105°C until steady weight.[Citation31] The volumetric method was based on a platform scale set-up as presented by Mohsenin.[Citation29] The volumetric process must not exceed a few seconds to prevent water absorption from the drying samples. The specific platform scale set-up helps the user to carry out fast volume measurements.
RESULTS AND DISCUSSION
Estimation of the Shrinkage during the Drying of Tomato Halves
Most of the agricultural products undergo extensive shrinkage and surface deformation during drying.[Citation32] In this context, volumetric analysis is the most efficient to describe the true shrinkage of highly deformable samples. The volume and the respective moisture content of the tomato halves were measured during drying and were plotted in Statistical analysis showed that a simple linear model, EquationEq. (7), can efficiently describe the shrinkage of tomato halves. This is consistent with Saravacos and Maroulis's[Citation33] discussion about the correlation of shrinkage with moisture content during drying.
where V is the volume of the tomato halves in cm3 and Mt
is the respective moisture content in . The values of a and b are tabulated in with the respective
values. Although a single linear model can be derived for all the drying cases, for accuracy reasons, a different linear model was derived for each of the drying cases.
Table 3 Parameters of EquationEq. (6) for the different drying cases
Estimation of the Drying Constants for Non-Salted and Salted Tomato Halves
The estimated MR curves were regressed against the seven single-layer drying models of . The derived drying models were sorted in descending order of and ascending of SEE and χ2. Two of the tested models had the best fitting efficiency, the Logarithmic and the Page models. From these two, the Logarithmic model was chosen since the final overall model incorporating the drying temperature effect had better fitting efficiency compared to the Page model. The a, c, and k parameters of the Logarithmic model for all the drying cases with the respective statistical coefficients
, SEE, and χ2 are tabulated in . In , the relaxation times are also tabulated, which according to Karathanos,[Citation14] are times (in s or h) in which the corresponding effect described by the solution of the general drying differential equation is decreased by 63.2%. The mean relaxation time ratio of the salted to non-salted tomato halves is 0.80 ± 0.08, which means that treating tomato halves with salt prior to the drying process will decrease the theoretical drying time by an average of 20% compared to the non-salted tomato halves under ideal drying conditions. As we have referred in our previous study,[Citation18] this type of conclusions must be treated cautiously since errors originated from the regression process may alter the relaxation time to some extent. In , some recent studies on tomato drying of various varieties, size, and geometry (halves or slices) are presented. From the tabulated data, it can be observed that the Logarithmic and the Page models have been proved to describe efficiently the single-layer drying of tomato.
Table 4 Regression analysis coefficients of the Logarithmic drying model
Table 5 Single-layer drying models that have been used for tomato drying
The effect of the drying temperature on the parameters of the Logarithmic model a, k, and c was determined. EquationEqs. (8a)–Equation(8c) refer to the Logarithmic model parameters for the non-salted tomato halves and EquationEqs. (8d)–Equation(8f) for the salted tomato halves.
where R is the universal gas constant (8.31 J mol−1 K−1) and T the air-drying temperature in °C. The experimental and the respective predicted MR values (EquationEqs. 8a–8f) for the three drying temperatures and the non-salted and salted tomato halves are presented in Statistical analysis of the overall drying model gave and RMSE for non-salted tomato halves 0.998 and 1.25% and for salted tomato halves 0.998 and 1.34%, respectively.
Figure 7 Drying curves of tomato halves (points = experimental values, solid lines = theoretical values).
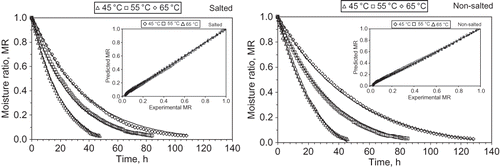
The calculated k values shown in for non-salted and salted tomato halves, as well the respective k values predicted from EquationEqs. (8a) and Equation(8d), are shown in The statistical t-test showed that a significant difference exists between the mean predicted k values for non-salted and salted tomato halves since P = 0.00036 < 0.05.
Figure 8 Experimental and predicted k values from EquationEqs. (8a) and Equation(8d).
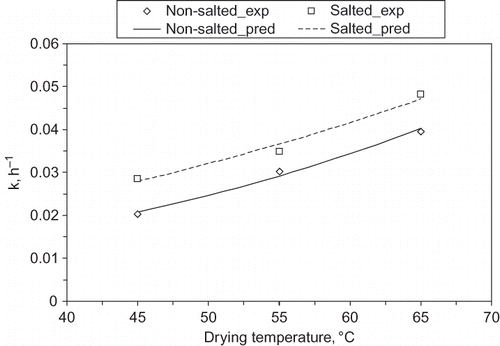
The Logarithmic model was also tested for the four hypotheses presented in the “Theory” section. The testing procedure was based on a visual evaluation of the relative for all the drying cases. The first hypothesis was fulfilled since the calculated MAE was very low, between 0.002–0.008. The normality test showed that all the estimated residuals followed a normal distribution. The homoscedasticity hypothesis was also fulfilled since in all the drying cases, the estimated studentized residuals allocated in a narrow value band in which the worst case ranged between −3.1−4.9 showing no systematic patterns. Finally, the autocorrelation hypothesis showed that overall no significant autocorrelation (P ≤ 0.05) was identified within the estimated studentized residuals. The choice of the Logarithmic model was based on the principle of the “least bad scenario” since it is difficult to have all the hypotheses fulfilled to the same extent. For example, the case of the salted tomato halves at 65°C performed well for the first three hypotheses and to a lesser degree for the normality test.
Estimation of the Effective Water Diffusivity for Non-Salted and Salted Tomato Halves
The Deff was estimated based on the method of slopes presented in the “Theory” section. The analytical solution of Fick's law for a slab geometry was used. The calculated mean Deff values are tabulated in together with Deff values from the literature. The Deff was calculated considering constant and varied sample thickness due to shrinkage effect. The calculated Deff values given in are in the same order of magnitude with the other cited Deff values, except for those given by Kingsly et al.[Citation6] and they lie within the range of 10−11–10-09 m2 s−1 for food stuff given by Saravacos and Maroulis.[Citation33]
Table 6 Estimated Def f values for tomato drying
The multifactor analysis of variance of Deff for the three drying temperatures, the two treatments (salted and non-salted), and the shrinkage effect showed that two of the factors (temperature and shrinkage effect) and their interactions (temperature × shrinkage, temperature × treatment × shrinkage) influenced Deff significantly at P ≤ 0.05. If shrinkage is taken into account, the calculated Deff values are 2–3 times lower than the respective Deff values without considering shrinkage.
Applying the Duncan[Citation23] multiple comparison test, statistically significant differences at P ≤ 0.05 were found in-between the mean Deff values for the different drying temperatures but not between the salted and non-salted cases. Although the Deff values of salted tomato halves lie higher than the respective of non-salted, as one can see in and , this difference is not statistically significant. Probably more salt was needed to further enhance the dehydration rate due to the osmotic effect of salt. The mean Deff values for all the drying cases (with and without shrinkage) are presented in where the Deff values considering no shrinkage lie higher than the respective ones with shrinkage.
Figure 9 Experimental (points) and predicted (dotted line: salted; continuous line: non-salted) Deff values for tomato halves with and without the shrinkage effect.
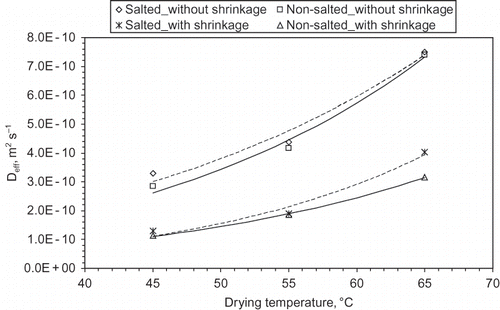
Based on the analysis of variance (P ≤ 0.05) and Saravacos,[Citation36] who has referred that Deff
depends mainly on the porosity and the drying temperature, while moisture content has a minor effect and any dependency of the Deff
from the moisture content is probably due to indirect effect of the porosity, which is strongly affected by the moisture content, the Deff
was assumed to vary only with drying temperatures. The drying temperatures were correlated using non linear regression with the mean Deff
employing an Arrhenius type equation. From the regression of the mean Deff
and the corresponding drying temperatures, EquationEq. (9) for non-salted tomato halves and EquationEq. (10) for salted tomato halves were derived with and SEE for salted tomatoes 0.950 and 3.18.10−11 and for non-salted tomatoes 0.997 and 5.83 10−12, respectively.
where R is the universal gas constant (8.31 J.mol−1 K−1) and T the drying temperature in °C. Similarly, the Ea was estimated for the salted and non-salted tomato halves. The Ea values in both cases with and without shrinkage are tabulated in . The Ea values calculated in this study are higher than the other cited values in the literature, which shows that the energy barrier for diffusion for the specific tomato variety is higher, probably due to high soluble solids concentration.
CONCLUSIONS
The drying behaviour of tomato halves with and without salt treatment was studied for three drying temperatures (45, 55, and 65°C). Seven single-layer drying models were tested in the study of the drying kinetics in both cases. The Logarithmic model was chosen as the best one to describe drying of salted and non-salted tomato halves based on model fitting efficiency and the fulfilment of the four statistical hypotheses. The drying constants and the relaxation times were estimated and presented. The relaxation time ratio of the salted to non-salted tomato halves was 0.80 ± 0.08. The estimated k values were correlated with the respective drying temperatures employing an Arrhenius type equation. The Deff values of salted and non-salted tomato halves were estimated using the method of slopes to tackle the non-linearity of the semi-logarithmic experimental MR curves. The estimated mean Deff values for non-salted tomato halves was 1.14 × 10−10, 1.85 × 10−10, and 3.16 × 10−10 m2 s−1 and for salted tomato halves it was 1.31 × 10−10, 1.90 × 10−10, and 4.02 × 10−10 m2 s−1 at 45, 55, and 65°C considering the shrinkage effect. The estimated Ea of the salted tomato halves (56.34 kJ mol−1) was found to be 20% times higher than that of the non–salted tomato halves (46.83 kJ mol−1).
NOMENCLATURE
Symbols
a, c | = |
Parameters of the Logarithmic model |
a′ | = |
Correction factor, EquationEq. (4) |
D | = |
Water diffusion coefficient (m2 s−1) |
Do , ko | = |
Arrhenius factors (m2 s−1) |
Ea | = |
Energy of activation (J mol−1) |
Fo | = |
Fourier number |
k | = |
Drying constant (h−1) |
M | = |
Water content |
MR | = |
Moisture ratio |
n | = |
Number of terms taken into consideration |
P | = |
Statistical significance level |
L | = |
Half-thickness of tomato halves (m) |
R | = |
Universal gas constant (8.31 J.mol−1 K−1) |
RMSE | = |
Root mean square error (%) |
MAE | = |
Mean absolute error (residuals) |
χ2 | = |
Chi-square |
t | = |
Drying time (in h or s) |
| = |
Coefficient of determination adjusted for the d.f. |
SEE | = |
Standard error of estimate |
cov | = |
Covariance |
T | = |
Drying air temperature (°C) |
DM | = |
Dry matter (g) |
V | = |
Tomato volume (cm3) |
Mass | = |
Initial fresh weight of whole tomato (g) |
E(ei ) | = |
Estimated residual, E(MRexp − MRpred ) |
x | = |
Spatial distance |
Indices
e | = |
Equilibrium moisture content |
o | = |
Initial |
tss | = |
Total soluble solids |
t | = |
Time |
eff | = |
Effective |
ACKNOWLEDGMENTS
The authors would like to thank the president of the general board of directors, Mr. Markos Kafouros, and the general manager, Mr. Dimopoulos Matthaios, of the Union of Santorini Cooperatives (SantoWines) for providing us consistently with fresh tomatoes throughout the experiments. Also, thanks go to Dr. J. L. Woods from the University of Newcastle upon Tyne, UK for his useful suggestions.
REFERENCES
- Giovanelli , G. , Zanoni , B. , Lavelli , V. and Nani , R. 2002 . Water sorption, drying and antioxidant properties of dried tomato products . Journal of Food Engineering , 52 : 135 – 141 .
- Doymaz , I. 2007 . Air-drying characteristics of tomatoes . Journal of Food Engineering , 78 : 1291 – 1297 .
- Sacilik , K. 2007 . The thin-layer modelling of tomato drying process . Agriculturae Conspectus Scientificus , 72 ( 4 ) : 343 – 349 .
- Hossain , M.A. and Gottschalk , K. 2009 . Single layer drying characteristics of half fruit tomato . International Journal of Food Properties , 12 ( 4 ) : 871 – 884 .
- Akanbi , C.T. , Adeyemi , R.S. and Ojo , A. 2006 . Drying characteristics and sorption isotherm of tomato slices . Journal of Food Engineering , 73 : 157 – 163 .
- Kingsly , A.R.P. , Singh , R. , Goyal , R.K. and Singh , D.B. 2007 . Thin-layer drying behaviour of organically produced tomato . American Journal of Food Technology , 2 ( 2 ) : 71 – 78 .
- Sacilik , K. , Keskin , R. and Elicin , A.K. 2006 . Mathematical modelling of solar tunnel drying of thin layer organic tomato . Journal of Food Engineering , 73 : 231 – 238 .
- Rajkumar , P. 2007 . Comparative performance of solar cabinet, vacuum assisted solar and open sun drying methods , Montreal , , Canada : MSc Thesis, McGill University .
- Brooker , D.B. , Bakker-Arkema , F.W. and Hall , C.W. 1992 . Drying and Storage of Grains and Oilseeds , 1st , New York : Van Nostrand Reinhold .
- Zhang , Q. and Litchfield , J.B. 1991 . An optimisation of intermittent corn drying in a laboratory scale thin-layer dryer . Drying Technology , 9 : 383 – 395 .
- Yaldiz , O. and Ertekin , C. 2002 . Thin-layer solar drying of some vegetables . Drying Technology , 19 : 583 – 596 .
- Henderson , S.M. 1974 . Progress in developing the thin-layer drying equation . Transactions of the ASAE , 17 : 1167 – 1172 .
- Wang , C.Y. and Singh , R.P. 1978 . A single layer drying equation for rough rice , 78 – 3001 . St. Joseph, MI , , USA : ASAE Paper No .
- Karathanos , V.T. 1999 . Determination of water content of dried fruits by drying kinetics . Journal of Food Engineering , 39 : 337 – 344 .
- Xanthopoulos , G. , Oikonomou , N. and Lambrinos , G. 2007 . Applicability of a single-layer model to predict the drying rate of whole figs . Journal of Food Engineering , 81 : 553 – 559 .
- Xanthopoulos , G. , Lambrinos , Gr. and Manolopoulou , H. 2007 . Evaluation of thin-layer models for mushroom (Agaricus bisporus) drying . Drying Technology , 25 : 1471 – 1481 .
- Xanthopoulos , G. , Yanniotis , S. and Lambrinos , Gr. 2009 . Water diffusivity and drying kinetics of air drying of figs . Drying Technology , 27 : 502 – 512 .
- Xanthopoulos , G. , Yanniotis , S. and Lambrinos , Gr. 2010 . Study of the drying behaviour in peeled and unpeeled whole figs . Journal of Food Engineering , 97 : 419 – 424 .
- Pabis , S. , Jayas , D.S. and Cenkowski , S. 1998 . Grain Drying: Theory and Practice , 1st , New York : John Wiley & Sons .
- Jayas , D.S. , Cenkowski , S. , Pabis , S. and Muir , W.E. 1991 . Review of thin-layer drying and wetting equations . Drying Technology , 9 ( 3 ) : 551 – 588 .
- Karathanos , V.T. and Belessiotis , V.G. 1999 . Application of a thin-layer equation to drying data of fresh and semi-dried fruits . Journal of Agricultural Engineering Research , 74 : 355 – 361 .
- Statgraphics Plus 5.1 . 2001 . Online Manual. Statistical Graphics Corp , VA , , USA : Statpoint Technologies .
- Mason , R.L. , Gunst , R.F. and Hess , J.L. 2003 . Statistical Design and Analysis of Experiments: with Applications to Engineering and Science , 2nd , New York : Wiley-Interscience .
- Karathanos , V.T. , Villalobos , G. and Saravacos , G.D. 1990 . Comparison of two methods of estimation of the effective water diffusivity from drying data . Journal of Food Science , 55 ( 1 ) : 218 – 223 . 231
- Perry , R.H. and Green , D.W. 1999 . Perry's Chemical Engineers’ Handbook , 8th , New York : McGraw-Hill .
- Rahman , M.D.S. and Lamb , J. 1991 . Air drying behaviour of fresh and osmotically dehydrated pineapple . Journal of Food Process Engineering , 14 : 163 – 171 .
- Crank , J. 1975 . Mathematics of Diffusion , 2nd , London : Oxford University Press .
- Efremov , G. and Kudra , T. 2005 . Model-based estimate for time-dependent apparent diffusivity . Drying Technology , 23 : 2513 – 2522 .
- Mohsenin , N.N. 1978 . Physical Properties of Plant and Animal Materials , 2nd , New York : Gordon and Breach Science Publishers .
- Kader , A.A. and Morris , L.L. 1976 . “ Correlating subjective and objective measurements of maturation and ripeness of tomatoes ” . In Proceedings of 2nd Tomato Quality Workshop , California , , USA : Vegetable Crops Series 178, University of California Davis: Davis .
- AOAC . 1997 . Official Methods of Analysis , 16th , Washington : Association of Official Analytical Chemists .
- Mayor , L. and Sereno , A.M. 2004 . Modelling shrinkage during convective drying of food materials: A review . Journal of Food Engineering , 61 : 373 – 386 .
- Saravacos , G.D. and Maroulis , Z.B. 2001 . Transport Properties of Foods , 1st , New York : Marcel Decker, Inc .
- Page , C. 1949 . Factors influencing the maximum rates of air drying of shelled corn in thin layer , Lafayette , Indiana, USA : MSc Thesis, Purdue University .
- Midilli , A. , Kucuk , H. and Yapar , Z. 2002 . A new model for single layer drying . Drying Technology , 20 : 1503 – 1513 .
- Saravacos , G.D. 2005 . “ Mass transfer properties of foods ” . In Engineering Properties of Foods , Edited by: Rao , M.A. , Rizvi , S.S.H. and Datta , A.K. 327 – 372 . New York : Taylor & Francis .