Abstract
Sweet potato is an important food crop having nutritive value in terms of starch, carotein, and minerals. The acceptability and overall quality of the products depend on their textural and rheological attributes. Cooking quality of the tubers could be related to the textural, pasting, and gelatinization properties, which vary depending on the flesh color of the tubers. Also, being a promising ingredient in many of the traditional and industrial products, the physico-chemical and functional properties of sweet potato flour are very much important for their selection for developing various value added products. The textural, gelatinization, and pasting characteristics of white, cream, and orange fleshed sweet potato tubers were analyzed and their thermal softening behavior was modeled by linear regression and fractional conversion techniques. Significant variations were observed in the properties among different varieties (p < 0.05). Kinetics of texture degradation on cooking were explained by first-order and dual-mechanism first-order models and the latter was found to be more suitable. The first three principal components explained about 85% of the total variation in texture profile parameters, gelatinization, and pasting properties. Though there was no similarity in the textural properties of the raw tubers among the varieties of same flesh color group, the quality of the cooked tubers and flours of different varieties in the same group are similar as revealed by the multivariate analysis.
INTRODUCTION
Sweet potato (Ipomoea batatas L.) is a versatile root crop owing to its wide adaptability to diverse agro climatic conditions, shorter growth period, round the year availability, higher yields with low agronomical inputs, and less field problems.Citation[1] Out of the 697.62 million tons of root and tuber production in the world from an area of 52.70 million hectares, share of sweet potato is 107.67 million tons from 8.10 million hectares, next to potato and cassava. Asia constitutes about 85.33 and 54.44%, respectively in production and area of the total sweet potato, yielding about 20.80 t/ha whereas the world harvest is only 13.29 t/ha.Citation[2] Sweet potato has a higher production efficiency of edible energy per hectare per day and protein yield per hectare.Citation[1]
Sweet potato roots are generally consumed as direct food after boiling, steaming, baking, and frying; processed form as frozen and canned products; as flour or starch, which form ingredients in various traditional foods, confectionaries, etc.; and as raw material for alcohol, sugar syrup or glucose, starch noodles, etc.[Citation1,Citation3–8] Depending upon the flesh color, sweet potato tubers can be classified into white, cream, yellow, orange, and purple colored. Among these, the latter three are of interest in health foods, because β-carotene in the yellow or orange fleshed and anthocyanin in the purple fleshed tubers are considered as dietary antioxidants. Also, yellow or orange fleshed sweet potato is a potential raw material in weaning food preparations as β-carotene helps to alleviate vitamin A deficiency among children below 6 years of age. Also, colored food products, such as flours, flakes, and pastes, can be made from these cultivars.Citation[9,Citation10]
Being a crop that is used as human food after thermal processing, the textural properties are important quality attributes to be studied as they influence the acceptability and overall quality of the product. Depending on the mouth feel and sensory attributes, the cooked tubers can be classified into moist or yam type with soft and syrupy texture and dry type with firm and mealy texture.Citation[1,Citation11,Citation12] The sensory texture assignment of moist type sweet potato is characterized by low shear stress values, whereas dry type sweet potato has high values.Citation[12] Truong et al.Citation[11] found shear stress by uniaxial compression test and fracturability, hardness, and gumminess by texture profile analysis, which correlated highly with both mouth feel and mechanical type sensory notes. Effect of curing and blanching on the firmness of the processed sweet potato was studied by many authors.Citation[11,Citation13,Citation14] Watson and Jarvis,Citation[15] by measuring the firmness of orange and white fleshed sweet potato tubers cooked at 70 and 100°C, found decreased firmness and increased dryness during cooking at 100°C. Although a lot of studies have been conducted on the textural changes of sweet potato tubers associated with boiling/baking, the textural attributes of the raw tubers of varied flesh colors having specific physico-chemical and functional properties and the kinetics of their thermal softening properties during cooking have received little attention.
Sweet potato starch and flour are promising ingredients in many of the traditional food preparations and industrial products and, hence, their physico-chemical and functional properties are very important for their selection for various purposes. Exhaustive literature is available on the physico-chemical properties and pasting properties of sweet potato starch and their genotypic variation.Citation[6,Citation16–21] The pasting properties of 20 new sweet potato lines have been compared with that of Koganesengen, a Japanese leading variety having regular starch properties and reported 10–20°C lower pasting temperature than the former.Citation[7] Jangchud et al.Citation[6] observed a starch pasting temperature of 80.5°C for orange fleshed and 74.8°C for purple fleshed tubers. Genotypic variation of pasting profile as studied by Collado et al.Citation[19] on 44 genotypes showed type A profile at 11% starch concentration and Type C profile at 7% starch concentration. As sweet potato flour is an important ingredient in a formulated food system, its functional and pasting properties also need attention. Physico-chemical properties of sweet potato flour were studied by Osundhahunsi et al.Citation[22] and flour characteristics of sweet potato prepared by different drying techniques by Yadav et al.Citation[23] The literature on the relationship between the pasting properties of sweet potato flour with the cooking quality of the tubers is scanty.
Different sweet potato varieties have been developed in India with a harvesting age of 90–105 days and yield potential ranging from 20.86 to 34.27 tons per hectare.Citation[24,Citation25] But the acceptability of the tubers depends not only on the yield potential but also on the processing quality for which texture and pasting properties assume a significant role. Studies on the textural changes of the raw and cooked tubers in relation to the pasting and gelatinization properties of sweet potato varieties with different flesh colors are sparse. Hence, the present study aims at evaluating the textural properties of the tubers of different flesh colored sweet potato varieties, viz., three white, two each cream and orange. Kinetics of the texture degradation due to cooking was analyzed by a linear regression method and fractional conversion techniques. Classification of the varieties based on the texture of the raw tubers and correlation between texture profile parameters of the cooked tubers, pasting properties, and gelatinization parameters and, thereby, their grouping was also carried out by multivariate analysis.
MATERIALS AND METHODS
Tubers of white fleshed sweet potato, viz., CO3-4, SV 280, SI60; cream, viz., Sree Arun, Sree Varun, and orange, viz., 362-7 and SV 98 harvested at 90 days maturity were used in the study . Fresh tubers free from any external cuts or bruises and weevil attack symptoms were washed with tap water to remove adhering soil, clay, and other foreign materials and kept overnight under ambient conditions to remove the surface moisture.
Figure 1 Different varieties of sweet potato tubers used in the study: (a) 362-7; (b) CO3-4; (c) SI 60; (d) Sree Arun; (e) SV 280; (f) SV 98; (g) Sree Varun. (Color figure available online.)
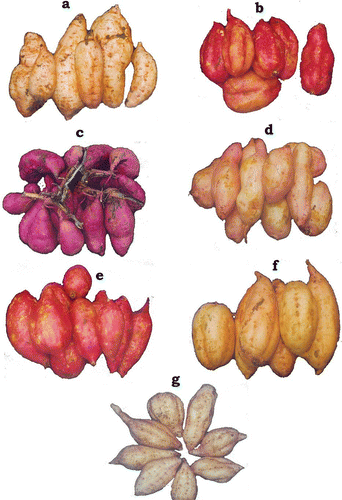
Biochemical Properties
Moisture/dry matter of the tubers was determined by standard methods of AOAC method 7.009.Citation[26] Starch, sugar, and fiber contents were determined by the methods detailed by Padmaja et al.Citation[27]
Instrumental Textural Properties
Textural properties of the tubers were determined using a food texture analyzer (TA HDi-Stable Microsystems, Surrey, UK) with Texture Expert Exceed built in software. Two tests, viz., puncture test and shear/cutting tests were performed where the probe was moved into the tubers up to a specified depth of 20 mm. Puncture tests were carried out using 5 mm cylindrical (P/5) and 2 mm needle (P/2N) probes and cutting tests using a HDP/BS Guillotine blade set (TA HDi-Stable Microsystems). All the tests were performed under the mode measure force in compression with return to start option with a test speed of 2 mm/s. The parameters recorded from the force deformation/time of the puncture test using a 5-mm probe were: firmness—maximum peak force (N), toughness—area under the curve (Ns), and stiffness—slope of the straight line up to the peak; using a 2-mm needle probe: piercing force—maximum peak force (N) and piercing energy—area under the curve (Ns). In the cutting/shearing experiments, the peak force obtained from the graph was taken as shear force (N) and the area under the curve as shear energy (Ns). The measurements were made using 50 tubers of each variety.
The tubers after peeling and washing were cut into 10-mm cubes using a hand slicer, placed in wire mesh baskets, and immersed in an electrically heated digital temperature controlled boiling water bath maintained at 98°C (Remi Equipments, Mumbai, India). Samples were taken out from the water bath at regular intervals of 2.5 min for up to 30 min and were allowed to cool at room temperature (30°C). A puncture test was carried out using a 5-mm stainless steel cylindrical probe at a test speed of 2 mm/s for a distance of 5 mm. Firmness was measured as the maximum peak force. Degree of cooking was measured by the method of Ramesh et al.,Citation[28] assuming 0% for raw and 100% for fully cooked tubers. Relative firmness was calculated as the ratio of the firmness of cooked sample at any time to the firmness of the raw sample.
Instrumental texture profile analysis (TPA) was performed for the sample cooked at 20 min (normal cooking time is 20 min) at a test speed of 1 mm/s for 25% compression with a time lag of 3 s. From the TPA curve, the following parameters were calculated by the method of Bourne:Citation[29] hardness (height of the force peak on the first compression cycle), adhesiveness (negative force area of the first compression cycle), cohesiveness (ratio of the positive force areas under the first and second compression), springiness (distance by which the food recovers its height during the time between the end of the first bite and the start of the second bite), and chewiness (energy required to masticate a food and is the product of hardness, cohesiveness and springiness). For all the tests to analyze the textural properties of cooked tubers, 20 samples were used.
Kinetic Modeling of Texture Degradation Due to Cooking
Cooking is a kinetic process and the textural changes during cooking of plant tissues can be explained by first order or dual order mechanism of a first order kinetic model.Citation[30,Citation31] A simple first order equation for the texture degradation due to cooking at constant temperature is given as:
where Ft = the firmness at heating time ‘t’ min, N; Fo = initial firmness of the product, N; ko = rate constant, min−1. From the plot of Ft /Fo versus cooking time, the exponential trend line is calculated for each variety. By applying fractional conversion techniques to the data on the firmness of tubers for different time intervals, the following equation can be applied for the thermal softening of tubers:
where Fe = firmness at equilibrium or the non zero maximum retainable firmness, N; k 1 = constant and k 2 = rate constant, min−1. The value of (Ft - Fe )/(Fo - Fe ) is plotted against cooking time (graph not shown) and the trend line is calculated with values of k 1 and k 2 and correlation coefficient (r 2).
Gelatinization Properties of Sweet Potato Flour
Sweet potato chips of about 3 mm thickness were prepared by manual slicing and dried at 50°C for 48 h. The dry chips were converted into flour by using a Cyclotec 1093 (Foss Tecator, Hoganas, Sweden) sample mill and used for further analysis. Gelatinization profiles of the flours were determined using a DSCe 822 differential scanning calorimeter (Mettler Toledo Analytical GmbH, Schwerzenbach, Switzerland) equipped with STARe software by the method of Moorthy et al.Citation[32] A weighed quantity of flour (5 μg) was transferred into a previously weighed aluminum pan. Distilled water (10 μl) was added to give a water/flour ratio of 2:1. The pan was sealed hermetically and placed on the DSC sensor in the furnace. An empty aluminum pan was used as reference and indium was used as a standard. The heating rate was 10°C/min. Gelatinization onset (To ), peak (Tp ), and conclusion (Tc ) temperatures and enthalpy of gelatinization (ΔH) were noted from the thermograms. Gelatinization temperature range was calculated as the difference between Tc and To . Peak height index was measured as the ratio of the enthalpy to the difference between Tp and To . Pasting characteristics of the flours were determined with a RVA-4 Rapid Visco Analyzer (New Port Scientific, Warriewood, Australia) at a fixed starch concentration of 10% and a constant speed of 160 rpm using the standard I profile, described by Garcia and Walter.Citation[18] The temperature profile was as follows: heating from 50 to 95°C at 12°C/min, holding at 95°C for 2 min, cooling to 50°C at 12°C/min, and holding at 50°C for 2 min. The values for peak, tough, final, setback, and break down viscosities and pasting temperature were obtained from the viscogram.
Statistical Analysis
The data were analyzed using the statistical package SAS 8.0 (SAS Institute, Inc., Cary, NC, USA) to perform analysis of variance (ANOVA), Duncan Multiple Range Test (DMRT), and correlation coefficient. The mean values obtained from ANOVA were subjected to DMRT for testing pair-wise comparison. Multivariate analysis, viz., cluster analysis and principal component analysis were carried out using SAS 8.0/MATLAB 6.5 (The Mathworks, Inc., Natick, MA, USA). Cluster analysis was done by the complete linkage method and principal component analysis was carried out on the correlation matrix. Simple linear regression models were fitted to study the relationship between degree of cooking and relative hardness of the cooked tubers of different varieties.
RESULTS AND DISCUSSION
Texture of Raw Tubers
The textural variability of the raw tubers of different sweet potato varieties as analyzed by ANOVA and DMRT on mean values for pair-wise comparison is given in and all the textural parameters are significantly different among the varieties (p < 0.05). The minimum value of firmness was recorded by 362-7 and maximum value by CO3-4. However, the values for the cream fleshed varieties (Sree Arun and Sree Varun), white fleshed varieties (SI 60, SV 280), and orange fleshed (SV 98) are statistically on par. The stiffness values ranged from 17.23 N/s for CO3-4 to 22.99 N/s for SI 60. There is no significant difference between the values for the variety CO3-4 and Sree Arun, and between SV 280, Sree Varun, SV 98, 362-7, and SI 60. Toughness, a measure of the energy required to move the probe through a certain distance in the tubers, significantly varied among the varieties with the minimum being 1049 Ns for 362-7 to the maximum being 1452 Ns for CO3-4. Significant difference existed between the varieties 362-7 and Sree Varun, but toughness of Sree Arun, Sree Varun, SV98, and white fleshed SI-60 and SV280, and SV 280 and CO3-4 were observed statistically on par (1334 to 1452 Ns).
Table 1 Textural properties of sweet potato raw tubers
Generally, sweet potato tubers are made into slices or chips by cutting before boiling or dried and milled to fine flour before being used for the preparation of value added products. Hence, cutting/shearing tests help to quantify the cutting force and energy required for the process. Cutting force was at a minimum for SV280 (137.79 N) and maximum for Sree Arun (211.5 N), and significant difference among the varieties existed (P < 0.05). SV280 had a significantly lower value of cutting force than Sree Arun, Sree Varun, SV 98, S160, and CO3-4. However, the values for SV280 and 362-7 are statistically on par and that also is the case for 362-7, SV98, and SI 60. Cutting energy values were also significantly different: maximum for Sree Arun (2733 Ns) and minimum for SV280 (1809 Ns). Here also, values for SV280 and 362-7 and CO3-4 and SI 60 were almost similar.
A puncture test was also performed with a 2-mm needle and the results are represented in terms of piercing force and piercing energy, which indirectly give an indication about the thickness of the peel of the tubers. There was not much variation in the piercing force as the values ranged from 20.13 N for 362-7 to 26.59 N for S160, which shows that the skin of S160 offered more resistance to piercing than 362-7. The piercing force was almost on par for the varieties SV 280, CO3-4, Sree Arun, and Sree Varun. Piercing energy for the variety 362-7 was a minimum of 235.7 Ns, which was significantly different from those of all other varieties. However, for other varieties the values ranged from 255.23 to 272.88 Ns, showing less variability.
The presence of varying amounts of biopolymers, such as starch, cellulose, and pectin, and the cellular and molecular organization of the plant materials, viz., composition of the cell wall, cell size and intercellular spaces, turgor pressure, overall structure and shape of the cell are the main factors attributed for the textural variation of the plant tissue.[Citation33–35] The wide variability in the texture of the sweet potato tubers may be due to the variation in the physico-chemical properties of tubers of different varieties as given in . Moisture content varied from 70.16% for SV280 to 76.77% for Sree Arun and there was a significant difference among varieties (p < 0.05). The starch content was minimum for 362-7 (59.13%) and maximum for SV 280 (68.38%); however, no significant difference in starch content among the varieties in the white flesh colored and in the cream flesh colored varieties. The fiber content was maximum for Sree Arun (2.8%) and minimum for 362-7(1.55%). It is also worth mentioning that all the biochemical constituents studied were statistically different only among the varieties in the orange fleshed varieties, but for the varieties in the other two groups, there was not much variation.
Table 2 Biochemical properties of sweet potato tubers
Texture Profile of Cooked Tubers
Texture profile parameters, viz., hardness, adhesiveness, and cohesiveness, were found to be significantly different among the varieties (p < 0.05), whereas springiness and chewiness were not different significantly (p > 0.05) (). However, among the orange-fleshed varieties, the values were almost the same. Hardness ranged from 9.36 N for Sree Arun to 26.84 N for CO3-4. For the orange-fleshed varieties, the firmness was 16.12 N for 362-7 and 14.76 N for SV98. Adhesiveness was minimum for 362-7 (0.154 Ns) and maximum for SV280 (0.63 Ns). The adhesiveness of SV98, Sree Varun, S160, and CO3-4 were almost similar. The highest and the lowest values of springiness were recorded for the orange fleshed varieties, i.e., 0.864 and 1.046 for 362-7 and SV 98, respectively. Cohesiveness was maximum for 362-7 (0.289) and minimum for CO3-4 (0.218). But for all other varieties, the cohesiveness was statistically on par. Chewiness, the energy required to masticate the product, was minimum for Sree Arun (1.80) and maximum for CO3-4 (5.89). But for all other varieties, it was almost similar. Softening of the tubers due to cooking is attributed to the physico-chemical changes occurring to the cell wall materials, such as reduction in hydrostatic pressure, gelatinization of starch and solubilization of pectic material in the middle lamella, cell separation, and an associated loss of turgor.[Citation36–40] During thermal treatment, starch granules imbibe water and swell to a large extent, leading to the process called gelatinization, which occurs around 70–80°C.Citation[41] This will lead to the expansion of cell size and volume, cell separation, and cell wall distension depending upon the amount of starch content.[Citation42,Citation43] Sweet potato tubers contain heat stable amylases and it is active for a period during cooking until the temperature rises above about 70°C and the starch gelatinizes;Citation[15] however, they are inactivated at higher temperatures. Up to 70°C cooking, starch breaks down to products of low molecular weight, which diffuse through the cell wall after thermal disruption of the plasmalemma; but at 100°C, thermal inactivation of the amylases was clearly rapid enough to prevent any major degradation of starch. Hence, the textural change is mainly due to the cell separation that occurred due to the starch swelling pressure.
Table 3 Texture profile parameters of cooked tubers
Truong et al.Citation[11] studied the relationship between the instrumental and sensory parameters of cooked sweet potato tubers and found that among the TPA parameters, fracturability, hardness, and gumminess could be related with the mealiness of the tubers, the higher the values, the higher the mealiness or dryness. Accordingly, it was found that maximum hardness and gumminess was observed for CO3-4 and minimum for Sree Arun. Hence, based on the above perception, the mealiness of the cooked tubers decreased in the following order: CO3-4 > SI 60 > SV 280 > 362-7 > Sree Varun > SV 98 > Sree Arun. From , similar trends could not be observed for the biochemical constituents of sweet potato tubers, which showed that the textural characteristics of cooked tubers cannot be correlated to any specific component of the tubers but depends on a number of factors, such as quality of the starch, interaction between the components in the tubers, and the structural make up of the tuber tissues.
Kinetics of Thermal Softening
Degree of cooking was found to be increased at an increasing rate in the initial period of cooking and at 20 min itself, the degree of cooking was more than 90% for all the varieties, i.e., 94.89% for 362-7, 96.34% for SV 98, 97.39% for SV 280, 93.98% for SI 60, 96.24% for CO3-4, 95.91% for Sree Varun, and 97.50% for Sree Arun. Degree of cooking with relative firmness was linearly dependent as evidenced from the linear regression equation in the form:
where Y = degree of cooking, x = relative firmness, and A and B are model constants, the values of which are represented in . For each variety, the values of A and B are the same; however, among the varieties, the values ranged from 105.34 for Sree Arun to 111.74 for 362-7. Similar results were also reported for carrot (A = 114.94, B = 115.09) and radish (A = 116.34, B = 116.0) by Ramesh and othersCitation[28] and for cassava tubers of short duration varieties (A = 105.74 to 127.52, B = 105.75 to 127.51) by Sajeev et al.Citation[44] The validity of the model was checked by calculating the modulus values as described by Lamauro and BakshiCitation[45] as:
Table 4 Values of constants in linear model relating degree of cooking and relative firmness of sweet potato tubersFootnote**
where n = number of observations; Fti act = actual value of firmness, N; Ftiprd = predicted value of firmness, N. If the modulus is less than 5, the model shows extremely good fit, between 5–10 reasonably good fit, and >10 is considered as poor fit. Since the modulus values for all the studied varieties are very low (), the linear equation is extremely good fit. However, the variety specific nature of the model constants and their narrow range necessitated the development of a general model for sweet potato tubers without giving weightage to the individual varieties, for which the values of A and B are found to be 108.18 and 107.48. The very small deviation from the general model to the individual variety model, as calculated by the modulus () also indicated that this equation has extremely good fit to predict the degree of cooking and firmness at different times of cooking. A comparison of the observed and predicted values of degree of cooking of different varieties of sweet potato is also given in
Figure 2 Comparison of the observed and predicted values of degree of cooking by the linear regression equations.
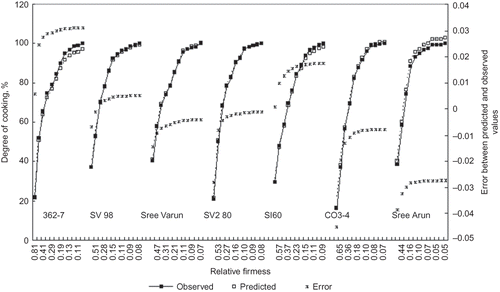
The texture degradation of sweet potato tubers during cooking was modeled by a first order and dual mechanism of first order kinetic model. From the exponential decay curves for firmness or linearized form with respect to cooking time (figures not shown), the trend lines for both models were calculated and the values of the model constants in the equations with corresponding correlation coefficients are given in . The highest values of r 2(>0.90) indicate the suitability of both the models in these cases and a comparison of the observed and predicted values of relative firmness and fractional conversion of firmness with cooking time of different varieties of sweet potato is represented in
Figure 3 Comparison of the observed and predicted values of the kinetic models for the thermal softening of sweet potato tubers.
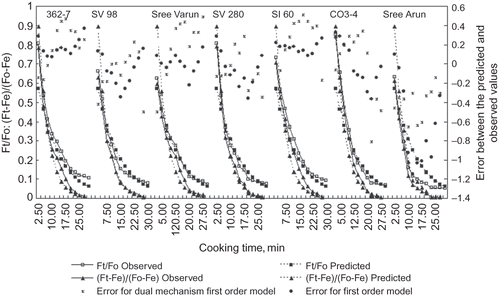
Table 5 Values of constants in the first order and dual mechanism first order kinetic model to explain thermal softening of sweet potato tubers
However, the dual mechanism first order model is considered to be more apt to explain the thermal softening characteristics and the lowest rate constants value was obtained for Sree Varun (0.153) and highest for CO3-4 (0.232). Based on the inverse relationship of rate constant with cooking time as described by Beleia et al.Citation[46,Citation47] and Sajeev et al.,Citation[44] these varieties can be grouped according to the ease of cooking as: CO3-4 > Sree Arun > SV 280 > SV 98 > SI 60 > 362-7 > Sree Varun. Here also the distinct difference could not be observed among the different color fleshed varieties.
Pasting Properties of Sweet Potato Flour
All the viscosity parameters were significantly different among the varieties (P < 0.05) (). Flour from the tubers of Sree Arun recorded the minimum value of trough (407 cP), final (553 cP), and setback (146 cP) viscosities and maximum pasting temperature (82.73°C), whereas peak viscosity was minimum for Sree Varun (617 cP) and breakdown for SI 60 (100 cP). However, CO3-4 recorded the highest value of peak (940 cP), trough (738 cP), final (955 cP), and setback (217 cP) and minimum pasting temperature (79.75°C). Except for the setback viscosity and pasting temperature, the orange fleshed varieties had a significant difference in their values. Setback ratio is used to predict the retrogradation tendency of starchy materials, which did not vary much and ranged from 1.29 to 1.37 only.
Figure 4 Viscometric properties of flours extracted from sweet potato tubers of different varieties. Columns with the same letters for each property are not significantly different (p < 0.05) by Duncan's multiple range test. The vertical line on each column/point represents error bars using standard deviation.
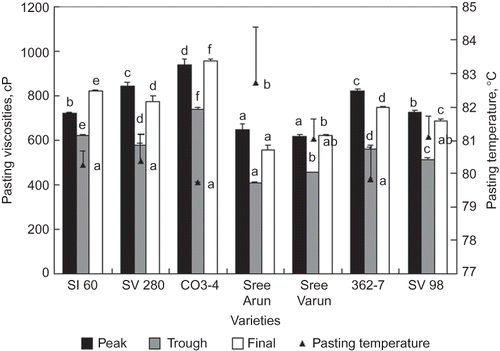
Genotypic variability in pasting properties of sweet potato flour/starch was observed by many authors.Citation[6,Citation7,Citation9,Citation16,Citation17,Citation19,Citation48–51] Differences in the viscometric properties are attributed to the genetic make up and structural variation of the starch present in the tubers. Variation in the associated forces in the starch granules can be responsible for the observed difference in the pasting properties of different varieties.[32] Besides, the varied quantity of starch in the flour also contributed for the difference in pasting properties, but no regular trend between starch content and pasting parameters could be observed. Hence, the contribution of sugar or fiber individually or their combined affect and ratio of the amylose/amylopectin content in the starch may be attributed for the reported changes in pasting properties of sweet potato flour.
The pasting properties can be used as a predictor to differentiate mealy and non mealy varieties. Singh et al.[21] classified the mealy varieties of potato based on their higher values of peak, final, and setback viscosity; lower pasting temperature; and high set back ratio. Variation in final and set back viscosity is in the same order for all the varieties, but not the peak viscosity except for the highest value for CO3-4. Hence, by noting the highest final and set back viscosity, pasting temperature, and set back ratio, the mealiness of the varieties decreased in the order: CO3-4 > SI 60 > SV 280 > 362-7 > SV 98 > Sree Varun > Sree Arun, which shows that mealiness of white fleshed varieties is higher than that of orange fleshed and cream fleshed varieties. This is almost in agreement with the classification of the varieties based on the texture profile parameters, wherein the order of mealiness of CO3-4, S I 60, SV 280, 362-7, and Sree Arun is the same, but a slight deviation for the other two varieties.
Gelatinization Properties of Sweet Potato Flour
Among the DSC gelatinization parameters, Sree Arun recorded the highest values for all the parameters (i.e., To , 82.80°C; Tp , 86.77°C; Te , 92.62°C; ΔH, 9.41J/g), whereas the lowest onset (79.27°C) for 362-7, peak (83.19°C) for SV280, and end set (88.04°C) for CO3-4 and SI60, and enthalpy (7.52J/g) for SV98 (). There was a clear cut difference among the two groups of varieties as far as enthalpies of gelatinization are concerned. For cream fleshed varieties, the values hovered around 9.23 J/g, whereas for orange fleshed varieties, the values were 7.68 and 7.52 J/g. It is worth noting that for orange fleshed varieties, the enthalpy was much lower (mean 7.60 J/g) than that of the white fleshed varieties (9.29 J/g). However, among the two orange fleshed varieties, the values of gelatinization temperature were almost the same except for To , for which the range was 79.27 to 80.15°C.
Figure 5 Gelatinization properties of sweet potato flours from different varieties. Columns with the same letters for each property are not significantly different (p < 0.05) by Duncan's multiple range test. The vertical line on each column/point represents error bars using standard deviation.
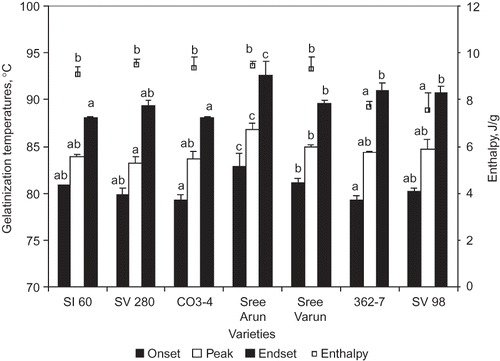
Collado et al.,Citation[19] in their studies on the genetic variation in the physico-chemical properties of sweet potato starch from 44 genotypes, observed a wide variability in the DSC gelatinization parameters and opined that starch differing in gelatinization temperature and enthalpy have different cooking characteristics affecting the individual process. In the present study, the enthalpy of cream fleshed varieties ranged only from 9.02 to 9.41 J/g, which is significantly different from that of orange fleshed sweet potato (7.52–7.62 J/g). However, even though the intra group variation was predominant, within the same group (intergroup), variability was much less. Similar was the case with gelatinization temperature range, for cream fleshed variety, variation was 7.23–9.82°C, whereas for orange fleshed sweet potato, it was 10.6–11.16°C, which shows high intra group variability. The peak height index also showed a less intergroup variation, i.e., for cream fleshed varieties it varied from 2.13 to 2.90 and for orange fleshed sweet potato it varied from 1.5 to 1.6 showing the varied level of uniformity of gelatinization of the starchy flour.
Variations in starch gelatinization properties measured by DSC are also due to the molecular structure of amylopectin within the same botanical origin of sweet potato.Citation[9,Citation20,Citation52] They also reported that the starches with lower To , Tp , and ΔH were shown to have higher content of extremely short chains with DP6 and DP7 in amylopectin molecules, and amylose/amylopectin ratio did not have an impact on DSC parameters. A negative correlation of molar percentage of DP6 and DP7 with To , Tp , and ΔH values was also observed.
Clustering and Principal Component Analysis
Cluster analysis was carried out to group the varieties based on the textural properties of raw tubers. The results obtained by hierarchical clustering is represented by a dendrogram (). At 80% similarity, three groups were obtained: Group I: Sree Arun, CO3-4; Group II: Sree Varun, SI 60, SV 98, and SV 280; and Group III: 362-7. These results showed that no visible difference among different colored varieties could be observed from the texture of the raw tubers. The varieties in Group I have the lowest group mean value of stiffness (17.68 N/s), whereas they have the highest mean value for firmness (97.24 N), toughness (1372 Ns), piercing force (25.0 N), cutting force (201.67 N), and cutting energy (2609 Ns). The variety in Group III (362-7) showed wide variation from the other two clusters, registering the highest group mean value of stiffness (22.93 N/s) and lowest mean values for firmness (75.88 N), toughness (1049 Ns), piercing force (20.13 N), cutting force (154.79 N), and cutting energy (1835 Ns).
Principal component analysis was carried out on the texture profile parameters, pasting properties and DSC gelatinization parameters of the varieties. It was observed that the first three PCs explained variances of about 52.06, 20.11, and 12.83%, respectively, which accounted for about 85% of total variation. The score plot of the varieties based on the first three principal components are drawn and represented in Based on PC1 and PC2, since the varieties CO3-4, SV 280, and SI 60 are located in the third quadrant, they can be grouped together and similar was the case for Sree Arun and Sree Varun, located in the fourth quadrant. Also, SV 98 and 362-7 are in the positive side of the PC 2 and they can be grouped together. Similarly, according to PC1 and PC3, the groupings were SI 60, SV 280, and 362-7; SV 98 and Sree Varun; and Sree Arun and CO3-4. Also, by PC2 and PC3, the varieties can be grouped into SI 60, SV 280, Sree Varun; SV 98 and 362-7; and CO3-4 and Sree Arun. The varieties SV 280 and SI 60 were grouped together in all the score plots. It is worth mentioning that PC1 and PC2 explain about 72.17%, PC2 and PC3 about 32.94%, and PC1 and PC3 about 64.89% of the total variation, respectively. Hence, by considering the PC1 and PC2, the grouping based on the properties of flour and cooked tubers are very much identical with the classification based on the flesh colors. From the multivariate analysis, it is evident that there was no similarity in the textural properties of the raw tubers among the varieties of same flesh color group. However, classification based on the texture profile parameters, pasting properties and DSC gelatinization parameters are same as that of the grouping selected for the study based on the flesh color of tubers. These results indicate that tubers possessing same flesh colors can be expected to have similar functional and cooking characteristics.
CONCLUSIONS
The study on the various textural, rheological, and gelatinization properties showed a significant difference among the different varieties of sweet potato. Both the first order and dual mechanism of the first order kinetic model were applied to explain the reduction in firmness of the cooked tubers, and based on the value of rate constants, the cooking quality of the tubers can be compared objectively and the varieties can be grouped according to the ease of cooking as: CO3-4 > Sree Arun > SV 280 > SV 98 > SI 60 > 362-7 > Sree Varun. This is almost in agreement with the classification of the varieties based on the pasting properties of flours extracted from different varieties. Degree of cooking can be predicted by using the linear model developed between degree of cooking and relative firmness. Principal component analysis of the texture profile parameters, differential scanning calorimetric properties, and pasting properties could also be used to group the varieties that are similar to the classification based on the color of the flesh of the tubers. Considering the high mealiness of the white flesh colored tubers, they can be a better option for the infant food formulations; however, to overcome vitamin A deficiency in children, orange fleshed varieties are ideal, showing that the suitability of tubers depends mainly on the purpose for which it is used.
REFERENCES
- Woolfe , J.A. 1992 . Sweet Potato—An Untapped Food Resource , Cambridge : University Press: Cambridge .
- FAO. FAO STAT statistical data base (online). 2009. http://apps.fao.org (http://apps.fao.org)
- Zang , T. and Oates , C.G. 1999 . Relationship between α-amylase degradation and physico-chemical properties of sweet potato starches . Food Chemistry , 65 : 157 – 163 .
- Toyama , J. , Ishiguro , K. , Noda , T. , Kumagai , T. and Yamakawa , O. 2003 . Influence of delayed harvest time on physico-chemical properties of sweet potato starch . Starch/Stärke , 55 : 558 – 563 .
- Zuraida , N. 2003 . Sweet potato as an alternative food supplement during rice shortage . Journal of Litbang Pertanian , 22 ( 4 ) : 150 – 155 .
- Jangchud , K. , Phimolsiripol , Y. and Haruthaithanasan , V. 2003 . Physico-chemical properties of sweet potato flour and starch as affected by blanching and processing . Starch/Stärke , 55 : 258 – 264 .
- Katayama , K. , Tamiyas , S. and Ishiguro , K. 2004 . Starch properties of new sweet potato lines having low pasting temperature . Starch/Stärke , 56 : 563 – 569 .
- Singh , S. , Raina , C.S. , Bawa , A.S. and Saxena , D.C. 2005 . Effect of heat-moisture treatment and acid modification on rheological, textural and differential scanning calorimetry characteristics of sweet potato starch . Journal of Food Science , 70 (6), E373–E378 )
- Noda , T. , Takahata , Y. , Sasto , T. , Ikama , H. and Mochida , H. 1996 . Physicochemical properties of starches from purple and orange fleshed sweet potato roots at two levels of fertilizers . Starch/Stärke , 48 ( 11/12 ) : 395 – 399 .
- Nandutta , A.M. and Howell , N.K. 2009 . Nutritional and rheological properties of sweet potato based infant food and its preservation using antioxidant . African Journal of Food Agriculture Nutrition and Development , 9 ( 4 ) : 1076 – 1090 .
- Truong , V.D. , Walter Jr , W.M. and Hamann , D.D. 1997 . Relationship between instrumental and sensory parameters of cooked sweet potato texture . Journal of Texture Studies , 28 ( 2 ) : 163 – 185 .
- Walter Jr , W.M. , Troung , V.D. , Wiesenborn , D.P. and Carvajal , P. 2000 . Rheological and physico-chemical properties of starches from moist and dry type sweet potatoes . Journal of Agriculture and Food Chemistry , 48 : 2937 – 2942 .
- Hamann , D.D. , Miller , N.C. and Purcell , A.E. 1980 . Effect of curing on the flavour and texture of baked sweet potato . Journal of Food Science , 45 : 992 – 994 .
- Damir , A.A. 1989 . Effect of heat penetration during cooking on some physico-chemical properties and microstructure of sweet potatoes . Food Chemistry , 34 : 41 – 55 .
- Watson , S. and Jarvis , M.C. 1995 . The origin and measurement of texture in sweet potatoes . Tropical Science , 35 : 229 – 235 .
- Takeda , Y. , Tokunaga , N. , Takeda , C. and Hizukuri , S. 1986 . Physico-chemical properties of sweet potato starches . Starch/Stärke , 38 ( 10 ) : 345 – 350 .
- Tian , S.J. , Rickard , J.E. and Blanshard , J.M.V. 1991 . Physico-chemical properties of sweet potato starch . Journal of the Science of Food and Agriculture , 57 : 459 – 491 .
- Garcia , A.M. and Walter , W.M. 1998 . Physico-chemical characteristics of starch from Peruvian sweet potato selection . Starch/Stärke , 50 : 331 – 337 .
- Collado , L.S. , Mabesa , R.C. and Corke , H. 1999 . Genetic variation in the physical properties of sweet potato starch . Journal of Agriculture and Food Chemistry , 47 : 4195 – 4201 .
- Noda , T. , Kobayashi , T. and Suda , I. 2001 . Effect of soil temperature on starch properties of sweet potato . Carbohydrate Polymer , 44 : 239 – 246 .
- Singh , N. , Kaur , L. , Ezekiel , R.J. and Guraya , H.S. 2005 . Microstructural, cooking and textural characteristics of poatato (Solanum Tubersome L.) tubers in relation to physico-chemical and functional properties of their flours . Journal of the Science of Food and Agriculture , 85 : 1275 – 1284 .
- Osundhahunsi , O.F. , Fagbemi , T.N. , Kesselman , E. and Shimoni , E. 2003 . Comparison of the physico-chemical properties and pasting characteristics of flour and starch from red and white sweet potato cultivars . Journal of Agriculture and Food Chemistry , 51 : 2232 – 2236 .
- Yadav , A.R. , Guha , M. , Tharanathan , R.N. and Ramteke , R.S. 2006 . Changes in characteristics of sweet potato flour prepared by different drying techniques . LWT—Food Science and Technology , 39 : 20 – 26 .
- Vimala , B. , Nair , G.M. , Ramanathan , S. , Jayaprakas , C.A. and Sreekumar , J. 2004 . “ Varietal improvement of sweet potato ” . In Annual Report 2003-04 , 34 – 36 . Trivandrum,Kerala , India : Central Tuber Crops Research Institute .
- Vimala , B. , Nair , G.M. , Ramanathan , S. , Jayaprakas , C.A. and Sreekumar , J. 2005 . “ Varietal improvement of sweet potato ” . In Annual Report 2004–05 , 36 – 37 . Trivandrum, Kerala , India : Central Tuber Crops Research Institute .
- Association of Official Analytical Chemists . 1984 . Official Methods of Analysis , 14th , Edited by: Williams , S. AOAC : Arlington, Virginia .
- Padmaja , G. , Moorthy , S.N. , Nambisan , B. , Babu , L. , Sundaresan , S. , Sajeev , M.S. , Nanda , S.K. , John , K.S. , Rajalekshmi , L. , Sudhadevi , K.S. and Nair , M.M. 2005 . Analytical Methodologies for Tropical Tuber Crops , Trivandrum, Kerala , , India : Central Tuber Crops Research Institute .
- Ramesh , M.N. , Sathyanarayana , K. and Girish , A.B. 1997 . Determination of degree of cooking of vegetables by compression testing. Journal of Food Science and Technology . 34 : 218 – 221 .
- Bourne , M.C. 2002 . Food Texture and Viscosity: Concept and Measurement , New York : Academic Press .
- Rizvi , A.F. and Tong , C.H. 1997 . Fractional conversion of determining texture degradation kinetics of vegetables . Journal of Food Science , 62 : 1 – 7 .
- Moreira , L.A. , Olivera , F.A.R. , Olivera , J.C. and Singh , R.P. 1994 . Textural changes in vegetables during thermal processing. I. A descriptive method to segregate effects of process treatments . Journal of Food Processing and Preservation , 18 : 483 – 490 .
- Moorthy , S.N. , Wenham , J.E. and Blanshard , J.M.V. 1996 . Effect of solvent extraction on the gelatinization properties of flour and starch of five cassava varieties . Journal of the Science of Food and Agriculture , 71 : 329 – 336 .
- Tijskens , L.M.M. , Hertog , M.L.A.T.M. , Vanschaik , A.C.R. and De Jager , A. 1999 . Modelling the firmness of Elstar apples during storage and transport . Acta Horticulture , 48 : 363 – 372 .
- Van Dijk , C. and Tijskens , L.M.M. “ Mathematical modeling of enzymatic reactions as related to the texture of fruits and vegetables after storage and mild preheat treatments ” . In Design of Minimal Processing Technology for Fruits and Vegetables , Edited by: Alzamora , S.M. , Tapia , S.M. , Lopez , A. , Malo , A. and Gaithersburg , M.D. 127 – 152 . New York, 2000 : Aspen Publishers Inc .
- Kilcast , D. 2004 . Texture in Foods: Vol 2: Solid Foods , Washington , DC : CRC Press .
- Lin , T.T. and Pitt , R.E. 1986 . Rheology of apple and potato tissue as affected by cell turgor pressure . Journal of Texture Studies , 17 : 291 – 313 .
- Rao , M.A. and Lund , D.B. 1986 . Kinetics of thermal softening of foods—A review . Journal of Food Processing and Preservation , 10 : 311 – 316 .
- Bourne , M.C. and Comstock , S.H. 1986 . Effect of temperature on firmness on thermally processed fruits and vegetables . Journal of Food Science , 51 : 531 – 533 .
- Kim , D.M. , Smith , N.L. and Lee , C.Y. 1994 . Effect of heat treatment on firmness of apples and apple slices . Journal of Food Processing and Preservation , 18 : 1 – 8 .
- Greve , L.C. , Shackel , K.A. , Ahmadi , H. , Mcardler , R.N. , Gohlke , J.R. and Labavitch , J.M. 1994 . Impact of heating on carrot firmness—Contribution of cellular turgour . Journal of Agriclture and Food Chemistry , 42 : 2896 – 2899 .
- Moorthy , S.N. 2001 . Tuber crop starches , Technical Bulletin , India : Central Tuber Crops Research Institute:Trivandrum, Kerala .
- Thybo , A.K. , Martens , H.J. and Lyshede , O.B. 1998 . Texture and microstructure of steam cooked, vacuum packed potato . Journal of Food Science , 6 : 692 – 695 .
- Otegbayo , B. , Aina , J. , Asiedu , R. and Bokanga , M. 2005 . Microstructure of boiled yam (Dioscorea spp.) and its implication for assessment of textural quality . Journal of Texture Studies , 36 : 324 – 332 .
- Sajeev , M.S. , Sreekumar , J. , Moorthy , S.N. , Suja , G. and Shanavas , S. 2008 . Texture analysis of raw and cooked tubers of short duration lines of cassava by multivariate and fractional conversion techniques . Journal of the Science of Food and Agriculture , 88 : 569 – 580 .
- Lamauro , C.J. and Bakshi , A.S. 1985 . Finite element analysis of moisture diffusion in stored foods . Journal of Food Science , 50 : 392 – 396 .
- Beleia , A. , Prudencio–Ferreira , S.H. , Yamashita , F. , Sakamoto , T.M. and Ito , L. 2004 . Sensory and instrumental texture analysis of cassava (Manihot esculenta Crantz) roots . Journal of Texture Studies , 35 : 542 – 553 .
- Beleia , A. , Yamashita , F. , de Moraes , S.R. , de Silveira , C.A. and Miranda , L.A. 2004 . Textural changes during cooking of cassava (Manihot esculenta Crantz) roots . Journal of the Science of Food and Agriculture , 84 : 1975 – 1978 .
- van Oirschot , Q.E.A. , Rees , D. and Aked , J. 2003 . Sensory characteristics of five sweet potato cultivars and their changes during storage under tropical conditions . Food Quality and Preference , 14 : 673 – 680 .
- Zhang , Z. , Christopher , C.W. , Wheatley , C.C. and Corke , H. 2002 . Biochemical changes during storage of sweet potato roots differing in dry matter content . Post Harvest Biology and Technology , 24 : 317 – 325 .
- Guraya , H.S. , Toledo , R.T. and Kays , S.J. 1998 . Effect of cultivar and root storage on pasting characteristics of sweet potato starches . Tropical Science , 38 : 57 – 61 .
- Moorthy , S.N. 2004 . “ Tropical sources of starch ” . In Starch in Food , Edited by: Eliasson , A.C. 312 – 359 . London : Woodhead Publishers .
- Noda , T. , Kimura , T. , Otani , M. , Ideta , O. , Shimada , T. , Saito , A. and Suda , I. 2002 . Physico-chemical properties of amylose free starch from transgenic sweet potatoes . Carbohydrate Polymer , 49 : 253 – 260 .