Abstract
Casein hydrolysates with a degree of hydrolysis of 13.5% were prepared by hydrolyzing casein with an alkaline protease Alcalase, and showed ACE-inhibition in vitro with an IC50 value of 45.2 μg/mL. The hydrolysates were modified by plastein reaction catalyzed by a neutral protease Neutrase to reveal the impact of the coupled Neutrase-catalyzed plastein reaction on the ACE-inhibition of the casein hydrolysates. The effects of addition level of Neutrase, substrate concentration, reaction temperature, and time on the plastein reaction of the casein hydrolysates were studied with the varying amount of free amino groups of the modified hydrolysates as index. The results illustrated that the amount of free amino groups of the modified hydrolysates increased in all occasions, and the addition level of Neutrase, substrate concentration, and reaction time had a clear impact on the plastein reaction. Six modified hydrolysates were prepared at a substrate concentration of 40% (by weight), Neutrase addition level of 3 kU/g peptides, reaction temperature of 35°C, and different reaction time. The assay results highlighted that the coupled Neutrase-catalyzed plastein reaction improved the ACE-inhibition of six modified hydrolysates with IC50 values ranging from 15.6 to 20.0 μg/mL. Size exclusion chromatography analysis showed that some plasteins with a molecular weight of about 68 kDa existed in the modified hydrolysates. The results also demonstrated that it was the coupled Neutrase-catalyzed plastein reaction but not further hydrolysis of casein hydrolysates that enhanced the ACE-inhibition of the modified casein hydrolysates.
INTRODUCTION
Hypertension is recognized as a serious risk factor for cardiovascular diseases and stroke.Citation[1] The condition can be controlled by some drugs that specifically inhibit the activity of angiotensin-converting enzyme (ACE, EC 3.4.15.1), such as captopril, enalapril, alacepil, lisinopril, and ramipril. Angiotensin I is cleaved by ACE into angiotensin II, a potent vasoconstrictor. Meanwhile, bradykinin, a vasodilator, is inactivated by ACE. Consequently, inhibition of ACE leads blood pressure to decrease. Synthetic drugs are used in clinic treatment of hypertension; however, many of them have several side effects, including coughing, taste disturbances, and skin rashes.Citation[2] Researchers have been devoted towards producing foods with ACE-inhibitory peptides, beneficial for individuals with higher blood pressure.Citation[3] ACE-inhibitory peptides can be derived from many food proteins,Citation[4] including milk proteins,Citation[5, Citation6] plant proteins,Citation[7– Citation11] and egg proteins,Citation[12] and used as potential alternatives to synthetic drugs. The ACE-inhibitory peptides are not active within the parent proteins but can be released and activated by enzymatic hydrolysis.Citation[13– Citation15] To obtain stronger ACE-inhibitory peptides from food proteins, fermentation and hydrolysis are two effective approaches used by the researchers.
Although the structure-activity relationship of ACE-inhibitory peptides is not fully understood, some general features have been found. Usually, ACE-inhibitory peptides contain 2 to 12 amino acid residues, even though some active peptides with up to 27 amino acid residues have been identified.Citation[16– Citation18] Much work revealed that the binding of ACE-inhibitory peptides to ACE was strongly influenced by the C-terminal sequence of the peptides, especially by the hydrophobic amino acids such as leucine, valine, and isoleucine in the C-terminal. If proline is present at each of the three C-terminal positions of the peptides, the peptides will be more active. When aromatic amino acids exist in the N-terminal of the peptides, they will also enhance the ACE-inhibitory activity of the peptides. Pripp et al. established quantitative structure-activity relationships for the ACE-inhibitory peptides derived from milk proteins.Citation[19] Gobbetti et al. reported that the catalytic sites of ACE had different conformational requirements; therefore, there is a need for developing a complex mixture of peptides, with slightly different conformational features, so as to inhibit ACE activity more effectively.Citation[20] In addition, the mechanism of ACE inhibition may involve the interaction of the inhibitor with the subsites not normally occupied by the substrates, or with an anionic inhibitor binding site that is different from the catalytic site of the enzyme.Citation[21]
Plastein reaction was considered to involve the formation of polypeptides by a reversal of the usual protein hydrolysis by proteases, and applied to prepare higher-molecular, protein-like substances.Citation[22] The precise reaction mechanism remains at the stage of an intellectual curiosity, and there is continuing argument about it.Citation[23] Three different mechanisms, in earlier thoughts, were suggested as condensation,Citation[24] transpeptidation,Citation[25] and physical forces.Citation[26] Recent work suggested that several pathways might play a role in the reaction simultaneously.Citation[27] Plastein reaction had been used to enhance biological value or functional properties of food proteins,Citation[28, Citation29] improve flavor quality of protein hydrolysates,Citation[30] or provide a way to synthesize new proteins.Citation[31] New peptides with amino acid sequences different from raw proteins might be generated, if condensation and transpeptidation do exist between the peptide molecules during plastein reaction of the protein hydrolysates. Therefore, this approach might be applied to modify the biological activity of the protein hydrolysates. It was reported that the antioxidant activity of the hydrolysates from squid hepatopancreas with Alcalase could be improved by plastein reaction,Citation[32] and the ACE-inhibitory activity in vitro of casein hydrolysates or soybean protein hydrolysates was enhanced significantly by Alcalase-catalyzed plastein reaction.Citation[33– Citation35]
In the reports mentioned, a protease was applied to prepare protein hydrolysates and to carry out the coupled plastein reaction, viz. the same protease was used in the two steps. However, the impact of the plastein reaction on the ACE-inhibitory activity of the modified hydrolysates is still not clear when another protease is applied in the coupled plastein reaction. Based on this consideration, casein hydrolysates were prepared by Alcalase-catalyzed hydrolysis of casein in the present work, and were then subjected to Neutrase-catalyzed plastein reaction for further modification. Some conditions of plastein reaction, including substrate concentration, ratio of enzyme to substrate (E/S), reaction time, and temperature, were primarily studied by single factor trials. The ACE-inhibitory activities of the casein hydrolysates and some modified casein hydrolysates prepared were evaluated in vitro and compared, in order to reveal the impact of the coupled Neutrase-catalyzed plastein reaction on the activity of the casein hydrolysates prepared with Alcalase.
MATERIALS AND METHODS
Materials and Chemicals
Casein, N-(3-[2-furyl]acryloyl)-L-phenylalanylglycylglycine (FAPGG), and rabbit lung acetone powder were purchased from Sigma-Aldrich Co. (St. Louis, MO, USA). Alcalase with a practical activity of 11 kU/g was purchased from Pangbo Biochem. Inc. (Nanning, China). Neutrase with a practical activity of 85 kU/g was purchased from Aoboxing Biotechnology Co., Ltd. (Beijing, China). Captopril (purity >99.0%) was purchased from Fluka (Fluka Chemie AG, Buchs, Switzerland). Other reagents used were reagent grade chemicals. Highly purified water prepared with Milli-Q PLUS (Millipore Corporation, New York, NY, USA) was used for the preparation of all buffers and solutions.
Preparation of Casein Hydrolysates
The preparation of casein hydrolysates followed the reported methodCitation[35] with some modifications. Casein (10 g, on protein basis) was dissolved in 100 mL of water to give an original protein concentration of 10% (w/v). The pH of casein solution was adjusted to 8.5 by a few drops of 2 mol/L NaOH. The Alcalase solution was prepared immediately prior to use. After withdrawal of a 5-mL sample (zero time sample) from the casein solution, the hydrolysis process was started by adding Alcalase solution to the remaining casein solution (giving approximately E/S ratio of 1 kU/g casein). The reaction mixture was kept at 55°C with continuous stirring, and 5-mL samples were withdrawn from the hydrolysis system after 2, 4, 6, 8, 10, and 12 h of hydrolysis, respectively. During hydrolysis, no NaOH solution was added into the hydrolysis system. The separated samples were heated at 100°C for 15 min to inactivate Alcalase, cooled to room temperature, adjusted to pH 4.6 with 1 mol/L HCl, and centrifuged at 11,000× g for 20 min. The supernatant, separated, was used for further analysis as described below. According to the analysis results, casein hydrolysates with the highest ACE-inhibitory activity in vitro were prepared, lyophilized, and stored at −20°C. The prepared hydrolysates were used in plastein reaction as substrate.
Analysis of Protease Activity and Protein or Peptide Content
The practical activity of Alcalase and Neutrase were spectrophotometric assayed by a method described by Sarath et al.Citation[36] with some modifications. Nitrogen content of the casein, casein hydrolysates, and modified casein hydrolysates were determined by the Kjeldahl procedure according to FIL-IDF 20B:1993,Citation[37] and multiplied by 6.38 to give the value of protein or peptide content.
Amount of Free Amino Groups and Degree of Hydrolysis
The amount of free amino groups of the casein, the casein hydrolysates, or the modified casein hydrolysates on protein or peptide basis was measured by o-phthaldialdehyde (OPA) assayCitation[38, Citation39] with some modifications. The OPA reagent was prepared by combing the following reagents to a final volume of 100 mL with water: 75 mL of 0.2 mol/L sodium borate buffer (pH 9.5), 5 mL of 400 g SDS/L, 80 mg OPA (in 1 mL methanol), and 400 μL β-mercaptoenthanol. The reagent was prepared daily and protected from light. The OPA assay was carried out by adding 3 mL of sample (or standard) solution to 3 mL of OPA reagent. The absorbance of the solution was measured at 340 nm with an UV spectrophotometer (UV-2401PC, Shimadzu, Kyoto, Japan), and taken after 5 min. L-Leucine standard solution was prepared as follows: L-leucine (0.3000 g) was dissolved in 1 mol/L HCl (5 mL) and diluted to a final concentration of 0.6 g/mL with water. A serial of diluted solutions in the range of 0 to 30 μg/mL was prepared by further dilution and used to determine the standard curve.
The degree of hydrolysis (DH) of the casein hydrolysates was determined by assaying the amount of free amino groups of the casein hydrolysates by OPA method, and calculated by using the equation given by Adler-Nissen:Citation[40]
Determination of ACE-Inhibitory Activity In Vitro
The assay for ACE inhibition was performed as per the method of Murray et al.Citation[41] with some modifications, with FAPGG as substrate and the extract of rabbit lung acetone powder as the ACE source. The reaction mixture contained 100 μL of the sample dissolved in deionized water in a concentration range from 0 to 10 mg/mL, 500 μL of 1.6 mmol/L FAPGG in 0.1 mol/L sodium borate buffer (pH 8.3) with 0.3 mol/L NaCl, and 300 μL 10× diluted rabbit lung acetone extract in 0.1 mol/L sodium borate buffer (pH 8.3) containing 5% (v/v) glycerol. The ACE extract was added to initiate the reaction. The reaction was terminated by adding 100 μL of 0.1 mol/L EDTA solution after 30 min incubation at 37°C. The EDTA solution was added immediately before the ACE extract in zero-time control assays. The decrease in absorbance at 340 nm was measured in triplicate over a 30 min incubation period in the spectrophotometer (UV-2401PC, Shimadzu, Kyoto, Japan), and taken as a measure of the ACE activity. A control sample containing 100 μL of deionized water instead of the casein hydrolysates was assayed in quadruplicate. The ACE inhibition (%) was calculated as [1 − (ΔA inhibitor/ΔA control)] × 100%, where ΔA inhibitor and ΔA control were the decrease in absorbance at 340 nm of the sample and the control, respectively. The concentration of the sample needed to inhibit ACE by 50% (IC50) under these conditions was obtained by assaying variously diluted solutions of the sample and plotting the ACE inhibition percentage as a function of the peptide concentration.Citation[42] A synthetic ACE inhibitor, Captopril, was used as the positive control with an IC50 of 5.2 nmol/L.
Modification of Casein Hydrolysates by Neutrase-Catalyzed Plastein Reaction
The casein hydrolysates prepared were dissolved in water to obtain a final concentration of 20, 30, 40, 50, and 60% by weight, respectively. Neutrase was added to the casein hydrolysates with a different amount to obtain a fixed E/S ratio of 3 kU/g peptides. The reaction mixture was adjusted to a fixed pH of 6.8 and kept at 35°C for 5 h with continuous stirring. After the reaction, the mixture was heated at 100°C for 15 min to inactivate Neutrase. The resulting product, classified as modified casein hydrolysates, were stored at −20°C until analysis of the amount of free amino groups and ACE-inhibitory activity. The varying amount of free amino groups of the modified casein hydrolysates was calculated by subtracting the amount of free amino groups of the casein hydrolysates before plastein reaction from that of the modified casein hydrolysates after plastein reaction, and expressed as μmol-NH2/g peptides.
A similar approach was used to study the influences of E/S ratio (by changing addition amount of Neutrase), reaction temperature (by keeping reaction mixture at different temperature), and reaction time (by choosing different reaction time) on the varying amount of free amino groups of the modified casein hydrolysates by single factor trials. The suitable conditions were selected based on the experimental results to insure less hydrolysis of the casein hydrolysates. After that, six modified casein hydrolysates were prepared with the selected conditions, and evaluated for the amount of free amino groups and ACE-inhibitory activity in vitro.
A control trial was carried out to hydrolyze the casein hydrolysates by Neutrase. The hydrolysis conditions applied were the same as the conditions applied in the preparation of the modified casein hydrolysates except that substrate concentration was 10% by weight and reaction time was 2, 4, and 6 h, respectively. After the reaction, the reaction mixture was heated at 100°C for 15 min to inactivate Neutrase. Three resulting products, classified as hydrolyzed casein hydrolysates, were stored at –20°C until analysis of their amount of free amino groups and ACE-inhibitory activity in vitro.
Assay of Size Exclusion Chromatography
The assay was performed as a reported methodCitation[43] with some modifications. The casein hydrolysates or the modified casein hydrolysates were dissolved in 0.1 mol/L Na2HPO4−0.1 mol/L NaOH buffer (pH 12) to give a final peptide concentration of 2 mg/mL. Half of a milliliter of the sample was applied to a 10 × 300 mm Amersham Pharmacia Superdex-75 10/300 GL column (GE Healthcare, Amersham Biosciences, Uppsala, Sweden) in an AKTA Explorer 100 (GE Healthcare). The assay was operated with a fixed pressure of 1.80 MPa and an elution rate of 0.5 mL/min in a temperature-controlled cabinet set at 4°C. The elution was monitored with an UV detector at 280 nm. Bovine serum albumin (66.2 kDa), cytochrome c (12.4 kDa), insulin (5.7 kDa), oxidized L-glutathione (0.6 kDa), and L-tyrosine (0.2 kDa) were used to show molecular weight distribution of the peptides in the samples.
Statistical Analysis
All data were expressed as means ± SD from at least three independent trials. Differences between the mean values of multiple groups were analyzed by one-way analysis of variance (ANOVA) and Duncan's multiple range tests with SPSS 13.0 for Windows (SPSS Inc., Chicago, IL, USA). The level of confidence required for significance was set at P < 0.01. Microsoft Excel version 2003 software (Microsoft Corporation, Redmond, WA, USA) were used to report the data.
RESULTS
DH and ACE-Inhibitory Activity of Casein Hydrolysates Prepared by Alcalase
Alcalase was used to prepare casein hydrolysates. The DH and ACE-inhibitory activity of the hydrolysate obtained over a 12-h hydrolysis period are shown in The DH of the hydrolysates increased as hydrolysis time was prolonged, ranging from 9.8 to 14.9%. The ACE-inhibitory activity in vitro of the hydrolysates increased during the first 6 h of hydrolysis, ranging from 36.9 to 52.9%. After then, the activity of the hydrolysates showed a decreasing trend, indicating that excess hydrolysis would impair the activity of the hydrolysates. If hydrolysis time was 6 h, the hydrolysates had free amino groups of about 1690 μmol/g peptides and a DH of 13.5%, and exhibited the highest ACE-inhibitory activity in vitro (IC50 = 45.2 μg/mL). Based on that fact, the casein hydrolysates with a DH of 13.5% were selected as the substrate of plastein reaction.
Figure 1 ACE-inhibitory activity and DH of casein hydrolysates prepared under casein concentration 10% (w/v), original pH 8.5, reaction temperature 55°C, E/S ratio 1 kU/g proteins with different hydrolysis time. The final concentration of casein or casein hydrolysates for ACE-inhibitory activity assay was 50 μg/mL on protein or peptide basis. Column chart was for ACE-inhibitory activity, and graph chart was for DH.
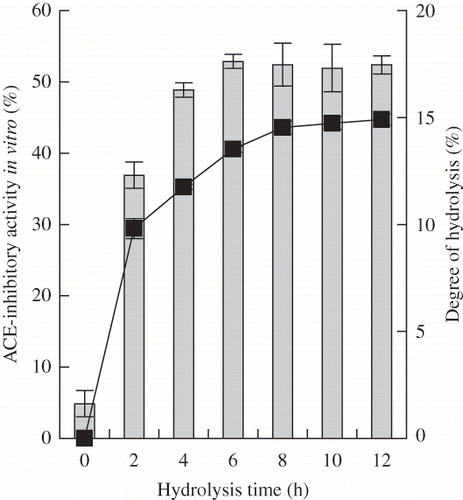
Modification of Casein Hydrolysates by Neutrase-Catalyzed Plastein Reaction
Neutrase was used in the coupled plastein reaction to modify the casein hydrolysates prepared. During plastein reaction, further hydrolysis of the hydrolysates induced by Neutrase might be possible and should not be ignored. Therefore, the suitable conditions should be selected to avoid further hydrolysis of the hydrolysates.
Impact of substrate concentration
The impact of substrate concentration on the plastein reaction of the casein hydrolysates catalyzed by Neutrase is shown in Analysis results showed that the amount of free amino groups of the modified casein hydrolysates increased after the plastein reaction, leading to the varying amount of free amino groups of the modified hydrolysates, a positive value. It indicated the occurrence of further hydrolysis in the reaction system. As substrate concentration increased from 20 to 60% (by weight), the varying amount of free amino groups of the modified hydrolysates decreased from 258.8 to 34.1 μmol/g peptides, indicating that the hydrolysis was alleviated. If the substrate concentration was higher than 50%, the modified hydrolysates took the physical form of thixotropic gels, which might hinder the catalysis efficiency of Neutrase for the reaction mixture was too viscous. If substrate concentration was too low (such as 20% by weight), much hydrolysis occurred. The suitable substrate concentration was selected at 40% by weight.
Figure 2 Effect of substrate concentration (%, by weight) on the varying amount of free amino groups of the modified casein hydrolysates during plastein reaction. The reaction was carried out at original pH of 6.8, E/S ratio of 3 kU/g peptides, reaction temperature of 35°C, and reaction time of 5 h. (Color figure available online.)
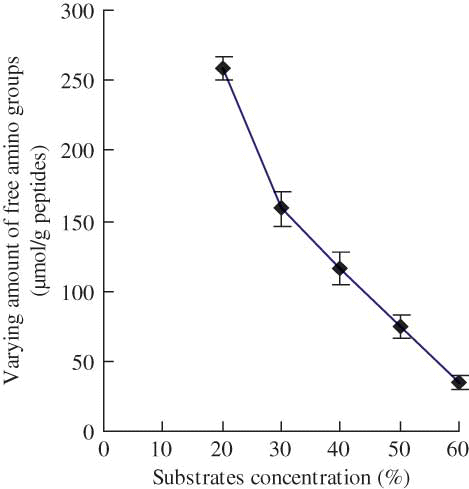
Impact of E/S ratio
The impact of E/S ratio on the plastein reaction of the casein hydrolysates catalyzed by Neutrase is given in When the Neutrase addition level increased from 0.3 to 7.5 kU/g peptides, the varying amount of free amino groups of the modified casein hydrolysates increased from 88.8 to 167.2 μmol/g peptides, implying that much hydrolysis occurred. Considering that too low of an addition level of Neutrase would decrease the rate of plastein reaction, and on the contrary, too high of an addition level of Neutrase would lead to severe hydrolysis of the casein hydrolysates, the selected E/S ratio was 3 kU/g peptides.
Figure 3 Effect of E/S ratio on the varying amount of free amino groups of the modified casein hydrolysates during plastein reaction. The reaction was carried out at original pH of 6.8, substrate concentration of 40% by weight, reaction temperature of 35°C, and reaction time of 5 h. (Color figure available online.)
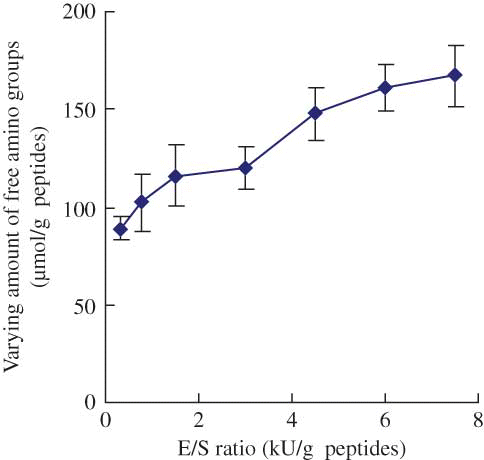
Impact of reaction temperature
The impact of reaction temperature on the Neutrase-catalyzed plastein reaction of the casein hydrolysates is shown as Reaction temperature showed a slight influence. The varying amount of free amino groups of the modified casein hydrolysates had a low value of 116.1 μmol/g peptides at 30°C, or a high value of 123.4 μmol/g peptides at 20°C. The temperature selected for the plastein reaction was 35°C.
Figure 4 Effect of reaction temperature on the varying amount of free amino groups of the modified casein hydrolysates during plastein reaction. The reaction was carried out at original pH of 6.8, substrate concentration of 40% by weight, E/S ratio of 3 kU·g−1 peptides, and reaction time of 5 h. (Color figure available online.)
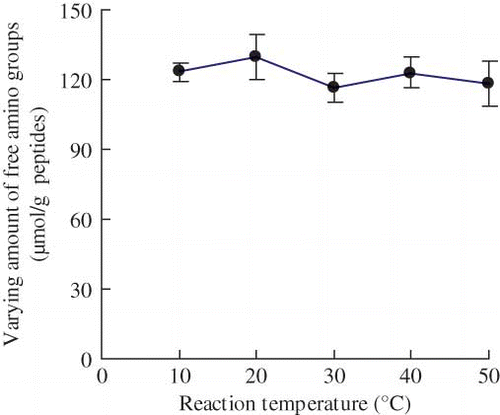
Impact of reaction time
The impact of reaction time on the Neutrase-catalyzed plastein reaction of the casein hydrolysates is listed in . When plastein reaction progressed from the beginning to 8 h, the varying amount of free amino groups of the modified casein hydrolysates showed a decreasing trend, indicating that less hydrolysis occurred relatively. After that, the varying amount of free amino groups of the modified hydrolysates showed an increasing trend, implying much hydrolysis occurred relatively. If the modified hydrolysates were intended to be prepared with less hydrolysis, the suitable reaction time should be 6 to 8 h.
Table 1 Varying amount of free amino groups and ACE-inhibitory activities of six modified casein hydrolysates prepared
ACE-Inhibitory Activity of the Modified Casein Hydrolysates In Vitro
Six modified casein hydrolysates were prepared with the selected conditions: original pH of 6.8, substrate concentration of 40% by weight, E/S ratio of 3 kU/g peptides, 35°C, and reaction time of 2, 4, 6, 8, and 12 h, respectively. The ACE-inhibitory activities of six modified casein hydrolysates are listed in . The data showed that the activities of six modified hydrolysates were enhanced by plastein reaction with IC50 values decreasing from 45.2 to 15.6–20.0 μg/mL. This demonstrated that Neutrase-catalyzed plastein reaction could improve the ACE-inhibitory activity of casein hydrolysates prepared by Alcalase.
A control study was carried out to show the impact of the further hydrolysis of the casein hydrolysates by Neutrase on the ACE-inhibitory activities of the resulting product (the hydrolyzed casein hydrolysates). When the casein hydrolysates were further hydrolyzed with Neutrase under the selected conditions, the ACE-inhibitory activities and varying amount of free amino groups of three hydrolyzed casein hydrolysates were changed, as shown by those listed in . The data showed that the varying amount of free amino groups of the hydrolyzed casein hydrolysates increased (viz. casein hydrolysates were hydrolyzed by Neutrase) and the ACE-inhibitory activity of each hydrolyzed casein hydrolysates was lower than that of the original casein hydrolysates. This supported the conclusion shown in that further hydrolysis of the casein hydrolysates with a DH of 13.5% would impair the corresponding ACE-inhibitory activity.
Table 2 Impacts of further hydrolysis of casein hydrolysates by Neutrase on ACE-inhibitory activity in vitro of the hydrolyzed casein hydrolysates obtained
Assay of Size Exclusion Chromatography
The analysis results of size exclusion chromatography for the original casein hydrolysate and a modified casein hydrolysate are shown in Based on the peptide distribution profiles and molecular weights of the standards (), the main fraction of the casein hydrolysates were eluted during a time period of 25 to 35 min with corresponding molecular weights ranging from 0.2 to 5.8 kDa. The main fraction of the modified hydrolysates shared a similar elution profile to that of the casein hydrolysates. Interestingly, some plasteins with molecular weight of about 68 kDa existed in the modified hydrolysates but not in the casein hydrolysates, indicating that plastein reaction (viz. condensation) did occur.
Figure 5 Analysis of the standards (a) casein hydrolysates and the modified hydrolysates (b) in AKTA Explorer 100 with Superdex-75 column. The analysis was carried out at a flow rate of 0.5 mL/min with 0.1 mol/L Na2HPO4 and NaOH buffer (pH 12) and monitored at 280 nm. The standards were bovine serum albumin (66.2 kDa), cytochrome c (12.4 kDa), insulin (5.7 kDa), oxidized L-glutathione (0.6 kDa), and L-tyrosine (0.2 kDa), and appeared as peak A to E in (a), respectively. Casein hydrolysates were prepared by hydrolyzing casein under casein concentration 10% (w/v), original pH 8.5, reaction temperature 55°C, E/S ratio 1 kU/g proteins, and reaction time 6 h to a DH of 13.5%. The modified hydrolysates were prepared from casein hydrolysates by Nutrase-catalyzed plastein reaction at an original pH of 6.8, substrate concentration of 40% (by weight), Neutrase addition level of 3 kU/g peptides, reaction temperature of 35°C, and reaction time of 6 h. (Color figure available online.)
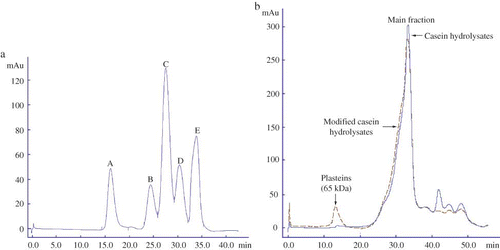
DISCUSSION
Preparation of Casein Hydrolysates
Much work had been carried out on casein-derived bioactive peptides, for they were encrypted within the primary structures of intact casein.Citation[44] Cheung et al. found that proline, tryptophane, tyrosine, and phenylalanine were the most effective at the ultimate C-terminal position, proline binding exceptionally well to ACE.Citation[45] Casein, rich in proline, tryptophane, tyrosine, and phenylalanine, gives Alcalase-catalyzed casein hydrolysates more opportunity to have these amino acids at the C-terminal position and thereby higher ACE-inhibitory activity, for Alcalase has higher specificity for hydrophobic amino acids.Citation[46] When sodium caseinate was hydrolyzed by thermolysin, the obtained hydrolysates showed an IC50 value of 95 μg/mL.Citation[13] Casein hydrolysates prepared with pepsin gave an IC50 value of 52.8 μg/mL.Citation[6] The casein hydrolysates prepared in the present work had a DH of 13.5% and an IC50 value of 45.2 μg/mL, which shared similarity to this work.
It is reasonable to use the prepared hydrolysates as substrate of plastein reaction. The hydrolysates exhibited the highest ACE-inhibitory activity, and excess hydrolysis of the hydrolysates with Alcalase would lead the hydrolysates to a lower activity (). If the hydrolysates were modified by the Neutrase-catalyzed plastein reaction or further hydrolysis, the impacts of two treatments on the activity of the resulting products could be reflected directly by the corresponding change in the activity.
Plastein Reaction Conditions of Casein Hydrolysates and ACE-Inhibitory Activity of the Modified Casein Hydrolysates
Sukan and Andrews reported that when highly concentrated solutions of protein hydrolysates were incubated with proteases, water-insoluble and gel-forming products formed.Citation[47, Citation48] Water insoluble products in the gel or thixotropic solutions had been used to evaluate the extent of plastein reaction.Citation[23] In the present work, the varying amount of free amino groups of the modified casein hydrolysates was used as the index of plastein reaction. Evaluation results showed that further hydrolysis occurred during Neutrase-catalyzed plastein reaction of the casein hydrolysates, which was different from the previous work.Citation[33– Citation35] Alcalase was used to carry out the hydrolysis of casein or soybean protein isolates and the plastein reaction of the prepared hydrolysates in the previous work, and the amount of free amino groups of the modified hydrolysates were lower than that of the original hydrolysates. Neutrase is a protease from Bacillus subtilis with specificity mainly for leucine and phenylalanine.Citation[46] Neutrase differs somewhat from Alcalase in the specificity. When Alcalase was used to prepare the casein hydrolysates and Neutrase was used to carry out plastein reaction of the hydrolysates, Neutrase would induce further hydrolysis of the hydrolysates for it could break those peptide bonds insensitive to Alcalase. Consequently, hydrolysis and peptide joint of the hydrolysates happened simultaneously during Neutrase-catalyzed plastein reaction. Due to adverse impact of further hydrolysis on the ACE-inhibitory activity of the hydrolysates, suitable conditions of plastein reaction should be selected to avoid much hydrolysis.
Plastein reaction undertook at a higher substrate concentration ranged from 30 to 50% by weight.Citation[49, Citation50] In the present work, substrate concentration showed a clear impact on Neutrase-catalyzed plastein reaction of the casein hydrolysates. When substrate concentration was too low, much hydrolysis occurred (). Addition level of Neutrase also gave impact on the varying amount of free amino groups of the modified casein hydrolysates (). The reaction temperature had two impacts on plastein reaction: first, the activity of protease was regulated by temperature; and second, low temperature was beneficial to peptide synthesis as plastein reaction is considered as an exothermic reaction.Citation[13] If the reaction temperature was too high, although the initial rate of plastein reaction was very rapid, the reaction might be soon stopped, and the overall result was much lower than that at low temperature for a long reaction time.Citation[29, Citation50] Reaction temperature only gave a small impact in the present study (). The impact of reaction time on plastein reaction of the casein hydrolysates was complex (). When reaction time was 6 or 8 h, the hydrolysis was less relatively, as the varying amount of free amino groups of the modified casein hydrolysates were 88.4 ± 3.9 or 76.9 ± 8.5 μmol/g peptides, which were lower than that of other modified casein hydrolysates. Therefore, substrate concentration of 40% (by weight), Neutrase addition level of 3 kU/g peptides, reaction temperature of 35°C, and reaction time of 6 or 8 h were selected as suitable conditions for Neutrase-catalyzed plastein reaction of the casein hydrolysates.
Hydrophobic amino acid residues are preferably linked together during plastein reaction.Citation[51] It could be inferred that some now peptides rich in hydrophobic amino acids should be generated during plastein reaction and, thus, have higher ACE-inhibitory activity. The specificity of Alcalase (mainly for hydrophobic amino acids) was helpful to prepare casein hydrolysates rich in hydrophobic amino acids, while that of Neutrase (mainly for leucine and phenylalanine) was helpful to link hydrophobic amino acid residues of the peptides in the hydrolysates during plastein reaction. It is reasonable that the new peptides as the resultants of plastein reaction would contain more hydrophobic amino acids and exhibit higher activity than the original peptides. Neutrase-catalyzed plastein reaction, therefore, enhanced the activity of the modified hydrolysates, although some hydrolysis also occurred during the plastein reaction (). Oppositely, further hydrolysis of the hydrolysates directly by Alcalase () or Neutrase () showed a clear adverse impact on the activity of the final products. Based on these facts, it could be concluded primarily here that it was plastein reaction but not further hydrolysis of the hydrolysates that enhanced the activity of the modified hydrolysates.
The present result illustrated that the coupled Neutrase-catalyzed plastein reaction was also an effective approach to improve the ACE-inhibitory activity of casein hydrolysates prepared with Alcalase. However, the nature of the peptides in the modified casein hydrolysates needs to be studied to reveal the detailed role of plastein reaction. On the other hand, the ACE-inhibitory activity of the modified casein hydrolysates in the present work was lower than that in the previous work,Citation[35] implying that the role of the protease used and the hydrolysis that occurred during plastein reaction should be studied.
CONCLUSIONS
Casein was hydrolyzed by Alcalase to prepare casein hydrolysates with a DH of 13.5%. Casein hydrolysates obtained showed ACE inhibition in vitro with an IC50 value of 45.2 μg/mL. Casein hydrolysates were modified by a Neutrase-catalyzed plastein reaction with suitable conditions as follows: an original pH of 6.8, substrates concentration of 40% by weight, Neutrase addition level of 3 kU/g peptides, reaction temperature of 35°C, and reaction time of 6 or 8 h. Size exclusion chromatography analysis showed that some plasteins with molecular weights about 68 kDa existed in the modified casein hydrolysates. Meanwhile, the ACE-inhibitory activities of the modified hydrolysates were enhanced by plastein reaction with IC50 values decreasing from 45.2 to 15–20.0 μg/mL. The result showed that it was Neutrase-catalyzed plastein reaction but not further hydrolysis of casein hydrolysates that enhanced the ACE-inhibitory activity of the modified hydrolysates.
ACKNOWLEDGMENTS
This work was supported by the National Natural Science Foundation of China (No. 30972132) and the Innovative Research Team of Higher Education of Heilongjiang Province (No. 2010td11). The authors thank Mrs. Bao-Jing Cheng for her help in size exclusion chromatography analysis, and also thank the anonymous reviewers and editors for their constructive work and valuable suggestions to this article.
REFERENCES
- López-Fandiño , R. , Otte , J. and van Camp , J. 2006 . Physiological, chemical and technological aspects of milk-protein-derived peptides with antihypertensive and ACE-inhibitory activity . International Dairy Journal , 16 : 1277 – 1293 .
- Atkinson , A.B. and Robertson , J.I.S. 1979 . Captopril in the treatment of clinical hypertension and cardiac failure . Lancet , 314 : 836 – 839 .
- FitzGerald , R.J. , Murray , B.A. and Walsh , D.J. 2004 . Hypotensive peptides from milk proteins . Journal of Nutrition , 134 : 980S – 988S .
- Li , G.H. , Le , G.W. , Shi , Y.H. , Shrestha and Angiotensin , S. 2004 . I-converting enzyme inhibitory peptides derived from food proteins and their physiological and pharmacological effects . Nutrition Research , 24 : 469 – 486 .
- Abubakar , A. , Saito , T. , Kitazawa , H. , Kawai , Y. and Itoch , T. 1998 . Structural analysis of new antihypertensive peptides derived from cheese whey protein by proteinase K digestion . Journal of Dairy Science , 81 : 3131 – 3138 .
- Miguel , M. , Contreras , M.M. , Recio , I. and Aleixandre , A. 2009 . ACE-inhibitory and antihypertensive properties of a bovine casein hydrolysate . Food Chemistry , 112 : 211 – 214 .
- Li , G.H. , Shi , Y.H , Liu , H. and Le , G.W. 2006 . Antihypertensive effect of alcalase generated mung bean protein hydrolysates in spontaneously hypertensive rats . European Food Research and Technology , 222 : 733 – 736 .
- Pihlanto , A. , Akkanen , S. and Korhonen , H.J. 2008 . ACE-inhibitory and antioxidant properties of potato (Solanum tuberosum) . Food Chemistry , 109 : 104 – 112 .
- Suh , H.J. , Whang , J.H. and Lee , H. A . 1999 . peptide from corn gluten hydrolysate that is inhibitory toward angiotensin I converting enzyme . Biotechnology Letters , 21 : 1055 – 1058 .
- Li , F.J. , Yin , L.J. , Lu , X. and Li , L.T. 2010 . Changes in angiotensin I-converting enzyme inhibitory activities during the ripening of Douchi (a Chinese traditional soybean product) fermented by various starter cultures . International Journal of Food Properties , 13 : 512 – 514 .
- Li , F.J. , Cheng , Y.Q. , Yin , L.J. , Liu , H.J. and Li , L.T. 2011 . Application of electrolyzed water to improve angiotensin I-converting enzyme inhibitory activities of fermented soybeans started with Bacillus subtilis B1 . International Journal of Food Properties , 14 : 145 – 156 .
- Miguel , M. , Alonso , M.J. , Salaices , M. , Aleixandre , A. and López-Fandiño , R. 2007 . Antihypertensive, ACE-inhibitory and vasodilator properties of an egg white hydrolysate: Effect of a simulated intestinal digestion . Food Chemistry , 104 : 163 – 168 .
- Fujimaki , M. , Kato , M. , Aria , S. and Yamashita , M. 1971 . Application of microbial proteinase to soybean and other materials to improve acceptability . Journal of Applied Bacteriology , 34 : 119 – 131 .
- Otte , J. , Shalaby , S.M.A. , Zakora , M. , Pripp , A.H. and El-Shabrawy , S.A. 2007 . Angiotensin-converting enzyme inhibitory activity of milk protein hydrolysates: Effect of substrate, enzyme and time of hydrolysis . International Dairy Journal , 17 : 488 – 503 .
- Otte , J. , Shalaby , S.M.A. , Zakora , M. and Nielsen , M.S. 2007 . Fractionation and identification of ACE-inhibitory peptides from α-lactalbumin and β-casein produced by thermolysin-catalysed hydrolysis . International Dairy Journal , 17 : 1460 – 1472 .
- Robert , M.C. , Razaname , A. , Mutter , M. and Juillerat , M.A. 2004 . Identification of angiotensin-I-converting enzyme inhibitory peptides derived from sodium caseinate hydrolysates produced by Lactobacillus helveticus NCC 2765 . Journal of Agricultural and Food Chemistry , 52 : 6923 – 6931 .
- Saito , T. , Nakamura , T. , Kitazawa , H. , Kawai , Y. and Itoh , T. 2000 . Isolation and structural analysis of antihypertensive peptides that exist naturally in Gouda cheese . Journal of Dairy Science , 83 : 1434 – 1440 .
- Yamamoto , N. , Akino , A. and Takano , T. 1994 . Antihypertensive effect of the peptides derived from casein by an extracellular proteinase from Lactobacillus helveticus CP790 . Journal of Dairy Science , 77 : 917 – 922 .
- Pripp , A.H. , Isaksson , T. , Stepaniak , L. and Sorhaug , T. 2004 . Quantitative structure-activity relationship modelling of ACE-inhibitory peptides derived from milk proteins . European Food Research and Technology , 219 : 579 – 583 .
- Gobbetti , M. , Stepaniak , L. , De Angelis , M. , Corsetti , A. and Cagno , R.D. 2002 . Latent bioactive peptides in milk proteins: Proteolytic activation and significance in dairy processing . Critical Reviews in Food Science and Nutrition , 42 : 223 – 239 .
- Meisel , H. 1997 . Biochemical properties of regulatory peptides derived from milk proteins . Biopolymers , 43 : 119 – 128 .
- Yamashita , M. , Arai , S. , Tsai , S.J. and Fujimaki , M. 1971 . Plastein reaction as a method for enhancing the sulfur-containing amino acid level of soybean protein . Journal of Agricultural and Food Chemistry , 19 : 1151 – 1154 .
- Williams , R.J.H. , Brownsell , V.L. and Andrews , A.T. 2001 . Application of the plastein reaction to mycoprotein: I. Plastein synthesis . Food Chemistry , 72 : 329 – 335 .
- Yamashita , M. , Arai , S. and Fujimaki , M. 1976 . Plastein reaction for food protein improvement . Journal of Agricultural and Food Chemistry , 24 : 1100 – 1104 .
- Combes , D. and Lozano , P. 1993 . α-Chymotrypsin in plastein synthesis. Influence of water activity . Annals of the New York Academy of Sciences , 672 (Enzyme Engineering XI), 409–414
- Andrews , A.T. and Alichanidis , E. 1990 . The plastein reaction revisited: Evidence for a purely aggregation reaction mechanism . Food Chemistry , 35 : 243 – 261 .
- Stevenson , D.E. , Morgan , K.R. , Fenton , G.A. and Moraes , G. 1999 . Use of NMR and mass spectrometry to detect and quantify protease-catalyzed peptide bond formation in complex mixtures . Enzyme and Microbial Technology , 25 : 357 – 363 .
- Ashley , D.V.M. , Temler , R. , Barclay , D. , Dormond , C.A. and Jost , R. 1983 . Amino acid-enriched plasteins: A source of limiting amino acids for the weanling rat . Journal of Nutrition , 113 : 21 – 27 .
- Sukan , G. and Andrews , A.T. 1982 . Application of the plastein reaction to caseins and to skim milk powder: II. Chemical and physical properties of the plasteins . Journal of Dairy Research , 49 : 279 – 293 .
- Wu , J.P. and Ding , X.L. 2002 . Characterization of inhibition and stability of soy-protein-derived angiotensin I-converting enzyme inhibitory peptides . Food Research International , 35 : 367 – 375 .
- Yamashita , M. , Arai , S. and Fujimaki , M. 1976 . A low-phenylalenine, high-tyrosine plastein as an acceptable dietetic food . Journal of Food Science , 41 : 1029 – 1032 .
- Ono , S. , Kasai , D. , Sugano , T. , Ohba , K. and Takahashi , K. 2004 . Production of water soluble antioxidative plastein from squid hepatopancreas . Journal of Oleo Science , 53 : 267 – 273 .
- Gao , B. and Zhao , X.H. 2012 . “ Modification of soybean protein hydrolysates by alcalase-catalyzed plastein reaction and the ACE-inhibitory activity of the modified product in vitro ” . In International Journal of Food Properties 15, 982–996.
- Li , Y.Y. , Li , T.J. and Zhao , X.H. 2010 . Preparation of Alcalase-catalyzed casein plasteins in the presence of proline addition and the ACE-inhibitory activity of the plasteins in vitro . European Food Research and Technology , 231 : 197 – 207 .
- Zhao , X.H. and Li , Y.Y. 2009 . An approach to improve ACE-inhibitory activity of casein hydrolysates with plastein reaction catalyzed by Alcalase . European Food Research and Technology , 229 : 795 – 805 .
- Sarath , G. , De La Motte , R.S. and Wagner , F.W. 1989 . “ Protease assay methods ” . In Proteolytic Enzymes, A Practical Approach , Edited by: Beynon , R.J. and Bond , J.S. 25 – 55 . Oxford , , UK : IRL Press .
- IDF. Milk. Determination of the nitrogen (Kjeldahl method) and calculation of the crude protein content, IDF Standard 20B . 1993 . International Dairy Federation: Brussels, Belgium
- Church , F.C. , Swaisgood , H.E. , Porter , D.H. and Catignani , G.L. 1983 . Spectrophotometric assay using o-phthaldialdehyde for determination of proteolysis in milk and isolated milk proteins . Journal of Dairy Science , 66 : 1219 – 1227 .
- Spellman , D. , McEvoy , E. , O'Cuinn , G. and FitzGerald , R.J. 2003 . Proteinase and exopeptidase hydrolysis of whey protein: Comparison of the TNBS, OPA and pH stat methods for quantification of degree of hydrolysis . International Dairy Journal , 13 : 447 – 453 .
- Adler-Nissen , J. 1979 . Determination of the degree of hydrolysis of food protein hydrolysates by trinitrobenzenesulfonic acid . Journal of Agricultural and Food Chemistry , 27 : 1256 – 1261 .
- Murray , B.A. , Walsh , D.J. and FitzGerald , R.J. 2004 . Modification of the furanacryloyl- L-phenylalanyl-glycylglycine assay for determination of angiotensin-I-converting enzyme inhibitory activity . Journal of Biochemical and Biophysical Methods , 59 : 127 – 137 .
- Shalaby , S.M. , Zakora , M. and Otte , J. 2006 . Performance of two commonly used angiotensin-converting enzyme inhibition assays using FA-PGG and HHL as substrates . Journal of Dairy Research , 73 : 178 – 186 .
- Chu , K.T. and Ng , T.B. 2004 . First report of a glutamine-rich antifungal peptide with immunomodulatory and antiproliferative activities from family Amaryllidaceae . Biochemical and Biophysical Research Communications , 325 : 167 – 173 .
- Korhonen , H. 2009 . Milk-derived bioactive peptides: From science to applications . Journal of Functional Foods , 1 : 177 – 187 .
- Cheung , H.S. , Wang , F.L. , Ondetti , M.A. , Sabo , E.F. and Cushman , D.W. 1980 . Binding of peptide substrates and inhibitors of the angiotensin-converting enzyme . The Journal of Biological Chemistry , 255 : 401 – 407 .
- Kunst , T. 2003 . “ Protein modification to optimize functionality: Protein hydrolysates ” . In Handbook of Food Enzymology , Edited by: Whitaker , J.R. , Voragen , A.F.J. and Wong , D.W.S. 221 – 236 . New York , NY : Marcel Dekker Inc .
- Sukan , G. and Andrews , A.T. 1982 . Application of the plastein reaction to caseins and to skim milk powder: I. Protein hydrolysis and plastein formation . Journal of Dairy Research , 49 : 265 – 278 .
- Sukan , G. and Andrews , A.T. 1982 . Application of the plastein reaction to caseins and to skim milk powder: II. Chemical and physical properties of the plasteins . Journal of Dairy Research , 49 : 279 – 293 .
- Lozano , P. and Combes , D. 1991 . α-Chymotrypsin in plastein synthesis: influence of substrate concentration on enzyme activity . Biotechnology and Applied Biochemistry , 14 : 212 – 221 .
- Pallavicini , C. , Finley , J.W. , Stanley , W.L. and Watters , G.G. 1980 . Plastein synthesis with α-chymotrypsin immobilised on chitin . Journal of the Science of Food and Agriculture , 31 : 273 – 278 .
- Belitz , H.D. , Gorsch , W. and Schieberle , P. 2009 . “ Amino acid, peptides, proteins ” . In Food Chemistry , Edited by: Belitz , H.D. , Gorsch , W. and Schieberle , P. 83 – 87 . Berlin , Heidelberg, Germany : Springer-Verlag .