Abstract
The aim of the study was to explore texture changes of Dutch-type cheese at its different locations during 126-day ripening at the temperature common for maturation of this type of cheese (10°C). Each block of cheese was divided into 3 slices and texture properties were studied in 13 segments evenly distributed in each slice. Due to cheese brining, a significant increase of hardness and elastic component was reported especially in the edge segments. During the subsequent ripening, both hardness and elastic component of natural cheese decreased gradually in all segments and slices. Studied parameters of individual parts of cheese did not demonstrate uniform rate of changes during the ripening period. In the whole experiment (even after 126-day ripening), hardness and elastic component of the cheese in core showed lower values compared to those obtained in the edge of all slices.
INTRODUCTION
From the biochemical and microbiological points of view, cheese represents a dynamic system that undergoes substantial changes during its ripening. Ripening period and conditions vary according to the type of cheese, which results in differences in properties of individual cheeses. Major biochemical changes occurring during cheese ripening can be classified into primary and secondary processes. The former ones comprise metabolism of lactose, lactate, citrate, lipolysis, and proteolysis. The consequent secondary biochemical processes that are important for production of sensory active compounds include metabolism of free fatty acids and free amino acids.[Citation1–4
Not only final cheese aroma, but also its texture properties (key factors important for consumers during purchase) develop during the process of ripening. Interactions of casein particles, their swelling, extent of proteolysis of cheese matrix, association of calcium with caseins (or, more precisely, with products of their hydrolysis), pH changes, and also changes of number of hydrogen bridges in matrix influence proper development of texture properties during cheese ripening.Citation[2,Citation4–8 Different ripening conditions, for example, air moisture, temperature, and length of ripening, can cause change of intensity of the above processes and thereby cause a change of texture properties of ripening cheese.Citation[4] Cheese texture is also affected by moisture and protein contents. Hardness of products increase usually with decreasing moisture content and increasing content of casein proteins.Citation[6]
Buňková et al.,Citation[9] Komprda et al.,Citation[10] Novella-Rodrígues et al.,Citation[11] and Pachlová et al.Citation[12] found that microbiological and biochemical changes might not demonstrate the same intensity in all parts of a cheese block. Especially moisture content, water activity (aw), salt concentration, and salt to moisture ratio can play a significant role. The above factors may influence activity of enzymes and thereby also texture properties of products.Citation[13,Citation14] Details on change of texture parameters in individual parts of natural cheese during ripening have not been reported satisfactorily in available literature.
Cheese represents a viscoelastic material combining properties of both elastic and viscous matter and shows an ability to absorb mechanical vibrations.Citation[15] Food texture can be sufficiently analyzed by instrumental methods, for example by relaxation tests. During a relaxation test, material is deformed using compression; a plate or probe is kept at the maximum force location for tens of seconds and the decline in force is recorded.[Citation15–22 The resulting force-deformation curve can be subjected to mathematical analysis using, for example, the Peleg's model;Citation[23,Citation24] its results provide information on changes of viscoelastic properties of material (i.e., on change of elastic and viscous components). The relaxation test and, subsequently, Peleg's model have successfully been applied for monitoring of viscoelastic properties of many food matrixes; processed cheese, dough, rainbow trout, cods or hot-air-puffed amaranth seeds might serve as examples.[Citation15,Citation22,Citation25–28
The aim of the study was to explore texture properties of individual parts of Dutch-type cheese via hardness and relaxation tests during 126-day ripening.
MATERIALS AND METHODS
Samples
Fourteen blocks of Dutch-type cheese (Edam cheese, similar to Gouda with a few small eyes; 50% w/w dry matter and 30% w/w fat in dry matter; average weight 1.42 ± 0.17 kg; approx. 90 mm in height, 90 mm in width, and 140 mm in depth) were taken from a batch manufactured within common production of a Czech producer of cheeses made from pasteurized milk. The manufacture protocol used by Pachlová et al.Citation[12] was followed except for the shape of the cheese blocks (). During the first day, the cheeses were manufactured, pressed, and placed into brine. On the second day, the blocks were taken out from the brine, packed into Cryovac packaging, and put into a ripening cellar with a temperature 10 ± 2°C. The cheeses were sampled on the following days: 1st (before the placement into brine), 4th, 7th, 14th, 42nd, 84th, and 126th. Two parallel cheese blocks were taken for textural analyses. Three batches were sampled for statistical purposes.
Figure 1 Sampling procedure of tested cheese. (a) A diagram depicting sampling of three slices—S1, S2, and S3—and division of each slice into three layers (the dimensions are indicated in millimeters). (b) Thirteen segments of individual cheese slices tested by relaxation test.
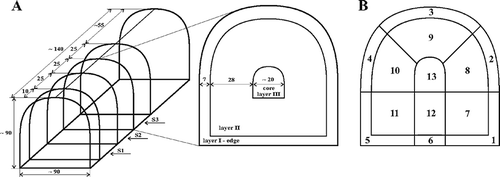
A 10-mm slice was cut off each block (the slice was not used for analysis) and three slices referred to as S1, S2, and S3 (the central slice) were taken for testing subsequently (approx. 90 mm in height, 90 mm in width, and each 25 mm in depth). Each tested slice was divided into three layers: 7 mm from the edge (referred to as layer I); the next 28 mm (referred to as layer II); and the remaining core (referred to as layer III). shows a diagram illustrating the sampling procedure. The layers I and II were further divided into six segments subjected to texture analysis (see and the section “Texture Analysis”).
The pH-values of different segments of slices were measured by a calibrated WP pH-meter Spearm Oakton (equipped with a small measuring electrode—diameter of approx. 3 mm; Eutech Instruments, Oakton, Malaysia) at 21 ± 2°C. Each segment was measured three times.
Texture Analysis
Prior to texture analysis, the tested slices were wrapped with plastic foil because of water loss prevention and they were tempered in the chamber at 20°C for 3 h. A relaxation test using a Texture Analyser TA.XTPlus (with a compression cell in capacity of 30 kg) (Stable Micro Systems, Surrey, UK) was carried out. Samples were compressed by 5 mm with a spherical probe of 5 mm diameter at the speed of 1 mm·s−1 (trigger force of 5 g), then the probe was kept at the maximum force position for 30 s and the decline in force was recorded. Subsequently, the probe was returned to the start position (speed of 1 mm·s−1). The probe was successfully used to prevent sample rupture, no sample was ruptured. Thirteen locations on all three slices were analyzed using the relaxation test: six segments in layer I; six segments in layer II; and a segment in the cheese core (; the side nearer to the removed 10-mm belt was analyzed in all three slices). Dependence of Ft on time t is expressed as a force-deformation curve in . Maximum force F max (N), which serves as an indicator of hardness, was acquired by analysis of the above curve.Citation[5,Citation18]
Figure 2 A force-deformation curve (the dependence of power Ft on time t) acquired during a relaxation test.
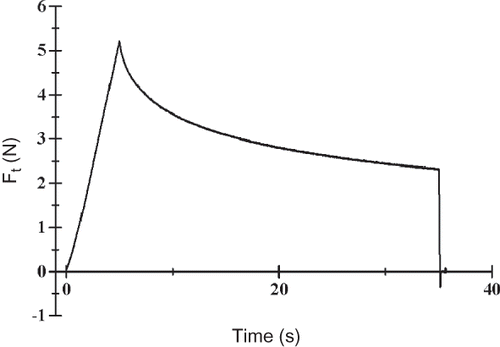
Data obtained from the relaxation test were subjected to further analysis and thereby the viscoelastic properties of tested samples could be estimated. Only the part of the force-deformation curve between the maximum force (F max) point and the start of the probe withdrawal from the sample (about 30 s of analysis, and ) was analyzed and subjected to calculation according to the Peleg's model.Citation[23,Citation24] At first, the values of Ft forces at time t were normalized according to EquationEq. (1). The normalized force-deformation curve () was linearized () according to EquationEq. (2) afterwards. Its right member represents a regression curve, whose parameters k 1 and k 2 were estimated by the non-linear regression analysis (Marquardt-Levenburg method). Special attention was paid to the parameter k 2 that indicates the extent of material relaxation. The k 2 = 1 values respond to material with ideal viscosity; the higher the values of k 2, the higher the proportion of elastic component. The k 1 parameter is an indicator of the initial rate of decrease of the mechanical stress imposed on the sample (initial rate of relaxation). High values of the parameter k 1 indicate slow relaxation and, hence, high proportion of elastic component of the sample.Citation[17,Citation19,Citation24]
Figure 3 Transformations carried out by calculations according to the Peleg's model: (a) an example of a force-relaxation curve acquired by testing a segment of a cheese slice; (b) the above relaxation curve normalized (Yt ) by the maximum force recorded (F max); (c) linear fit of the expression t/Yt using the Peleg's equation t/Yt = k 2 t + k 1.
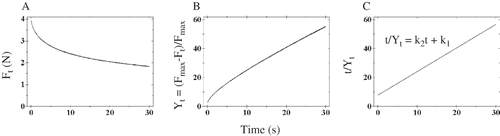
The values of hardness (F max) and parameters k 1 and k 2 were subjected to one-way analysis of variance (ANOVA; separately for each factor studied—the effect of different location of segments, slices, and ripening period) and to the Duncan test. The relation between hardness and the parameters k 1 and k 2 was evaluated by the means of Pearson's correlation coefficient (r) subsequently. The Unistat 5.5 statistical software (Unistat Ltd., London, UK) was used to evaluate results of experiments.
RESULTS AND DISCUSSION
Before brining on the 1st day, the initial pH values ranged between 5.31–5.38 in all segments and slices. During the next 6 days, values of pH decreased to 5.09–5.20 (P < 0.05) and then they rose continuously and slowly (P < 0.05) from the 7th till 126th day. On the 126th day, cheese pH reached values of 5.52–5.60 regardless of the segments and/or slices tested. During the whole experiment, the pH values of individual segments and slices changed in a similar way (P ≥ 0.05).
Changes of cheese hardness in individual segments of three tested slices are shown in . On the day of natural cheese production (1st day; before brining), its hardness in individual segments of all slices was similar and ranged between 4–5 N (P ≥ 0.05).
Figure 4 The changes of hardness (N) for each of 13 segments of the slices S1, S2, and S3 tested during 126-day ripening at 10°C (mean: n = 6).
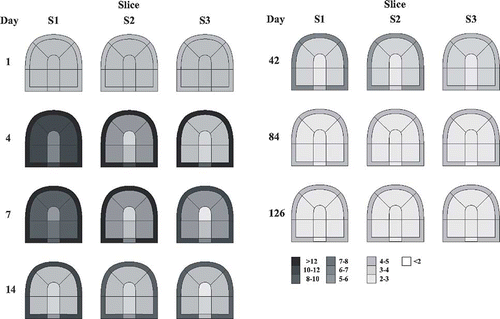
On the 4th day post manufacture, when the cheeses were brined up, hardness in the segments 1–5 of all the tested slices increased substantially (P < 0.001) exceeding values of 12 N. No significant differences between the segments 1–5 of all the tested slices (P ≥ 0.05) were detected. Hardness in the segments 7–11 of the slice S1 situated closely to the frontal margin rose significantly and reached values between 10–11 N (P < 0.001). Relevant segments of the slice S2 showed also a significant growth (P < 0.01), but their hardness ranged between 5–6 N only. A significant increase of cheese hardness in the segments 7–11 of the slice S3 was not observed (P ≥ 0.05). On the 4th day, the segments 6 of all tested slices and the segments 12 and 13 (core) of the slice S1 demonstrated higher hardness (P < 0.001); however, cheese in these segments was softer than in the other parts of the corresponding layers I and II (P < 0.01). Hardness of cheese in segment 12 of the slice S2 did not show a significant change (P ≥ 0.05) during the first 4 days. Hardness in segment 13 (core) of the slices S2 and S3 and in segment 12 of the slice S3 demonstrated even decrease to 3–4 N (P < 0.05).
During the next 3 days, hardness of cheese in the segments 1–5 of the slices S1 and S2 and also in the segments 7–12 of the slice S2 did not change significantly (P ≥ 0.05). The core of the slice S1 and segment 6 of the slices S1 and S2 showed a decrease of hardness (P < 0.01). Compared to values acquired on the 4th day, hardness of cheese in segment 12 of the slice S1 grew to 7–8 N (P < 0.05). The segments 1–6 and 13 (core) of the slice S3 demonstrated a decrease (P < 0.05). On the contrary, hardness in the segments 7–12 showed growth (P < 0.05).
During the second week of ripening (between the 7th and 14th days), cheese hardness in all the segments and slices decreased (P < 0.05); only the core of the slices S2 and S3 did not show significant changes (P ≥ 0.05). Hardness in segments 1–5 of the slice S1 and both S2 and S3 slices dropped to 10–12 N and 8–11 N, respectively (P < 0.05). The segments 6–12 of all tested slices showed a decline of hardness (P < 0.01).
Between the 14th and 42nd day, the values of hardness continued decreasing in most segments and slices (P < 0.05), specifically hardness in the segments 1–5 of the slices S1, S2, and S3 ranged between 6–7 N, 5–6 N, and 4–5 N, respectively. Decrease to 3–4 N was observed in the segments 7–11 of all tested slices (P < 0.01). The core of the slices S1 and S2 showed decline of hardness (P < 0.05). As between the 7th and 14th days, changes of hardness in the S3 core were not significant (P ≥ 0.05). Since the 84th day, cheese hardness in the segments 1–5 stabilized in the range of 4–5 N and it steadied down to 2–3 N in the segments 6–13. The above mentioned values did not show significant changes in comparison with those acquired on the 126th day (P ≥ 0.05) of experiment.
lists values of the k 1 and k 2 coefficients calculated by the non-linear regression method using the Peleg's model. Comparing the 1st and 4th days' values of the parameter k 2, a significant increase of cheese elasticity in all segments and slices (P < 0.05) as the result of brining emerges clearly. In the following days of ripening, natural cheese segments of slices demonstrated a gradual drop of elastic component (P < 0.05). The above drop was observed (P < 0.05) also between the 84th and 126th days, when hardness values did not demonstrate significant changes (see above). The segments in the layer I (1–5) of individual slices demonstrated higher values of elasticity (P < 0.05) in comparison with the other segments during the whole ripening experiment. Cheese core showed a lower elastic component (P < 0.05) than the other segments (with the exception of the first day). Similarly to hardness, elastic component in the segments 6 and 12 was significantly lower (P < 0.05) than in other segments of the particular layers I and II. Analysis of variance (ANOVA) showed differences in changes of elasticity in individual slices and segments during ripening (P < 0.05). The dependence between k 2 and hardness values assessed by Pearson correlation coefficient was significant (r = 0.7662; P < 0.01). The above mentioned findings are in accordance with the works of PelegCitation[23,Citation24] and Markowski et al.Citation[28], who stated that the Peleg's model and especially the parameter k 2 are suitable for characterization of the viscoelastic properties of food.
Table 1 The parameters k 1 and k 2 calculated from the force-deformation curves of 13 segments (n = 6; mean ± SD) of individual cheese slices (S1, S2, and S3) using the Peleg's model.Footnote*
On the other hand, the parameter k 1 did not describe the changes in viscoelastic properties of individual cheese segments satisfactorily (). For example, most of k 1 values of the segments 1–5 (in all slices) decreased between the 1st and 4th day though the cheese brining had finished and the elasticity of the above mentioned segments was generally supposed to increase (see below). During ripening, many k 1 values obtained in individual segments of the slices S1, S2, and S3 varied without showing any unambiguous trend. The Pearson correlation coefficients between the parameter k 1 and hardness values or values of the parameter k 2 are relatively small (r = 0.4888 and r = 0.3719, respectively). The authors' findings correspond with the opinion of Hatcher et al.,Citation[17] Peleg,Citation[23] and Singh et al.,Citation[19] who reported difficult and ambiguous interpretation of the parameter k 1. According to Peleg,Citation[23] the parameter k 1 is affected rather by other factors, for example, by experimental errors and shape changes than by the parameter k 2.
Prior to insertion of cheese blocks into brine, hardness in individual segments and slices had shown balanced values. However, the parameter k 2 (indicator of the elastic component of material) suggests a slightly higher elastic component in the segments 1–5, which can be related to the process of pressing and to moderate heterogeneity of material in the layer I immediately after pressing.Citation[3] During brining, a substantial growth of hardness and also of the parameter k 2 caused by diffusion of NaCl into cheese was reported in the segments directly exposed to brine (especially the segments 1–5 of all tested slices). In comparison with the relevant segments of the slices S2 and S3, the segments 6–13 of the slice S1 showed significantly higher hardness and elastic component. The above can be explained by faster diffusion of NaCl into the slice S1, because salt did not penetrate only from the edge of cheese (layer I), but also from the front side of the block situated most closely to the slice S1, which is in accord with Fox et al.Citation[3] and also Chevanan and Muthukumarappan.Citation[29] They state that NaCl content and the salt to moisture ratio exhibit a significant effect on texture properties of cheese. The following two basic processes influencing texture properties of cheese can be expected during the ripening experiment: (i) diffusion of NaCl (hardness and elastic component increase with the growing content of NaCl and vice versa); and (ii) proteolytic changes of casein proteins causing decrease of hardness and elastic component. Intensity of proteolytic changes in individual parts of cheese then results especially from different microenvironmental conditions for metabolism of lactic acid bacteria, or more precisely, from conditions for activity of particular enzymes.Citation[1,Citation3,Citation5,Citation12,Citation14] In the first 7–14 days of the experiment, NaCl diffused intensively into the layers II and III of individual slices (in the direction of concentration gradient), which led to a decrease of hardness and elastic component in the layer I and, conversely, to an increase of these parameters in the layers II and III. The core of the slice S3 experiencing slower diffusion of NaCl represented certain exception. Texture parameters of the above core were influenced rather by proteolytic reactions during the first days of the experiment. However, a continuous decrease of hardness and elastic component could be observed since the 14th day of the experiment. According to Pachlová et al.,Citation[12] who studied changes of free amino acid content in layers of natural cheeses, the above is caused by proteolytic processes. This finding is reported also by other authors, for example, by Chevanan and Muthukumarappan,Citation[29] Delgado et al.,Citation[5] and Topçu and Saldamli.Citation[14] Proteolytic processes and subsequent reaction of free amino acids during ripening are generally associated with increase of pH (especially due to production of ammonia and other alkaline substances),Citation[3,Citation12] which was observed also in the authors' experiments. Unfortunately, changes of pH-values could not cause differences in development of cheese texture in individual segments, because their pH values did not differ (in given days).
Compared to the layers II and III, significantly higher values of hardness and elastic component in the layer I (segments 1–5) were reported in all tested slices even after 126 days of ripening. According to Pachlová et al.,Citation[12] the above is caused mostly by slightly higher content of dry matter in the layer I (higher by circa 2% w/w). The above authors have reported that even after 168 days of ripening the dry matter content in the edge and in the other parts of the cheese did not equalize.Citation[12] That phenomenon has not been explained. Fox et al.Citation[3] pointed out possible interactions of the outer layers of cheese, packaging materials, and environmental conditions.
Interesting changes were found in the segment 6 (and by the 42nd day also in the segment 12) of the tested slices. Their hardness and the k 2 elastic component showed significantly lower values than in the other segments of the layer I, or the layer II (the segment 12). Satisfactory information explaining the above phenomenon has not been found in the literature. The above findings can be elucidated by the fact that cheese blocks were put on a board when salted in brine. Sodium chloride could not penetrate into the segment 6 straight (this area was not in direct contact with the brine), but it could only diffuse from adjacent areas represented especially by the segments 1 and 5 (). Thus, possible lower content of NaCl can influence growth and metabolism of lactic acid bacteria and also affect proteolytic processes.Citation9–12
CONCLUSION
Texture parameters of individual parts of cheese did not demonstrate uniform changes. Their development in individual slices and layers differed. Even after 126 days of ripening, texture parameters of Dutch-type cheese did not equalize completely. Edge parts remained harder than the other parts of the cheese. Diffusion of NaCl and intensity of proteolytic reactions probably rank among the main factors influencing texture properties. Besides monitoring the standard parameter of hardness, the use of relaxation tests with analysis of force-deformation curve is recommended. The above analysis can provide detailed information on changes of texture parameters.
ACKNOWLEDGEMENT
This study was supported by the project of the Ministry of Education, Youth and Sports of the Czech Republic (MSM7088352101).
REFERENCES
- McSweeney , P.L.H. 2004 . Biochemistry of cheese ripening . International Journal of Dairy Technology , 57 : 127 – 144 .
- Sousa , M.J. , Ardö , Y. and McSweeney , P.L.H. 2001 . Advance in the study of proteolysis during cheese ripening . International Dairy Journal , 11 : 327 – 345 .
- Fox , P.F. , Guinee , T.P. , Cogan , T.M. and McSweeney , P.L.H. 2000 . Fundamentals of Cheese Science , 638 Gaithersburg , , MD, USA : Aspen Publication .
- Forde , A. and Fitzgerald , G.F. 2000 . Biotechnological approaches to the understanding and improvement of mature cheese flavour . Current Opinion in Biotechnology , 11 : 484 – 489 .
- Delgado , F.J. , Rodríguez-Pinilla , J. , González-Crespo , J. , Ramírez , R. and Roa , I. 2010 . Proteolysis and texture changes of a Spanish soft cheese (‘Torta del Casar’) manufactured with raw milk and vegetable rennet during ripening . International Journal of Food Science and Technology , 45 : 512 – 519 .
- Pinho , O. , Mendes , E. , Alves , M.M. and Ferreira , I.M.P.L.V.O. 2004 . Chemical, physical, and sensorial characteristics of “Terrincho” ewe cheese: Changes ripening and intravarietal composition . Journal of Dairy Science , 87 : 249 – 257 .
- Lucey , J.A. , Johnson , M.E. and Horne , D.S. 2003 . Perspectives on the basic of the rheology and texture properties of cheese . Journal of Dairy Science , 86 : 2725 – 2743 .
- Lawrence , R.C. , Creamer , L.K. and Gilles , J. 1987 . Cheese ripening technology . Journal of Dairy Science , 70 : 1748 – 1760 .
- Buňková , L. , Buňka , F. , Mantlová , G. , Čablová , A. , Sedláček , I. , Švec , P. , Pachlová , V. and Kráčmar , S. 2010 . The effect of ripening and storage conditions on the distribution of tyramine, putrescine and cadaverine in Edam-cheese . Food Microbiology , 27 : 880 – 888 .
- Komprda , T. , Smělá , D. , Novická , K. , Kalhotka , L. , Šustová , K. and Pechová , P. 2007 . Content and distribution of biogenic amines in Dutch-type hard cheese . Food Chemistry , 102 : 129 – 137 .
- Novella-Rodrígues , S. , Veciana-Nogués , M.T. , Izquerdo-Pulido , M. and Vidal-Carou , M.C. 2003 . Distribution of biogenic amines and polyamines in cheese . Journal of Food Science , 68 : 750 – 755 .
- Pachlová , V. , Buňka , F. , Buňková , L. , Weiserová , E. , Budinský , P. , Žaludek , M. and Kráčmar , S. 2011 . The effect of three different ripening/storage conditions on distribution of selected parameters in individual parts of Dutch-type cheese . International Journal of Food Science and Technology , 46 : 101 – 108 .
- Floury , J. , Camier , B. , Rousseau , F. , Lopez , C. and Tissier , J.P. 2009 . Reducing salt level in food: Part 1. Factors affecting the manufacture of model cheese systems and their structure-texture relantionships . LWT–Food Science and Technology , 42 : 1611 – 1620 .
- Topçu , A. and Saldamli , I. 2006 . Proteolytical, chemical, textural and sensorial changes during the ripening of Turkish white cheese made of pasteurized cow's milk . International Journal of Food Properties , 9 : 665 – 678 .
- Herrero , A.M. , Heia , K. and Careche , M. 2004 . Stress relaxation test for monitoring post mortem textural changes of ice-stored cod (Gadus marhua L.) . Journal of Food Science , 69 : 178 – 182 .
- Alvis , A. , Villamiel , M. and Rada-Mendoza , M. 2010 . Mechanical properties and viscoelastic charakteristics of two varieties of yam tubers (Dioscorea alata) . Journal of Texture Studies , 41 : 92 – 99 .
- Hatcher , D.W. , Bellido , G.G. , Dexter , J.E. , Anderson , M.J. and Fu , B.X. 2008 . Investigation of uniaxial stress relaxation parameters to characterize the texture of yellow alkaline noodles made from durum and common wheats . Journal of Texture Studies , 39 : 695 – 708 .
- Sirisomboon , P. , Tanaka , M. , Akinaga , T. and Kojima , T. 2000 . Evaluation of the textural properties of Japanese pear . Journal of Texture Studies , 31 : 665 – 677 .
- Singh , H. , Rockall , A. , Martin , C.R. , Chung , O.K. and Lookhart , G.L. 2006 . The analysis of stress relaxation data of some viscoelastic foods using a texture analyzer . Journal of Texture Studies , 37 : 383 – 392 .
- Blahovec , J. and Esmir , A.A.S. 2001 . Stress relaxation in cooked potato tubers expressed by improved rate controlled model . International Journal of Food Properties , 4 : 485 – 499 .
- Joshi , N.S. , Jhala , R.P. , Muthukumarappan , K. , Acharya , M.R. and Mistry , V.V. 2004 . Textural and rheological properties of processed cheese . International Journal of Food Properties , 7 : 519 – 530 .
- Zaidul , I.S.M. , Karim , A.A. , Manan , D.M.A. , Azlan , A. , Norulaini , N.A.N and Omar , A.K.M. 2003 . Stress relaxation test for sago-wheat mixtures gel . International Journal of Food Properties , 6 : 431 – 442 .
- Peleg , M. 1979 . Characterization of the stress relaxation curves of solid foods . Journal of Food Science , 44 : 277 – 281 .
- Peleg , M. 1980 . Linearization of relaxation and creep curves of solid biological materials . Journal of Rheology , 24 : 451 – 463 .
- Dimitreli , G. and Thomareis , A.S. 2009 . Instrumental textural and viscoelastic properties of processed cheese as affected by emulsifying salts and in relation to its apparent viscosity . International Journal of Food Properties , 12 : 261 – 275 .
- Rodríguez-Sandoval , E. , Fernández-Quintero , A. , Sandoval-Aldana , A. and Quicazán , M.C. 2008 . Effect of cooking time and storage temperature on the textural properties of cassava dough . Journal of Texture Studies , 39 : 68 – 82 .
- Michalczyk , M. and Surówka , K. 2009 . Microstructure and instrumentally measured textural changes of rainbow trout (Oncorhynchus mykiss) gravads during production and storage . Journal of the Science of Food and Agriculture , 89 : 1942 – 1949 .
- Markowski , M. , Ratajski , A. , Konopko , H. , Zapotoczny , P. and Majewska , K. 2006 . Rheological behavior of hot-air-puffed amaranth seeds . International Journal of Food Properties , 9 : 195 – 203 .
- Chevanan , N. and Muthukumarappan , K. 2008 . Viscoelastic properties of cheddar cheese: Effect of calcium and phosphorus, residual lactose, salt-to-moisture ratio and ripening time . International Journal of Food Properties , 11 : 624 – 637 .