Abstract
An exopolysaccharide-producing strain of Streptococcus thermophilus was evaluated in the production of Mexican manchego-type cheese. This ropy strain improved water and fat retention, and significantly increased cheese yield. Furthermore, the ropy strain cheese retained more moisture than control cheese during ripening, suggesting that exopolysaccharide strongly bound water within the protein matrix of the cheese. Scanning electron microscopy confirmed that exopolysaccharide bound to the protein matrix of the cheese, producing a dense network that helped to increase water and fat retention and leading to a more open structure of the cheese that gave a softer product, as confirmed by instrumental texture profile analysis and sensory evaluation. Comparison of scanning electron microscopy micrographs of the different sections of the cheese showed higher concentration of exopolysaccharide in the centre than in the outer sections, indicating that exopolysaccharide production continued during ripening and that the environment at the centre of the cheese (moisture and/or oxygen concentration) favoured exopolysaccharide production. Instrumental texture profile analysis also demonstrated that the ropy strain cheese was more cohesive and less elastic than the control; in contrast, exopolysaccharide did not affect chewiness. The changes in texture could be correlated to composition: hardness increased as water and fat decreased, while springiness decreased with increasing fat. The interactions of exopolysaccharide with the cheese protein matrix had an affect on the increase in cohesiveness of the ropy strain cheese.
INTRODUCTION
Exopolysaccharide (EPS)-producing lactic acid bacteria (LAB) have been used in the production of some dairy products. Most studies that have used this kind of bacteria have been performed in yoghurt, where it has been shown to increase viscosity or decrease product syneresis.[Citation1] These characteristics could be useful in generating other dairy products, such as cheese. Among the studies involving cheese, it has been reported that the use of a capsule-forming strain produced microstructural changes in the curd of mozzarella cheese, eliciting a more open casein network with larger pore sizes and softer texture than control cheese.[Citation2] Other investigations have reported that the use of a capsule-forming strain of Streptococcus thermophilus in mozzarella cheese production increases its moisture content, hence improving its melting properties.[Citation3–Citation5] Besides mozzarella, other types of cheese using EPS-producing cultures include feta,[Citation6] in which non-fat cheese made with a non-capsule-forming culture have a more compact structure compared to that made with a capsule-forming culture; karish, an Egyptian acid-coagulated cheese;[Citation7] half-fat cheddar;[Citation8] and panela, a fresh Mexican cheese.[Citation9] In all cases, textural modifications were observed. However, to the best of our knowledge, very little work has been undertaken with full fat cheese, where the fat content determines characteristics, such as flavour and texture. The influence of EPS on fat retention, yield improvement and textural properties has not been widely assessed.
Most types of Mexican cheese are fresh, similar to most fresh white Latin American cheeses. However, about 20% of the Mexican cheese market is composed of ripened cheese, the main representatives being the manchego-type and Chihuahua. These are very similar: slightly ripened with a semi-hard texture and usually made with a combination of mesophilic and thermophilic cultures. The yield achieved for slightly ripened cheese is lower than that of fresh types of cheese, which makes them less competitive in the Mexican market. Thus, anything that could help increase yield without diminishing its taste and quality would increase the competitiveness of these products. The aim of the current study was to evaluate the use of Streptococcus thermophilus SY-102, an EPS-producing strain, on the yield and fat retention and some physical attributes of Mexican manchego-type cheese.
MATERIALS AND METHODS
Milk
Fluid pasteurised and homogenised whole cow’s milk containing 30 g·L−1 fat, 31 g·L−1 protein, and a density of 1.032 g·mL−1 (Santa Clara, Productos Lácteos S.A. de C.V., Mexico City, Mexico) was used to produce cheese.
Starter Cultures
The ropy strain cheese was developed with a commercial mixed culture of Lactococcus lactis subsp. lactis and Lactococcus lactis subsp. cremoris (Rhône-Poulenc de Mexico, Mexico City, Mexico) plus the ropy strain Streptococcus thermophilus SY-102 (Rhodia-Texel Ltd., London, UK). The milk for cheese production was inoculated with 2% of the mesophilic cultures plus 0.5% of the S. thermophilus culture. The control cheese was produced with 2% of the mesophilic cultures, but the EPS-producing S. thermophilus strain was replaced with the non-ropy strain Streptococcus thermophilus TA050 (Rhône-Poulenc de Mexico). Starter cultures were prepared by incubating at either 37°C (mesophilic) or 42°C (S. thermophilus) for 18 h in skimmed milk with 10% total solids (Svelty, Nestlé, Mexico City, Mexico), sterilised at 121°C for 5 min.
Cheese Making
The cheese was prepared in triplicate batches in bench cheese vats with 3.5 L of milk and 0.2 mL·L−1 of 0.3% calcium chloride solution (w/w). The milk was incubated at 37°C using 2% of the corresponding starter mixture culture with constant measurements (every 30 min) of viscosity, acidity, and pH until pH reached a value of 6.0. The milk was then clotted using microbial rennet (3.5 mL, strength 1:10000; Cuamex, Mexico City, Mexico) and incubated for approximately 30 min. After coagulation, the curd was cut into cubes of approximately 1 cm per side; the temperature was slowly increased (1°C min−1) up to 45°C ± 2°C, while the curd was gently stirred. Once the temperature was reached, most of the whey (approximately 80% of volume) was drained and the curd was kept in the vat flooded as small blocks in the remaining whey, turning the pieces periodically until the lactic acid in the whey reached 0.20 to 0.22%. The cheese was moulded into 1 kg round moulds and pressed for 24 h at a constant pressure of 1 kg·cm−2. After pressing, the cheeses were submerged in 10% NaCl brine and allowed to salt for 24 h at 10°C. The cheese was ripened in a chamber (10 to 12°C, 80 to 90% relative humidity) for 2 weeks, turning the cheeses every third day and cleaning the surface with a 0.2% solution of potassium sorbate.
Physico-Chemical Analyses
Milk apparent viscosity was analysed by means of a Brookfield LV-DV III viscometer (Brookfield Engineering Laboratories Inc., Stoughton, MA, USA). Readings were taken with LV4 or ULA spindles at speeds of 40 and 60 rpm, respectively, every 30 min up to 180 min. Milk and whey acidity were determined by titration with 0.1 mol·L−1 NaOH (Sigma Chemicals, Mexico City, Mexico), using phenolphthalein as an indicator. The pH was determined with a pH-meter Conductronic pH 20 (Conductronic, Mexico City, Mexico). The moisture content of cheese was analysed using 1 g of sample dried at 100°C to constant weight; total solids were calculated by difference of total weight and moisture. Fat content was determined using the Gerber method. Thereafter, fat in dry weight bases and total solids were calculated.[Citation9] Total protein in the cheese was determined by extraction with an 8 mol·L−1 urea solution at pH 8.0 as reported by Yong and Young.[Citation10]
Cheese yield, reported as percentage, was calculated by the ratio between the final weight of the cheese at different stages of production and the weight of the milk used for its production.
Scanning Electron Microscopy
The samples were taken with a cheese probe after 15 days of ripening from three different sections of the cheese (centre, middle, and rind). They were cut into pieces of approximately 3 × 3 × 1 mm, fixed overnight at 4°C in a 10 mL·L−1 glutaraldehyde solution (Electron Microscopy Science, Washington, DC, USA) with 5 mg·L−1 of ruthenium red to stain the EPS, followed by post fixation in 20 g·L−1 osmium tetroxide (Electron Microscopy Science). Dehydration was carried out at room temperature by an ethanol (J.T. Baker, Xalostoc, Mexico) dehydration series (10 min each in 25, 50, 75, and 99% ethanol solution, and finally three 10-min changes in 100% ethanol). Final dehydration was performed in a Samadri 780-B critical point dryer (Tousimis Research Corporation, Santa Clara, CA, USA) using CO2 as a transition medium. The specimens were mounted on aluminium scanning electron microscopy (SEM) stubs with carbon paint and coated with a layer of gold with a Sputter Coater SCD 050 (Bal-Tec, Balzers, Lietchtenstein). Specimens were viewed on a Zeiss DSM 940 digital scanning microscope (Oberkochen, Germany) at either 10 or 20 kV.
In spite of certain shrinking that could originate from sample treatment for SEM, the technique used to dehydrate the samples consisted of gradually replacing water with ethanol followed by the replacement of ethanol with CO2. After that, CO2 was quickly sublimated to leave a dry sample. This technique allows the preservation of the original structure of the sample as much as possible. Thus, we can assume that even though the microstructure of the cheese might have changed during the process, the structure observed in the micrographs is close to the original.
Instrumental Texture Profile Analysis (TPA)
Texture profile analysis was carried out in a TA.XT2i texture analyser (Stable Micro Systems, Texture Technologies Corp., White Plains, NY, USA). Cylindrical samples were obtained from the centre of 15-day ripened cheese pieces using a 0.5-inch borer. Their height was adjusted to 1.1 cm with a blade. Samples were placed into Petri dishes and kept at 20°C for 1 h; afterwards, they were compressed 60% in two cycles using the P50 probe and a crosshead speed of 120 mm·min−1; five samples of every cheese were analysed. The values of the textural characteristics, hardness, springiness, cohesiveness, and chewiness were calculated from the resulting curves by means of the equipment software (Texture Expert 1.20, Stable Micro Systems Ltd.).[Citation11]
Sensory Evaluation
A selected and trained panel of ten members was used for the sensorial texture evaluation. Selection of the panel was done using duo-trio and triangle tests to differentiate commercial cheeses based on textural attributes. The selected members of the panel were trained using duo-trio, triangle tests, and ranking multi-sample tests through several sessions. Additional sessions were done to fully define textural attribute descriptors and scales knowledge. Training sessions were done with five commercial cheeses for every attribute. To evaluate the textural attributes, the method of scaling was used by means of a 10-cm unstructured scale with references at the ends and the evaluated attributes were: hardness, springiness, cohesiveness, and chewiness. Cheese produced with either ropy or non-ropy starter cultures were evaluated by the quantitative description analysis technique. Samples were prepared by cutting cubical portions of approximately 1 cm per side, kept at room temperature for 1 h before tasting, and coded using random three-digit numbers.
Statistical Analyses
All results were analysed by analysis of variance (ANOVA) using the Statistical Analysis Software (Statistica 5.0, Stat Soft, Tulsa, OK, USA), with P < 0.05 used as the threshold for statistical significance.
RESULTS
Milk Fermentation
Results obtained for pH, acidity, and apparent viscosity during milk fermentation with either control or the ropy strain are shown in . There were no significant differences in pH (P = 0.0928) and acidity (P = 0.5835) in milk fermented with either culture during the whole fermentation process. illustrates that the apparent viscosity of milk fermented with either the control or the ropy strain remained constant and equal for both cultures during the first hour (P = 1.000 for time and P = 0.98 between cultures), and increased slightly (P = 0.0007 for time), but still with no difference between the control and the ropy culture (P = 0.98), up to 90 min of fermentation. When the pH reached a value below 6.2 (after 120 min of fermentation), the apparent viscosity of the ropy strain culture increased significantly (P < 0.02) with respect to the control, despite the fact that pH diminished equally for both cultures.
Yield and Chemical Composition of the Cheese
The yield of manchego-type cheese was significantly higher (P = 0.0003) for the ropy strain cheese than for the control. shows the cheese yield before and after salting and in the final product. The increase in yield was statistically significant (P < 0.001) at the three stages of production, demonstrating the effect of EPS. Composition analysis of the cheese is shown in , where it can be observed that water and fat contents are higher in the ropy strain cheese with a slight decrease in total protein content. shows the loss of weight of cheese during ripening, demonstrating a greater loss of water in the control cheese.
Table 1 Cheese yield
Table 2 Chemical composition of the cheese (g·100 g−1)
Microstructure
and show the microstructure by SEM of all the sections of both control and ropy strain cheese. Microscopic studies of the control cheese demonstrated no EPS production () and a three-dimensional protein matrix formed by casein micelles to which microorganisms adhered. SEM showed that in the ropy strain cheese (), the protein network had more and larger open cavities than the control (). The polymer in the ropy strain cheese could be seen as part of the network binding to the protein matrix and to the fat globules; moreover, the number of visible fat globules was higher than in the control cheese.
Figure 3 Microstructure (×5000) of three different sections of control Mexican manchego-type cheese: (A) rind, (B) medium, and (C) centre. The scale bar indicates 5 μm.
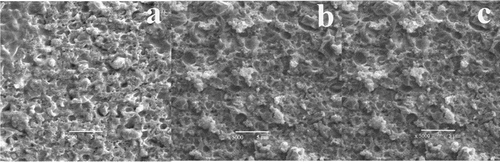
Figure 4 Microstructure (×5000) of three different sections of ropy Mexican manchego-type cheese: (A) rind, (B) medium, and (C) centre. The scale bar indicates 5 μm.
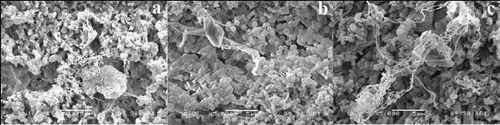
As manchego-type cheese is a pressed cheese, a different microstructure was expected across the different sections of the cheese. Due to this, for the SEM, three different sections of the cheese were observed: the centre, the middle, and the rind. The cheese microstructure was different in the different sections studied: the cavities in the protein matrix were wider in the centre than in the outer sections of the cheese, which is in agreement with the fact that the centre retained more water than the rind. Furthermore, comparison of the three sections showed that there was a higher concentration of EPS in the centre than in the rind (, , and ).
Instrumental Texture Profile Analysis (TPA)
representative TPA curves obtained for both kinds of cheeses. From these force-time graphs, the following parameters were evaluated: hardness, cohesiveness, springiness, and chewiness, which were calculated according to Bourne.[Citation11] shows the mean values of these TPA parameters and their standard deviation. Hardness and springiness were significantly lower (P < 0.0001, P = 0.0299, respectively) in the ropy strain cheese, while cohesiveness was significantly higher (P = 0.0321) and chewiness was not significantly different (P = 0.1184) between the two kinds of cheese.
Table 3 Textural characteristics of ropy and control cheese
Sensory Evaluation
The same textural attributes that were evaluated by TPA were also assessed by their sensorial appraisal by a trained panel. Results are shown in and in this case hardness and cohesiveness were significantly lower (P < 0.03) in the ropy strain cheese than in the control, while springiness was significantly higher (P = 0.0299). Chewiness, on the other hand, was not perceived as significantly different by the panellists (P = 0.3629).
DISCUSSION
Milk Fermentation
The decrease in pH together with the increase in acidity observed for both cultures () indicate that the starter cultures developed as expected. When analysing the apparent viscosity obtained with both cultures (), it was observed that it remained almost the same until a pH of 6.2 was reached. After that point, the apparent viscosity of the ropy strain culture increased significantly despite the fact that pH diminished very similarly for both cultures. The measurement of apparent viscosity () was used as an indirect indicator of EPS production. The changes in casein conformation due to acidification modify the apparent viscosity of acidified milk. Nevertheless, it is generally agreed that even though there is no straight relationship between an increase in viscosity and the concentration of EPS, when EPS is produced the viscosity of the medium increases.[Citation1,Citation12–Citation14] Therefore, the higher increase in apparent viscosity of milk fermented with the ropy culture indicates that EPS was produced.
Yield and Chemical Composition of the Cheese
The yield of manchego-type cheese at the three stages of production was higher when the ropy strain was used (P < 0.001), with an increase of 10.9% of the final product (P = 0.0003) (). Before salting, an increase of 10.8% was observed (P = 0.0002), reflecting the retention, despite the pressing procedure, of water and fat because of their interactions with the EPS. Since salting was done by submerging in brine, some water could be absorbed by the cheese to increase its weight, as seen in the control cheese, which showed a significant increase (P = 0.0468) of 4.54%. The ropy strain cheese did not show a significant increase in weight (P = 0.8765), most likely because after pressing, EPS was already holding as much water as it could and it could not absorb any more. During ripening, loss in weight occurs due to the evaporation of water. As shown in , the ropy strain cheese lost significantly less water than the control cheese (16.65% for the ropy and 19.16% for the control, P = 0.0003) as a consequence of the ability of EPS to retain moisture efficiently. An increment in yield between 13.7 and 16.8% in Mexican panela cheese has been reported when an EPS-producing culture is used.[Citation9] However, panela is a fresh cheese with high moisture content, while manchego-type is a pressed and ripened cheese. Therefore, a lower increase in the yield was expected, but the increase in the yield was statistically significant (P = 0.0003), demonstrating the effect of EPS on cheese yield. Observing the composition of the two types of cheese (), yield improvement could be related to higher moisture and fat retention in the ropy culture cheese than in the control. Similar conclusions were reported by Rynne et al.[Citation8] in half-fat cheddar cheese made with a ropy culture; they obtained higher moisture and milk fat content, and an increase in the actual cheese yield.
Total protein content () decreased slightly in the ropy strain cheese. As reported elsewhere, the ropy behaviour of EPS-producing strains might be due to a complex relationship between the exopolysaccharides and milk proteins beyond the sole production of the EPS, which has been explained partially by texture profile analysis by Dominguez-Soberanes et al.,[Citation15] who determined that the higher the protein content, the more EPS-protein interactions occur in milk, leading to enhanced texture properties of the product. However, despite protein concentration, EPS-protein interactions contributed to the textural characteristics of the product, which was observed as an increase in viscosity during milk fermentation due to the matrix formed by caseins and EPS, as suggested by Hess et al.[Citation16] and Dominguez-Soberanes et al.[Citation15] This matrix could retain more fat and water as demonstrated in the chemical analysis of the cheese ().
Microstructure
A higher water or fat content would change the cheese microstructure. To evaluate the effect of EPS on cheese microstructure, SEM was used. Microscopic studies of control cheese () demonstrated no EPS production and a three-dimensional protein matrix formed by casein micelles to which microorganisms adhered. This is in accordance with other reports.[Citation15,Citation16]
SEM of the ropy strain cheese () showed that the protein network had more and larger open cavities than the control. These cavities contained higher amounts of water, possible due to the interactions of EPS with milk components and the protein matrix of the cheese, as observed in the SEM micrographs () that show the interaction between EPS and the protein matrix leading to larger hollow spaces than in the control cheese. Data reported in confirm the higher retention of water and fat within the cheese, hence increasing the yield. These results agree with those reported by Hassan et al.[Citation6] who observed by confocal scanning laser microscopy a more open structure in feta cheese made with a capsule-forming culture of S. thermophilus. Guzel-Seydim et al.[Citation13] reported that EPS strongly bound to water within the cheese matrix, retaining it for a longer time than the control cheese. This was observed during the ripening of the cheese, in which the ropy strain cheese retained more water than the control ().
The cheese microstructure was different along the different sections studied: the cavities in the protein matrix were wider in the centre than in the outer sections of the cheese, since the centre retained more water than the rind.
Comparison of the three sections of the ropy strain cheese (, , ) demonstrated that there was a higher concentration of EPS in the centre than in the rind. It seems that the production of EPS continued during ripening and occurred more frequently in the centre where higher moisture content and an anaerobic environment was found compared to the outer sections like the rind, where moisture is lower and oxygen concentration higher. Thus, the environment influences the growth of bacteria and/or EPS production.
Instrumental Texture Profile Analysis (TPA)
The higher content of water and fat in the ropy strain cheese () contributes to a more open structure ( and ), leading to a softer cheese that is also more cohesive but less elastic (). This could be explained by the fact that higher fat and water contents tend to weaken the protein structure, resulting in a softer cheese, as has been reported by several authors.[Citation17–Citation19] Additionally, according to Chen et al.[Citation20] and Emmons et al.,[Citation21] higher fat content results in smoother and softer cheese. Furthermore, data reported by Bryant et al.[Citation22] regarding cheddar cheese with different fat contents established that the microstructure of lower-fat cheese had a more compact protein matrix with less open spaces that would have otherwise been occupied by fat globules. This is associated with a harder texture, even when the moisture content is high. These authors also showed that the open-intricate microstructure of the cheese analysed by SEM was lost, accompanied by a decrease in fat content. The same effect can be observed in and , where the ropy strain cheese shows a more open structure, with milk fat globules observed within the protein matrix. All these findings are in accordance with data reported by Lobato-Calleros et al.[Citation23] who observed that in Mexican manchego-type cheese, the reduction of fat resulted in a harder cheese due to the compact and denser larger areas of protein matrix uninterrupted by fat globules.
Moreover, it is well established that cheese with higher moisture are less firm than their lower-moisture counterparts due to the fact that the hydrophobic interactions, important for stabilising casein matrix structure, are weakened by adsorption of water by proteins.[17,24] This could contribute to the softer texture found in the ropy strain cheese. Since EPS increased water and fat contents, these factors could have primarily contributed to a softer cheese when the ropy culture was used. However, beyond the augmented content of water and fat in the ropy strain cheese, EPS and its interaction with proteins and other cheese constituents should influence the texture profile. Cohesiveness is expected to diminish as water and fat contents increase,[Citation22] but on the other hand, interactions between EPS and milk components, mainly proteins, could increase cohesiveness in the ropy strain cheese, as has been established by Dominguez-Soberanes et al.,[Citation15] who suggested that an interaction between EPS and caseins increased cohesiveness in fermented milk. In a solid product, cohesiveness represents how well its structure withstands a deformation and/or tearing apart; the higher cohesiveness of the ropy strain cheese found in this study suggests a strong interaction between EPS and caseins of the cheese matrix, in spite of the higher fat and water contents.
The results in springiness showed a less elastic ropy strain cheese than control. Bryant et al.[Citation22] reported that springiness in cheddar cheese increased with decreasing fat content, consistent with Emmons et al.[Citation21] whom established that reduced fat cheese tends to be more elastic. Based only on fat content, our results would be in agreement with those reported by Emmons et al.[Citation21] and Bryant et al.[Citation22] However, the effect of moisture content on springiness is not clear, and both increases and decreases in springiness with moisture content have been reported.[Citation24]
It is clear that the relationship between cheese composition and its texture profile is very complex, and that other factors, such as process conditions and microbiota, might also influence the texture of the product. This makes it hard to establish a straight relationship between the components and the texture profile alone, as has been reported by van Hekken et al.[Citation25] and Tunick et al.[Citation26] for Chihuahua cheese, which is similar to the Mexican manchego-type cheese.
Sensory Evaluation
Despite the fact that instrumental TPA is designed to simulate consumer perception of the physical properties of foods, human perception of the textural properties of food depends on many complex factors that the instrument does not consider on the whole while making the measurements. Because of this, it is always important to measure both instrumental and sensory perception of the texture of a product.
When comparing the results obtained in the TPA with those obtained by sensory evaluation (), a direct correlation was found for hardness and chewiness. In both cases, hardness was higher for the control than for the ropy strain cheese, which (as discussed above) could be due to the higher water and fat contents of the ropy strain cheese. There was no significant difference in the chewiness of both types of cheese. The other parameters, cohesiveness and springiness, were perceived differently by the panellists compared to the instrument.
It is clear that the same textural attributes are not necessarily perceived equally by the human senses and an instrument, even when equipments such as the texture analyser have been designed to mimic consumer texture perception. This has been pointed out by Marshall[Citation27] and Jack et al.[Citation28] The texturometer can simultaneously quantify a number of textural properties, although only hardness has been shown to relate well to data obtained from sensory analysis. Fracture patterns obtained by human actions differ markedly from those produced by instruments.[Citation17,Citation27]
In summary, the use of the exopolysaccharide-producing strain of S. thermophilus SY-102 in Mexican manchego-type cheese increased moisture and fat retention within the cheese matrix. This was reflected in the greater yield of cheese obtained. Scanning electron microscopy showed that EPS bound to the protein matrix of the cheese, microorganisms, and milk fat globules, leading to a more open structure of the cheese and producing an EPS-protein network that helped increase water and fat retention. Furthermore, interactions between EPS and water were strong enough to retain moisture during cheese ripening.
The higher water and fat contents of the ropy strain cheese was reflected in a change in the textural characteristics, hardness being the most significant and also perceived by the sensory evaluation panellists. The changes in textural characteristics were related to the changes in cheese composition: hardness increased as water and fat decreased, while springiness decreased with increasing fat. The interactions of EPS with the protein matrix of the cheese affected cohesiveness, increasing it in the ropy strain cheese. The composition of the cheese also influenced its sensory characteristics, giving a softer texture in the ropy than the control cheese. Other textural parameters, such as cohesiveness and elasticity, were perceived differently by the panellists and the texturometer.
REFERENCES
- Wacher-Rodarte, C.; Galván, M.V.; Farrés, A.; Gallardo, F.; Marshall, V.M.E.; Garcia-Garibay, M. Yogurt Production from reconstituted skim milk powders using different polymer and non-polymer forming starter cultures. Journal of Dairy Research 1993, 60, 247–254.
- Hassan, A.; Frank, J.F. Modification of microstructure and texture of rennet curd by using a capsule-forming non-ropy lactic culture. Journal of Dairy Research 1997, 64, 115–121.
- Perry, D.B.; McMahon, D.J.; Oberg, C.J. Effect of exopolysaccharide-producing cultures on moisture retention in low fat mozzarella cheese. Journal of Dairy Science 1997, 80,799–805.
- Low, D.; Ahlgren, J.A.; Horne, D.; McMahon, D.J.; Oberg, C.J.; Broadbent, J.R. Role of Streptococcus thermophiles MR-1C capsular exopolysaccharide in cheese moisture retention. Applied and Environmental Microbiology 1998, 64, 2147–2151.
- Petersen, B.L.; Dave, R.I.; McMahon, D.J.; Oberg, C.J.; Broadbent, J.R. Influence of capsular and ropy exopolysaccharide-producing Streptococcus thermophilus on mozzarella cheese and cheese whey. Journal of Dairy Science 2000, 83, 1952–1956.
- Hassan, A.N.; Frank, J.F.; Corredig, M. Microstructure of feta cheese made using different cultures as determined by confocal scanning laser microscopy. Journal of Food Science 2002, 67, 2750–2753.
- Hassan, A.N.; Corredig, M.; Frank, J.F.; Elsoda, M. Microstructure and rheology of an acid-coagulated cheese (Karish) made with an exopolysaccharide-producing Streptococcus thermophilus strain and its exopolysaccharide non-producing genetic variant. Journal of Dairy Research 2004, 71, 116–120.
- Rynne, N.M.; Beresford, T.P.; Kelly, A.L.; Tunick, M.H.; Malin, E.L.; Guinee, T.P. Effect of exopolysaccharide-producing adjunct starter cultures on the manufacture, composition and yield of half-fat cheddar cheese. Australian Journal of Dairy Technology 2007, 62, 12–18.
- Jiménez-Guzmán, J.; Flores-Nájera, A.; Cruz-Guerrero, A.E.; García-Garibay, M. Use of an exopolysaccharide-producing strain of Streptococcus thermophilus in the manufacture of Mexican panela cheese. LWT–Food Science and Technology 2009, 42, 1508–1512.
- Yong, K.J.; Young, W.P. SDS-PAGE of proteins in goat milk cheeses ripened under different conditions. Journal of Food Science 1996, 61, 490–495.
- Bourne, M.C. Food Texture and Viscosity: Concept and Measurement; Academic Press: New York, 1982.
- Broadbent, J.R.; McMahon, D.J.; Oberg, C.J.; Welker, D.L. Use of exopolysaccharide-producing cultures to improve the functionality of low fat cheese. International Dairy Journal 2001, 11, 433–439.
- Guzel-Seydim, Z.B.; Sezgin, E.; Seydim, A.C. Influences of exopolysaccharide producing cultures on the quality of plain set type yogurt. Food Control 2005, 16, 205–209.
- Champagne, C.P.; Barrette, J.; Roy, D.; Rodrigue, N. Fresh cheesemilk formulation fermented by a combination of freeze-dried citrate-positive cultures and exopolysaccharide-producing lactobacilli with liquid lactococcal starter. Food Research International 2006, 39, 651–659.
- Dominguez-Soberanes, J.; García-Garibay, M.; Casas-Alencáster, N.B.; Martínez-Padilla, L.P. Instrumental texture of set and stirred fermented milk. Effect of a ropy strain of Lactobacillus delbrueckii subsp. bulgaricus and an enriched substrate. Journal of Texture Studies 2001, 32, 205–217.
- Hess, S.J.; Roberts, R.F.; Zieglier, G. Rheological properties of non-fat yogurt stabilized using Lactobacillus delbrueckii subsp. bulgaricus producing exopolysaccharide or using commercial stabilizer systems. Journal of Dairy Science 1997, 80, 252–263.
- Jack, F.R.; Paterson, A. Texture of hard cheeses. Trends in Food Science and Technology 1992, 3, 160–164.
- Tunick, M.H.; Mackey, K.L.; Shieh, J.J.; Smith, P.W.; Cooke, P.; Malin, E.L. Rheology and microstructure of low-fat mozzarella cheese. International Dairy Journal 1993, 3, 649–662.
- Tunick, M.H.; Malin, E.L.; Smith, P.W.; Shieh, J.J.; Sullivan, B.C.; Mackey, K.L.; Holsinger, V.H. Proteolysis and rheology of low-fat and full-fat mozzarella cheeses prepared from homogenized milk. Journal of Dairy Science 1993, 76, 3621–3628.
- Chen, A.H.; Larkin, J.W.; Clark, C.J.; Irwin, W.E. Textural analysis of cheese. Journal of Dairy Science 1979, 62, 901–907.
- Emmons, D.B.; Kalab, M.; Larmond, E.; Lowrie, R.J. Milk gel structure X. Texture and microstructure in cheddar cheese made from whole milk and homogenized low-fat milk. Journal of Texture Studies 1980, 11, 15–34.
- Bryant, A.; Ustunol, Z.; Steffe, J. Texture of cheddar cheese as influenced by fat reduction. Journal of Food Science 1995, 60, 1216–1219, 1236.
- Lobato-Calleros, C.; Robles-Martínez, J.C.; Caballero-Perez, J.F.; Aguirre-Mandujano, E.; Vernon-Carter, E.J. Fat replacers in low-fat Mexican manchego cheese. Journal of Texture Studies 2001, 32, 1–14.
- Tunick, M.H.; Mackey, K.L.; Smith, P.W.; Holsinger, V.H. Effects of composition and storage on the texture of mozzarella cheese. Netherlands Milk and Dairy Journal 1991, 45, 117–125.
- Van Hekken, D.L.; Tunick, M.H.; Tomasula, P.M.; Molina-Corral, F.J.; Gardea, A.A. Mexican Queso Chihuahua: Rheology of fresh cheese. International Journal of Dairy Technology 2007, 60, 5–12.
- Tunick, M.H.; Van Hekken, D.L.; Call, J.; Molina-Corral, F.J.; Gardea, A.A. Queso Chihuahua: Effects of seasonality of cheesemilk on rheology. International Journal of Dairy Technology 2007, 60, 13–21.
- Marshall, R.J. Composition, structure, rheological properties, and sensory texture of processed cheese analogues. Journal of the Science of Food and Agriculture 1990, 50, 237–252.
- Jack, F.R.; Paterson, A.; Piggott, J.R. Relationships between rheology and composition of cheddar cheeses and texture as perceived by consumers. International Journal of Food Science and Technology 1993, 28, 293–302.