Abstract
The effect of incorporating montmorillonite nanoclay into lentil starch dispersion was studied by oscillatory rheological measurement. Rheological measurements were carried out at non-isothermal (40–95°C) and isothermal (70–90°C) condition. The elastic modulus (G′) of starch/clay blend showed a significant improvement in the magnitude in the melt when clay concentration was at 5% wt or higher. The peak complex viscosity modulus (Tη*max) decreased from 81.6 to 80.4°C with increasing clay concentration during non-isothermal heating. The empirical principle of time-temperature superposition was tested to bring experimental dynamic rheological data at selected temperatures together into single master curves at a reference temperature of 80°C. The complex viscosity (η*) master curve was found to follow the rule with slight deviation. The elastic modulus superposition (G′-ω) was found to fail in the temperature regime considered in this study. The failure of time-temperature superposition was believed to have happened due to complex starch-nanoclay-water matrix formation, temperature response of individual components, and phase change during gelatinization of starch.
INTRODUCTION
Starch has been used in different forms: neat starch, high amylose starch, modified starch, plasticized starch, etc. Starches are shaped into films by a casting method from aqueous solution. Starch-based films exhibit moderate oxygen barrier properties but poor moisture barrier and mechanical properties, which limit their wide applications.Citation[1, Citation2] The starch can be fluidized through reactive extrusion in the presence of a plasticizer (water, glycerol, etc.), which is well known as thermoplastic starch (TPS)Citation[3] and behave similar to conventional polymers. Plasticizers are used to enhance the flexibility and processability of TPS. The desired properties (mechanical, water solubility, and water absorption) of TPS can be controlled by monitoring the moisture/plasticizer content, amylose/amylopectin ratio of raw material, and the temperature and pressure in the extruder.Citation[4]
Nano-biocomposites are a new class of hybrid materials composed of nano-sized filler (nanofiller) incorporated into a bio-based matrix.Citation[5] Furthermore, the research for new materials with optimized properties and performance is an area in constant expansion. Currently, there is a considerable interest in biopolymer-clay nanocomposites due to significant property enhancements by incorporating only a few weight percent of rigid particles (nanofillers) in the nanometer range (≈100 nm). The most commonly used nanoclays include montmorillonite, a 2:1 phyllosilicate. Nanoclays have a stacked platelet structure with each platelet having a thickness of approximately 1 nm and lateral dimensions on the order of micrometers. These nanofillers have been found to improve the mechanical, barrier, electrical, gas permeability, and thermal properties of base polymer significantly at very low filler concentration with respect to their conventional microcomposite counterparts.Citation6–11 Citation Citation Citation Citation Citation11] These remarkable property enhancements make nanocomposites superior candidates for materials application in the food packaging, electronics, and automotive industries.Citation[12, Citation13] Such properties enhancements rely both on the nanofiller geometry and on its surface area, which is directly linked to the nanofiller dispersion state in a polymer matrix.Citation[14] Starch properties also improved significantly by incorporating nanoclay to starch formulations,Citation[15, Citation16] However, the relationship between mechanical behavior and nanoparticle dispersion still remains unresolved.Citation[17]
Rheology of nanocomposites provides insight of the nanoparticles-biopolymer interaction and mechanical spectra of the blend. Furthermore, the particle-particle and the particle-polymer interactions play an important role in the suspensions. In particular, the nanoparticles tend to form clusters in the absence of external forces or if the external forces are weak.Citation[18] The clusters are then responsible for certain characteristics to nanocomposites’ rheological behavior. Park et al.Citation[19] had shown that incorporating only 5 wt% nanoclay into potato starch reduced water vapor transmission rates by nearly one-half. In addition, nanoclay samples exhibited higher dynamic elastic moduli and tensile strengths. The reason for these improvements is that the nanoclays became intercalated, resulting in better dispersed platelets. In addition, the dispersed platelets provide more surface area for starch–nanoclay interactions, resulting in better reinforcement and improved mechanical properties.
Limited studies have been carried out on measuring the evolving dynamic rheological properties of starch–nanoclay composites during and after gelatinization.Citation[16, Citation20, Citation21] An understanding of the rheological properties of starch/clay blends is important for prediction of processing behavior. For multiphase blends, the empirical principle of time-temperature superposition (TTS) is mostly failed. However, the rule is applicable for miscible blends, which behaved as a single phase. These rheological properties could provide insight into starch–nanoclay interactions as well as material property changes after the gelatinization process. In this work, effect of clay concentration on oscillatory rheology of nanocay/lentil starch blend were studied at a selected temperature range (70–90°C) using the TTS principle and were compared with neat starch dispersion.
MATERIALS AND METHODS
Materials
Canadian grown red lentil (unknown variety) flour was obtained locally and used for starch isolation. Details of the sample preparation are discussed elsewhereCitation[22] and one sample lot was used throughout the study. Briefly, the lentil flour was soaked overnight in sodium hydroxide solution (0.25% w/v), and thoroughly washed with water. The slurry was passed through 125-μm screen and settled for 2 h. The supernatant was discarded and insoluble materials were suspended into absolute ethanol for an hour. The insoluble part was dried in an oven. The obtained starch was grounded and passed through #100 mesh screen aperture (0.15 mm sieve opening as per ASTME II). The starch sample contained 5.7% moisture, 0.05% ash, 0.3% protein, 0.25% lipid, and the amylose content in the starch was 43.6%.Citation[23] The organically modified montmorillonite, Cloisite 30B (30B–MMT) was supplied by Southern Clay Products (TX, USA) and has 90 mequival. of ammonium ions/100 g clay.
Sample Preparations
The blends were prepared by first adding clays to starch in concentrations of 2.5, 5.0, 7.5, and 10% (w/w) relative to starch in glass vials and mixed well. Water was added to the blended sample to maintain a starch to water ratio of 1:3, which was found to be optimum from the preliminary runs. All clay-starch dispersions were sonicated for 30 min in an Aquasonic Model 50HT water bath (VWR Scientific, Mississauga, Ontario, Canada) followed by continuous magnetic stirring for 2 h to ensure well blending of nanoclay and starch dispersion.
Thermal Analysis
Thermal analysis was performed on a TA Q100 differential scanning calorimeter (DSC) (TA Instruments, Newcastle, DE, USA) calibrated with indium under an ultra high purity nitrogen atmosphere (flow rate 50 mL/min) at a 5°C/min heating ramp. An empty aluminum pan served as reference. Sample weights of approximately 10 mg were employed and the samples were encapsulated in aluminium pans and sealed.
Oscillatory Rheology
Rheological measurements of lentil starch and composites were performed on a controlled-stress rheometer (AR 2000Ex, TA Instruments, New Castle, DE, USA) with parallel plate geometry (40 mm in diameter). The width of the gap between two plates was 1000 μm. The sample temperature was internally controlled by a Peltier system (−20 to 200°C with an accuracy of ±0.1°C) attached with a water circulation unit. A platinum resistance thermometer sensor positioned at the center of the plate ensured temperature control and measurement. For each test, neat starch and blend dispersions (approximately 2 mL) were placed at the center of the rheometer plate for 3 min for temperature equilibration before the actual measurements. The sample perimeter was covered with a thin layer of high-temperature-resistant silicone oil to prevent sample dehydration. Following an initial equilibration of samples for 5 min at 40°C, a linear temperature increment from 40 to 95°C (non-isothermal heating) was carried out at 2.5°C/min at a constant frequency of 1 Hz as per the method described by Ahmed et al.Citation[23] for rice starch. The isothermal frequency sweep tests were carried out at different temperatures ranging from 70 to 90°C in the selected frequency range between 0.1 and 10 Hz. The domain of linear viscoelasticity was established by the oscillatory stress sweep tests for each sample. The temperature range was chosen because the range was above the gelatinization temperature of lentil starch (68°C). Rheology Advantage software (TA Instruments, New Castle, DE, USA) was used to acquire data. The average of the duplicate runs was reported as the measured value. The master curves were generated by using the principle of time-temperature superposition and shifted to a common reference temperature (Tref ) of 80°C, which was chosen as the temperature above gelatinization temperature of starch.
Data Analysis
Linear regressions were carried out using Minitab statistical software package (version 15.1.30.0; Minitab Inc., PA, USA). Trends were considered significant while means of compared sets differed at p < 0.05 (Student's t-test).
RESULTS AND DISCUSSION
Thermal Analysis
The thermal transitions of the bionanocomposites have traditionally been elucidated using DSC. The starch is semi-crystalline in nature and undergoes a thermal transition (i.e., gelatinization) in the presence of sufficient water. The peak gelatinization temperature (Tp ) for the neat starch was recorded at 65.7°C. This value was slightly lower than that of our earlier study,Citation[22] which is believed to be attributed by starch-water ratio. Incorporation of clay into starch (2.5, 5, 7.5, and 10% w/w) affected the Tp significantly, and the value increased from 65.7 to about 70°C although thermograms became flat at higher clay concentrations (). An increase in Tp in the blend as function of clay concentration was expected since there was a competition between starch and nanoclay towards water, and furthermore, availability of water was competitive at higher clay concentrations. It is also believed that a significant portion of polymer chains become immobilized in the clay nanocomposites.Citation[21] From X-ray studies, Chiou et al.Citation[16] observed that the Cloisite 30B sample had a d001 spacing of 2.11 nm, which was higher than the 1.87 nm spacing for pure Cloisite 30B, and an intercalation occurred during gelatinization.
Rheology
Effect of clay addition on neat lentil starch at 30°C before thermal treatment is illustrated in . The elastic modulus (G′) for both samples showed consistently higher values (3–4 times) than the viscous modulus (G″) and, therefore, a pre-dominating solid-like property was observed. The complex viscosity (η*) decreased systematically with frequency and the clay-enriched sample showed higher values throughout the frequency range. The phase angle (δ) values decreased in the lower frequency range (0.1 to 1 Hz), and increased thereafter. However, mostly the δ values remained in small domain (9–12°) and behaved like a true visco-elastic fluid. Similar solid-like behavior of clay/starch blend before gelatinization has been reported by Chiou et al.Citation[16] However, authors found that hydrophilic clay (Cloisite Na+) incorporated nanocomposite exhibited higher G′ (almost two orders of magnitude).
Non-Isothermal Starch/Clay Rheology
The evolving complex viscosity (η*) of lentil starch/clay blend during linear heating (2.5°C/min) from 25 to 95°C is presented in . The complex viscosity (η*) development of lentil starch/clay blend dispersion was slow in the beginning, attained a peak value during non-isothermal heating (function of time and temperature) leading to sol–gel conversion, and decreased thereafter. The peak gelatinization temperature was detected at about 70°C, which was higher than that of DSC observation. This difference is attributed by two different measurement techniques and sensitivity of the instruments. However, no distinct gelatinization temperature was observed when clay was incorporated into starch dispersions. With further heating, the η* increased drastically. The maximum value of η* corresponding to temperature T during heating ramp can be considered as the peak complex modulus (T η*max) temperature. The T η*max value marginally decreased from 81.6 to 80.4°C with an increase in clay concentration. Earlier, Chiou et al.Citation[16] also observed similar results for starch/Cloisite 30B blend. This indicated that these nanoclays did not have much of a reinforcement effect at elevated temperatures for short contact time. A better intercalation was found between starch and Cloisite Na+ nanoclay where the leached amylose and smaller amount of amylopectin containing hydroxy groups interact with the hydrophilic Cloisite Na+ nanoclay.Citation[16]
Isothermal Starch/Clay Rheology
In order to broaden the observation's horizon of viscoelastic properties, the method of superposition of time (frequency) and temperature was applied.Citation[24] This is a basic method for data analysis originating from rheological measurements of synthetic polymers. In this technique, log-log plots of the isotherms of the elastic modulus (G′) and complex viscosity (η*) can be superimposed by horizontal shifts log (aT ), along the log (ω) axis, and vertical shifts given by log (bT ) such that:Citation[6 Citation,10 Citation,11 Citation,24 Citation,25]
The linear visco-elastic master curves for the neat starch dispersions are generated by the time-temperature superposition (TTS) principle and shifted to a reference temperature (Tref ) of 80°C with both the horizontal shift actor aT and the vertical shift factor bT . and show the reduced angular frequency, aT ω dependence of elastic modulus (G′), and complex viscosity (η*) for neat specimens for five selected temperatures (70, 75, 80, 85, and 90°C). In some cases, it is necessary to apply vertical shift to the visco-elastic moduli to obtain better superposition. The TTS clearly indicated that G′-ω data was not satisfactory for starch dispersion at 70 and 90°C and did not follow the rule. Calculated values of aT coefficient are not close to unity and, therefore, scaling has significant effect on extending the frequency window. However, the TTS was fitted well for complex viscosity except at 70°C (). One of the possible reasons for non-applicability of TTS at 70°C could be the close proximity of applied temperature to the gelatinization temperature of starch where semi-crystalline starch granules have not completely converted to amorphous region. Hagen and WeissCitation[26] opined that the TTS should be strictly used in amorphous region.
Figure 4 Applicability of TTS for neat starch sample: (a) elastic modulus and (b) complex viscosity.
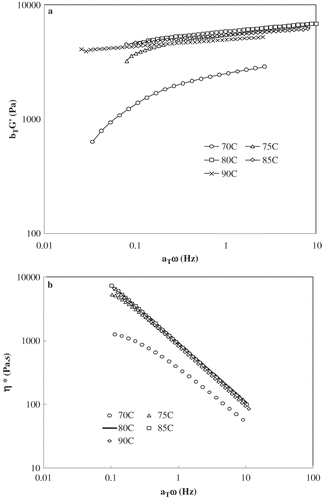
An addition of 2.5–5% nanoclay to starch blend showed marginal improvement in the superposition (η*-ω data) compared to neat starch dispersion ( and ). The η*-ω data for both clay concentrations deviated at 70°C. Further increase in clay concentration showed better superposition and an excellent superposition was observed at 10% incorporation ( and ). Calculated values of aT coefficient 10% clay in the blend are almost close to unity, and therefore, scaling has less effect on the frequency window (). However, G′-ω data failed to follow the TTS rule especially at 70°C as discussed earlier for neat starch dispersion. Samples experiencing a short thermal history (corresponding to high frequencies, 1–10 Hz) produce a satisfactory overlapping of the curves. However, longer thermal exposure (0.1–1 Hz) produces significant departures from time-temperature superposition (especially G″) due to ongoing chemical degradation.Citation[27]
Table 1 Shift factor for η-ω TTS superposition
Figure 8 Applicability of TTS for 10% clay blended starch sample: (a) elastic modulus and (b) complex viscosity.
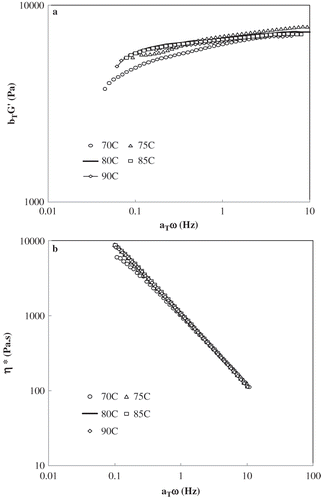
The miscibility of starch and clay into a blend were further analyzed by the Cole-Cole plot of the rheological data representing the relationship between real (η′) and imaginary (η″) parts of complex viscosity.Citation8–12 Citation Citation Citation Citation12, Citation28, Citation29] A smooth, semi-circular shape of the plotted curves indicates good compatibility, i.e., phase homogeneity in the melt, and any deviation from the shape indicates nonhomogenous dispersion or immiscibility. The η′, the real part, and η″, the imaginary part, of complex viscosity are calculated according to the following equations:
illustrates a Cole-Cole plot for starch/clay blend at 80°C. A well smooth semicircular shape of the nanocomposites was observed when clay was loaded to starch dispersion with well distribution between starch and nanoclay. This observation is in contrast to polylactide-nanoclay miscibility as observed earlier by Ahmed et al.,Citation[11] where a threshold concentration was recorded at 6%, and there was no effect afterwards. It is worth mentioning that the Cole-Cole plot is not an absolute technique to determine the miscibility of blends. Other supporting rheological data are required to strengthen the hypothesis.
illustrates the mechanical strength of starch/clay blend. The G′ value increased as function of clay concentration with long contact time. The mechanical strength of nanocomposites is attributed by mechanical reinforcement by clay particle and intercalation at high temperature where the polymer chains swell the gallery spacing and lead to a well-ordered alternating polymer-silicate layered nanostructure.Citation[6] The clay enrichment enhances mechanical property of starch blend, and it could show potential of starch/clay blend for packaging or other biodegradable materials incorporation with suitable plasticizers.
Effect of clay incorporation significantly affects the visco-elasticity of the starch/clay blend after thermal treatment. The slopes of the linear regression of the power-type relationship of ln ω versus ln G′ (Eq. 5) were calculated and presented in . The slope is the indicator of the solid-like characteristics of polymers. A true gel is ascribed by a zero slope of the power law model.Citation[30] The magnitude of the slope for each sample in general decreased with an increase in temperature and exhibiting more solid-like character. There are two clear distinctions of thermally treated samples: one set of samples thermally treated below 80°C and another set at and above 80°C. The second set of samples had completely gelatinized, converted to amorphous state, and there was no change in visco-elasticity (constant slope). Furthermore, clay did not influence in the visco-elasticity of the amorphous state of starch/clay blended gel since the more hydrophobic nanoclays contain a hydrophobic interlayer, which the amylose and amylopectin cannot readily penetrate into.Citation[16] However, more studies on the structure of the blend will provide insight of this observation:
Table 2 Power law parameters for lentil starch/clay blend dispersion during isothermal heating (starch to water ratio 1:3)
CONCLUSIONS
Incorporation of clay into starch dispersions increased the mechanical strength of the composite and showed predominating solid-like behavior (G′ > G″) properties whereas the visco-elasticity of clay-enriched gel remained constant. Two different trends were observed by starch and starch/clay blended dispersions in the studied temperature regime. Overall, the time-temperature superposition (TTS) rule has failed. However, the rule could be valid in the amorphous region (gelatinized sample) as observed from complex viscosity-frequency data at and above 80°C. The form of the oscillatory master curve shows characteristics not before reported, and this needs to be the subject of further investigation. Structural analysis of composite could provide more insight into the blend and clay dispersion.
REFERENCES
- Mali , S. and Grossmann , M.V.E. 2003 . Effects of yam starch films on storability and quality of fresh strawberries . Journal of Agricultural and Food Chemistry , 51 ( 24 ) : 7005 – 7011 .
- Mali , S. , Grossmann , M.V.E. , García , M.A. , Martino , M.N. and Zaritzky , N.E. 2006 . Effects of controlled storage on thermal, mechanical and barrier properties of plasticized films from different starch sources . Journal of Food Engineering , 75 ( 4 ) : 453 – 460 .
- Magalhães , N.F. and Andrade , C.T. 2009 . Thermoplastic corn starch/clay hybrids: Effect of clay type and content on physical properties . Carbohydrate Polymers , 75 : 712 – 718 .
- Mohanty , A.K. , Misra , M. and Hinrichsen , G. 2000 . Biofibres, biodegradable polymers and biocomposites: An overview . Macromolecular Materials and Engineering , 276/277 : 1 – 26 .
- Ray , S.S. and Okamoto , M. 2003 . New polylactide/layered silicate nanocomposites, melt rheology and foam processing . Macromolecular Material Engineering , 288 : 936 – 944 .
- Ray , S.S. , Maiti , P. , Okamoto , M. , Yamada , K. and Ueda , K. 2002 . New polylactide/layered silicate nanocomposites. 1. Preparation, characterization and properties . Macromolecules , 35 : 3104 – 3110 .
- Angellier , H. , Molina-Boisseau , S. and Dufresne , A. 2005 . Mechanical properties of waxy maize starch nanocrystal reinforced natural rubber . Macromolecules , 38 : 9161 – 9170 .
- Joshi , M. , Butola , B.S. , Simon , G. and Kukaleva , N. 2006 . Rheological and viscoelastic behavior of HDPE/Octamethyl-POSS nanocomposite . Macromolecules , 39 : 1839 – 1849 .
- Ginzburg , V.V. , Weinhold , J.D. , Jog , P.K. and Srivastava , R. 2009 . Thermodynamics of polymer-clay nanocomposites revisited: Compressible self-consistent field theory modeling of melt-Intercalated organoclays . Macromolecules , 42 : 9089 – 9095 .
- Ahmed , J. , Varhney , S.K. and Auras , R. 2010 . Rheological and thermal properties of polylactide/silicate nanocomposites films . Journal of Food Science , 75 : N17 – 24 .
- Ahmed , J. , Varhney , S.K. , Auras , R. and Hwang , S.W. 2010 . Thermal and rheological properties of L-polylactide/polyethylene glycol/silicate nanocomposites films . Journal of Food Science , 75 : N97 – 108 .
- Treece , M.A. and Oberhauser , A. 2007 . Soft glassy dynamics in polypropylene-clay nanocomposites . Macromolecules , 40 : 571 – 582 .
- Ahmed , J. and Varhney , S.K. 2011 . Polylactides—Chemistry, properties and green packaging—A review . International Journal of Food Properties , 14 : 37 – 58 .
- Mackay , M.E. , Tuteja , A. , Duxbury , P.M. , Hawker , C.J. , Van Horn , B. , Guan , Z. , Chen , G. and Krishnan , R.S. 2006 . General strategies for nanoparticle dispersion . Science , 311 : 1740 – 1743 .
- McGlashan , S.A. and Halley , P.J. 2003 . Preparation and characterization of biodegradable starch-based nanocomposite materials . Polymer International , 52 : 1767 – 1773 .
- Chiou , B.S. , Yee , E. , Glenn , G.M. and Orts , W.J. 2005 . Rheology of starch–clay nanocomposites . Carbohydrate Polymers , 59 : 467 – 475 .
- Akcora , P. , Kumar , S.K. , Moll , J. , Lewis , S. , Schadler , L.S. , Li , Y. , Benicewicz , B.C. , Sandy , A. , Narayanan , S. , Ilavsky , J. , Thiyagrajan , P. , Colby , R.H. and Douglas , J. 2010 . Gel-like mechanical enforcement in polymer nanocomposite melts . Macromolecules , 43 : 1003 – 1010 .
- Eslami , H. , Grmelaa , M. and Bousmina , M. 2010 . Linear and nonlinear rheology of polymer-layered silicate nanocomposites . Journal of Rheology , 54 : 539 – 562 .
- Park , H.-M. , Lee , W.-K. , Park , C.-Y. , Cho , W.-J. and Ha , C.-S. 2003 . Environmentally friendly polymer hybrids. Part 1. Mechanical, thermal, and barrier properties of thermoplastic starch/clay nanocomposites . Journal of Materials Science , 38 : 909 – 915 .
- Wang , Y. , Lue , A. and Zhang , L. 2009 . Rheological behavior of waterborne polyurethane/starch aqueous dispersions during cure . Polymer , 50 : 5474 – 5481 .
- Kalambur , S. and Rizvi , S.S.H. 2006 . Rheological behavior of starch–polycaprolactone (PCL) NC melts synthesized by reactive extrusion . Polymer Engineering & Science , 46 : 650 – 658 .
- Ahmed , J. and Auras , R. 2011 . Effect of hydrolysis on rheological properties of lentil starch slurry . Food Science and Technology. , 44 : 976 – 983 .
- Ahmed , J. , Ramaswamy , H.S. , Ayad , A. and Alli , I. 2008 . Thermal and dynamic rheology of insoluble starch from Basmati rice . Food Hydrocolloids , 22 : 278 – 287 .
- Rao , Y.Q. and Pochan , J.M. 2007 . Mechanics of polymer-clay nanaocomposites . Macromolecules , 40 : 290 – 296 .
- Ferry , J.D. 1980 . Viscoelastic Properties of Polymers , New York : John Wiley .
- Hagen , R. and Weiss , R.A. 1995 . Assessment of time-temperature superposition of linear viscoelastic behaviour of strongly interacting polymer blends: N-methylated nylon-2,10 and lightly sulfonated polystyrene ionomers . Polymer , 36 : 4657 – 4664 .
- Palade , L.I. , Lehermeier , H.J. and Dorgan , J.R. 2001 . Melt rheology of high L-content poly(lactic acid) . Macromolecules , 34 : 1384 – 1390 .
- Cho , K. , Lee , B.H. , Hwang , K.M. , Lee , H. and Choe , S. 1998 . Rheological and mechanical properties in polyethylene blends . Polymer Engineering & Science , 38 : 1969 – 1975 .
- Kim , L.H. , Rana , D. , Kwag , H. and Choe , S. 2000 . Melt rheology of ethylene 1-octene copolymer blends synthesized by Ziegler-Natta and metallocene catalysts . Korea Polymer Journal , 8 : 34 – 43 .
- Ross-Murphy , S.B. 1984 . “ Rheological methods ” . In Critical Reports on Applied Chemistry, Vol. 5. Biophysical Methods in Food Research , Edited by: Chan , H.W.S . Vol. 1984 , 138 – 199 . Oxford : Blackwell Scientific .