Abstract
The simulation of heat transfer during thermal treatments of foods requires the knowledge of thermophysical properties of the material. In this study some thermophysical properties of okara, such as density, specific heat, thermal conductivity, thermal diffusivity, heat transfer coefficient, and mass transfer coefficient, were obtained during a drying process. Okara was extruded in the shape of spherical pellets and then dried in a fixed bed dryer from 78% of moisture content (w.b.) until 3%. During the drying process, the water content and the temperature of the samples were periodically measured. The thermal properties were obtained from empirical correlations found in the literature, which use the okara centesimal composition, analyzed in a laboratory, and the relative humidity and velocity of the drying air, measured during the process. The results in this study showed that it is possible to estimate the thermal properties cited using the experimental moisture content history and the centesimal composition of the food.
INTRODUCTION
The thermophysical properties of food play an important role in the project of equipments and processes that involve heat and mass transfer, such as drying and freezing of foods, because they are needed in the calculation of thermal charges, energetic dispends, and equipment dimensions.[Citation1,Citation2] Properties such as density, thermal diffusivity, specific heat, and thermal conductivity of the food material are used to estimate the energetic demand and also to predict the microbiological and biochemical spoilage, representing a tool for food safety and quality control.[Citation3,Citation4]
The methods used to measure the thermophysical properties of foods can de divided in steady and non steady state methods, as can be found in scientific literature.[Citation1,Citation5 Citation Citation Citation–8] The line heat source probe is recommended for measuring thermal conductivity of foods because of its simplicity, fastness, convenience, low cost, and suitability for small sample sizes as related in Sweat.[Citation8] The most recommended method to obtain specific heat is the differential scanning calorimeter as cited in Mohesnin.[Citation9] Thermal properties like thermal conductivity and thermal diffusivity can be measured by several steady state methods, such as guarded hot plate, concentric cylinder, and concentric sphere as related in Woodams and Nowrey,[Citation10] and unsteady state methods, such as line source probe, thermal comparator, and thermistor probe. All of these methods have several advantages and disadvantages, and the sources of error during the measurements for each method were listed in detail in the scientific literature.[Citation11,Citation12]
In Elam et al.,[Citation13] the thermal conductivity of three typical crude oils, composed predominantly of aromatic and paraffinic compounds, were measured using the transient hot-wire method. Also, in Lee and Irvine Jr.,[Citation14] the thermal conductivities of non-Newtonian fluids in a shear field were determined, where a coaxial cylinder apparatus with a rotating outer cylinder was used to establish the velocity field in the test fluid, while Komiya and Maruyama[Citation15] proposed a novel technique for short-time measurement of mass diffusion coefficients in transparent liquids using a counter flow type diffusion cell. The mass diffusion coefficients of water solutions of sodium chloride, sodium chlorate, and L-alanine over a wide range of concentrations were derived from measurement data within the maximum error of ±8.5%. Duangthongsuk and Wongwises[Citation12] related a comparison of the differences between using measured and computed thermophysical properties to describe the heat transfer performance of TiO2–water nanofluids. From the previous studies cited, it was note that different techniques can be used for determining thermophysical properties of different products. Some studies about food thermophysical properties' experimental measurement are for processed meat and poultry products as in Marcotte et al.;[Citation16] rice in Zhou et al.;[Citation17] fruits and roots in Alves-Filho et al.;[Citation18] coconut milk in Tansakul and Chaisawang;[Citation19] ice cream in Andrieu et al.;[Citation20] plain yogurt in Kim and Bhowmik;[Citation21] apple, cherry, grape, orange, and tomato juices in George;[Citation22] non-clarified orange juice in Telis-Romero et al.;[Citation23] and meats, fruits, vegetables, milk, and cereals in Polley et al.[Citation24]
The food thermophysical properties are strongly dependent on the temperature and chemical composition, therefore, it is almost impossible to determine experimentally and tabulate the thermal properties of foods and beverages for all the conditions and compositions possible, as cited in ASHRAE.[Citation25] Another method of obtaining thermal properties of foods, which is based on the centesimal composition, can be used. This technique calculates the thermophysical properties based on the values for each pure component of the food, such as moisture, fat, proteins, and ashes, which are found in Singh and Heldman.[Citation26] There are several works in the literature that used this method, such as for predicting enthalpy and thermal conductivity of frozen meat and fish products as in Van der Sman,[Citation27] some properties for clarified juices cited in Peacock[Citation28] and stone fruit from Phomkong et al.,[Citation29] and mass diffusivity for okara from Lescano and Tobinaga.[Citation30] Also, based on this method, Okos and Choi[Citation31] and Heldman[Citation32] have compiled prediction equations for various food products' thermophysical properties.
Okara is the residue of the soy beverage and tofu production, which is obtained in very large quantities. It consists in a mass with around 75% (w.b.) of moisture content, which contains around 95% of the soy grain solid components; therefore, its nutritional value may be high according to Fikiin and Fikiin.[Citation3] Okara contains, for example, around 22 to 27% (d.b.) of good quality vegetable protein, fibers, and lipids and constitutes a low price product for human consumption in accordance with Chan and Ma,[Citation34] Travaglini et al.,[Citation35] and Wang and Calvins.[Citation36]
Using okara as a food component is a method of taking profit of its nutritional value and at the same time represents a way of avoiding the environment contamination because of its high biological value. In order to allow the use of okara in other food products, it is indispensable to dehydrate this product to avoid its fast degradation. However, an extensive literature search revealed that very limited data are available for okara. Regarding okara drying, for example, some works have been published, such as the studies of Taruna and Jindal,[Citation37] Tatsumi et al.,[Citation38] Wachiraphansakul and Devahastin,[Citation39] and Perussello et al.[Citation40] In fact, the goal of this work is to contribute to obtain thermophysical properties for okara, facilitating the design of processing methods, which make possible its use as a food component.
MATERIALS AND METHODS
Okara was previously extruded in spherical pellets of 5 mm diameter, in an extruder brand WEG (model 22STI, WEG, Jaragua do Sul, SC, Brazil), in order to facilitate the heat and mass transfer. The okara pellets were dried in the fixed bed dryer from 78% (w.b.) until the desirable moisture content of about 3%. During the drying processes, carried out with three different drying air temperatures (130, 150, and 170°C), the moisture content and the temperature of the pellets were periodically measured in intervals of 30 s. The analysis of moisture content was carried out by the gravimetric method, which consists of submitting the sample to a convection drying in a stove at 105°C for around 8 h. The temperature profiles were obtained through the measurement of the pellets' temperature with T-type thermocouples. An HP Agilent data acquisition system (model 33250A, Agilent Technologies, SiliconValley, CA, USA) and a computer were also used for data storage. The relative humidity was obtained through the measurements of the air dry bulb temperature (with a thermometer) and wet bulb temperature (with a thermometer with its extremity covered by a humid gauze) and the use of a psychometric chart. The air velocity was measured with a Testo hot wire anemometer (model 445, Testo do Brazil, Campinas, SP, Brazil).
Construction of the Fixed Bed Dryer
The drying equipment used in this study, which is shown in , is a fixed bed dryer. In order to simulate a fixed bed dryer, an adaptation in a Lab Plant spray-dryer (model SD-05, Lab Plant, North Yorkshire, UK) was made. A cylinder with a diameter of 12 cm that fits in the air exit was built. At the end of the tube, a structure that consists of a 5-cm-height cylinder was encased. This structure has its upper part opened to permit the air flow and its lower part is a drilled net, which accommodates the okara pellets that are being dried. The components of the fixed bed dryer are indicated in and can be summarized as follows: (1) adjustment, control, and indication of the air temperature; the temperature control is performed by a proportional integral and derivative (PID) controller; (2) operational section of the dryer, which comprises a blower, a compressor, a heater, and a peristaltic pump; (3) air supply tube; (4) extension of the air supply tube, built for this work; (5) fixed bed dryer, where the product is placed during the drying process, also built for this work. Another method for the design and redesign of pneumatic dryers, applied for potato particles that suffered from ignition of resident particles, was proposed in de Best et al.[Citation41]
Centesimal Composition Analysis of Okara
The centesimal composition of okara () was analyzed in a laboratory according to standard techniques, which can be found in Matissek et al.[Citation42] The analysis of moisture content used samples of 3.0 ± 0.5 g, which were conditioned in porcelain capsules and heated in a stove regulated at 105°C. The protein analysis was carried out by the Kjeldahl digestion method, the fat evaluation was made by the continuum extraction in Soxhlet type equipment, and the ashes analysis or fixed mineral residue was carried out by the mass loss through the incineration of the sample in a mufla stove at 550°C. The total carbohydrates were calculated by difference, by subtracting from 100 the values found for moisture content, proteins, lipids, and ashes. The tests were performed in triplicate.
Table 1 Centesimal composition of okara
Table 2 Drying air relative humidity and velocity in function of its temperature
Porosity is a key property that influences the thermophysical properties of food products. Its effect on specific heat is negligible, but its effect on density and thermal conductivity can be absolutely meaningful according to Mannaperuma and Singh.[Citation43] Thus, if porosity is not considered inside the product, the heat transfer numerical analyses and, in consequence, the mass transfer during drying can be erroneously obtained. Therefore, the okara's porosity in this study was obtained in the laboratory, using the picnometry technique. The density of two okara samples was measured using a pycnometer of 50 mL. The first sample is extruded okara, the product used in all the experiments. The second sample is okara homogenized in such a way that the air from the extrusion was removed. To remove the air, the okara mass was pressed between two cylinders, in an equipment for pasta formatting. The results of those measurements are presented in , and the final result for porosity was calculated by EquationEq. (1):
During the drying process, the moisture content and water activity (aw ) in the okara pellets are reduced to certain levels. The moisture content and water activity of these samples are key quality factors of okara. Too high moisture content and water activity may lead to microorganism growth and poor quality characteristics, while too low moisture content and water activity may lead to excessive energy consumption and product quality damages. The water activity with different known moisture contents was measured with an Aqualab hygrometer (model CX-2, Decagon Devices, Pullman, WA, USA).
The thermal properties were obtained experimentally from the variable composition of the okara during the drying process. As each process condition tested in this work (time and temperature) influences the water loss of the okara during drying, the thermal properties were evaluated at all air temperatures tested. Density and thermal conductivity were obtained from the centesimal composition considering the porosity (ϵ). Density is multiplied by a factor (1 − ϵ). In order to account for the porosity effect, this adjustment on density corrects also the thermal conductivity, which is calculated using density.
In order to obtain density, specific heat, and thermal conductivity, the properties of each okara pure component (water, carbohydrates, fats, proteins, ashes) obtained by George[Citation22] were used, in proportion of its respective mass fraction, as described in EquationEqs. (2) Equation Equation–Equation(5):
The equilibrium moisture content values for each drying condition used were also obtained. The equilibrium moisture content, Xe , was obtained by using a correlation for okara between Xe and the relative humidity of the drying air (RH), according to Lescano et al.,[Citation48] as EquationEq. (11) shows:
EXPERIMENTAL RESULTS
In wet and dry basis, respectively, the analysis of the centesimal composition of okara provided the results presented in . The calculation of the dry basis composition from the wet basis composition was done by EquationEq. (12). The high nutritive value of okara becomes evident when one observes the values for dry basis composition. For example, almost 40% of the solid fraction is composed by proteins:
Obtaining the Moisture Content Profile for Okara
The conditions of the air supplied by the spray-dryer are variable with temperature. The results, obtained for drying air relative humidity and velocity, are described in . One can observe that the air relative humidity decreased with the increase in temperature. The air velocity, however, increased with temperature. It must be noted that due to the spray-dryer limitations, the air velocities obtained experimentally are extremely low. For this reason, it was not possible to analyze the air velocity influence on the drying time. However, it is known that if it was possible to use velocities of the order of 1 m/s, as is usual in food drying processes, the drying time would decrease considerably. According to Desrosier,[Citation49] the use of drying air at constant temperatures with a speed of 1.15 m/s reduces drying time in half in comparison with the still air. When using 2.3 m/s, the drying rate triples. Axtell and Bush[Citation50] suggest air velocities between 0.5 to 1.5 m/s to dry food products.
shows the moisture content versus time for each drying temperature used. The increase in air temperature reduces drying times, which were 9, 10, and 15 min for 170, 150, and 130°C, respectively. In up to 4 min of drying, the behavior of the curves is similar, but after this period the effect of temperature is more pronounced on the drying time reduction, especially to the process using 170°C. That happened because the air relative humidity is lower to this process (0.77% versus 1.17%) and the air velocity is higher (0.073 m/s versus 0.051 m/s). Such variables, i.e., relative humidity and velocity, are a function of the air temperature and could not be set to a different value due to the equipment limitations. Although they were only measured, air temperature was controlled during the whole drying process by the PID controller of the spray-dryer. In spite of the differences on drying times, all the curves presented the same tendency, i.e., the moisture content decreases faster until 5 min of process and after this the drying speed decreases. The moisture transfer velocity decreases because, as the water content to be removed reduces, the reminiscent water in the product has to migrate to its surface and also because the water is stronger attached to the other food components, being more difficult to be removed.
Knowing that the water activity (aw ) of a food must be decreased until approximately 0.3 in order to avoid the microbiological and enzymatic degradation reactions, an equation that correlates aw and moisture content for okara was obtained. Okara samples with different values of moisture content were submitted to the measurement of aw in a moisture analyzer (Aqualab) and a polynomial fit was done using the software MATLAB©. The results obtained for the water activity are illustrated in and are expressed by:
Obtaining the Thermophysical Properties for Okara
The values of the okara thermophysical properties are presented in Figs. in function of the process time and moisture content. One can observe that the okara density increases with time, while the specific heat, thermal conductivity, and thermal diffusivity decrease with time. This means that as the moisture is removed from the product, the heat transfer turns more difficult; hence, the energetic demand for the process increases. Observing the curves of thermal properties versus time, it is evident that the okara properties for 170 and 150°C present nearer values than the comparison between 150 and 130°C, although the temperature interval is the same, i.e., 20°C. This result was obtained because the air speed difference between the temperatures of 150 and 170°C is almost three times greater than the difference between 130 and 150° (0.007 m/s versus 0.022 m/s), although the difference between the relative humidity is roughly equal (-0.40% versus -0.39%). This fact led to a major similarity between the moisture content of the products being dried at 130 and 150°C, explaining the results discussed, since the thermal properties are strongly dependent on food composition and moisture content. The values found for density ( and ) are between 825 and 1125 kg/m3, increasing along the drying process and with the decrease on moisture content. These results were expected, since the solid fraction of okara presents a larger density than that of the water.
Figure 6 Okara thermal conductivity during drying versus (a) time (min) and (b) moisture content (kg/kg).
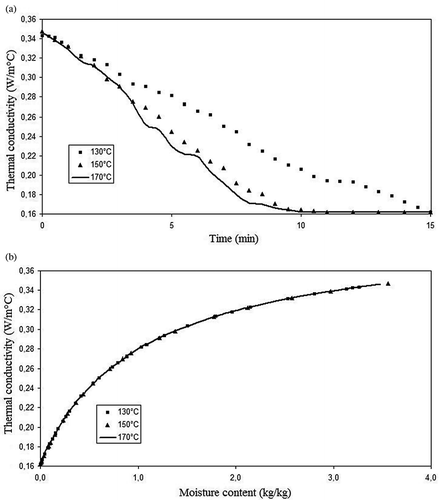
Figure 7 Okara thermal diffusivity during drying versus (a) time (min) and (b) moisture content (kg/kg).
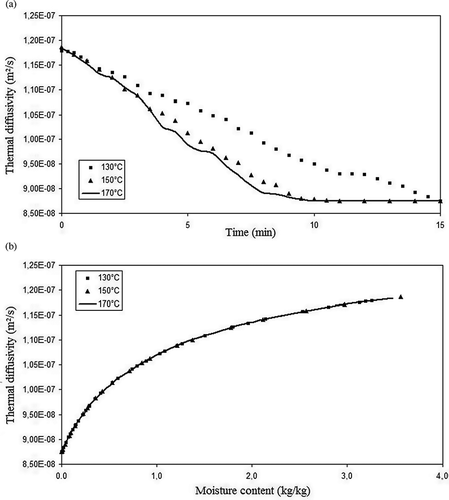
Specific heat places between 1500 and 3500 J/kg·K, decreasing along time and with the decrease on moisture content. In fact, according to Sweat,[Citation8] there is a strong linear correlation between specific heat and water content, especially over the highest range of water contents. Over the lower range of water contents, it depends more on the other constituents of the food. The very same result is observed in , in which specific heat varies almost linearly with moisture content until the value of 2 kg/kg, after this the correlation becomes polynomial.
The values found for thermal conductivity are between 0.20 and 0.45 W/m·K. In and the thermal conductivity decreased with process time and with the decrease on moisture content, which is coherent with Sweat.[Citation8] Besides, thermal conductivity of porous foods depends strongly on the porosity, which was accounted in this work. Similar results for 25 types of fresh fruits and vegetables, considering also porous materials, such as apples or beans, were reported by Sweat,[Citation8] with values varying from 0.1 to 0.8 W/m·K.
The thermal diffusivity ( and ), by its turn, represents the speed of the product's temperature response in function of the drying air temperature, and the values found its place between 0.85 × 10−7 m2/s and 1.20 × 10−7 m2/s. Thermal diffusivity varied similarly as thermal conductivity, like it was reported by Sweat[Citation8] in regards to food products in general.
In summary, the values obtained for these thermophysical properties of okara are coherent with the values found in ASHRAE[Citation25] for food products with centesimal composition alike.
The polynomials that correspond to the properties above in function of moisture content for the range of temperatures used in this work are presented in . These polynomials were fit using the software MATLAB©.
The results for the air velocity, Re, and Nu for all drying temperatures tested experimentally are found in . The values of Re and Nu increase with the increase in drying temperature, and indicate that the inertial forces are about 10 times higher than the viscosity forces, i.e., the flow is laminar. Arrieche[Citation51] studied the phenomenon of fluid-particle interactions for spheres in a drying process by forced convection. The air has a velocity called v ∞, which is zero at the stagnation point and increases as the fluid surrounds the body, along the zenith angle, θ, forming a boundary layer. The higher the Re values, the smaller the angle theta in the separation of the boundary layer, influencing the convective drying of materials. This is the major reason why industry uses artificial processes with forced convection to decrease drying time like Vilela and Artur.[Citation52] This explains also why the process at 170°C has a higher rate of moisture removal from the okara pellets.
Table 3 Okara thermophysical properties
Table 4 Air velocity, Re, and Nu for okara during drying at different temperatures
Table 5 Okara drying parameters
The results for Xe , h, hm , and Dap for all the drying temperatures tested are presented in . The equilibrium moisture decreases with the increase in temperature, i.e., it is possible to obtain drier products using higher temperatures. The influence of temperature on the heat transfer coefficients and in the mass transfer coefficients is remarkable, as obtained by several works regarding food drying. The mass transfer coefficient in the final stages of drying was lower for the tests accomplished at 130°C and reflects a great influence of the internal resistance to the mass transfer in operating in a lower temperature. The mass diffusivity of the water inside the okara pellets is also directly proportional to the air drying temperature, favoring drying at higher temperatures. Among the values used, the mass diffusivity is of the same order of magnitude and is placed inside the interval expected for okara or foods with composition similar to it, which varies from 10−9 to 10−11 m2/s, according to Lescano and Tobinaga.[Citation30] Considering that Dap represents the capacity of the water to migrate from inside the product to its outside in function of the temperature and velocity of the drying air, if the equipment used made possible the use of the same air velocity for the different temperatures tested, it would be expected that the difference on the diffusivity as a function of temperature would be larger.
CONCLUSIONS
In the present work, the thermophysical properties of okara were obtained from equations that utilize its centesimal composition, the moisture content profiles, and the drying air properties, obtained experimentally during the drying processes. The results showed that as the product is being dried, the energetic dispends enlarge, which is represented by a decrease of the properties specific heat, thermal conductivity, and thermal diffusivity along the drying process. The equations used to calculate the thermophysical properties of okara presented satisfactory results, providing values inside the range expected for products with centesimal composition similar to okara, such as skim, found in ASHRAE.[Citation25] The values found for density place between 825 and 1125 kg/m3, specific heat varied from 1500 to 3500 J/kg·K, thermal conductivity placed between 0.20 and 0.45 W/m·K, and thermal diffusivity varied from 0.85 × 10−7 to 1.20 × 10−7 m2/s for the range of drying air temperatures used in this work. Regarding the values found for the heat transfer coefficient (h), mass transfer coefficient (hm ), and apparent mass diffusivity (Dap ), which depend strongly on the drying air conditions used (such as temperature and velocity), the results obtained for okara also place inside the expected range. The values obtained for h varied from 8.93 to 11.72 W/m2·K and the values for hm placed between 1.26 × 10−5 and 1.63 × 10−5 kg/m2s. Similarly to the results obtained by Lescano and Tobinaga[Citation30] for okara, Dap varied from 4.1 × 10−9 to 7.4 × 10−9 m2/s.
NOMENCLATURE
aw | = |
Water activity |
cp | = |
Specific heat (J/kg·K) |
cpa | = |
Air specific heat (J/kg·K) |
d | = |
Okara pellets diameter (m) |
Dm | = |
Water mass diffusivity in the air (m2/s) |
Dap | = |
Water mass apparent diffusivity in the okara sample (m2/s) |
h | = |
Convection heat transfer coefficient (W/m2·K) |
hm | = |
Mass transfer coefficient (kg/m2s) |
k | = |
Thermal conductivity (W/m·K) |
kair | = |
Air thermal conductivity (W/m·K) |
m,K, q, w | = |
Parameters involved in EquationEq. (13) |
Nu | = |
Nusselt number |
Re | = |
Reynolds number |
RH | = |
Air relative humidity (%) |
v | = |
Air flow velocity (m/s) |
X | = |
Moisture content w.b. (%) |
Xe | = |
Okara equilibrium moisture content dry basis (kg/kg) |
xn | = |
Percentage of the solid component in the okara wet basis composition (%) |
xj | = |
Each pure component mass fraction in the okara composition |
xs | = |
Percentage of the solid fraction in the okara wet basis composition (%) |
xvj | = |
Each pure component volumetric fraction in the okara composition |
α | = |
Thermal diffusivity (m2/s) |
α air | = |
Air thermal diffusivity (m2/s) |
ϵ | = |
Porosity (%) |
μ | = |
Air viscosity (kg/m·s) |
ρ | = |
Okara density (kg/m3) |
ρ a | = |
Density of the okara sample without air (g/cm3) |
| = |
Density of the extruded okara sample (g/cm3) |
| = |
Air density (kg/m3) |
ACKNOWLEDGMENTS
The authors would like to thank CAPES (Brazil) for the scholarship granted to Camila Augusto Perussello, as well as CNPq (Brazil) (Processes: 568221/2008-7, 475689/2010-0, and 302786/2008-2/PQ) for the financial support of this work.
REFERENCES
- Hamdami , N. , Monteau , J.Y. and Le Bail , A. 2004 . Transport properties of a high porosity model food at above and sub-freezing temperatures. Part 2: Evaluation of the effective moisture diffusivity from drying data . Journal of Food Engineering , 62 : 385 – 392 .
- Njie , D.N. , Rumsey , T.R. and Singh , R.P. 1998 . Thermal properties of cassava, yam and plantain . Journal of Food Engineering , 37 : 63 – 76 .
- Fikiin , K.A. and Fikiin , A.G. 1999 . Predictive equations for thermophysical properties and enthalpy during cooling and freezing of food materials . Journal of Food Engineering , 40 : 1 – 6 .
- Lewis , M. 1983 . Propiedades Físicas de los Alimentos y de los Sistemas de Procesado , Zaragoza : Acribia .
- Ali , S.D. , Ramaswamy , H.S. and Awuah , G.B. 2002 . Thermo-physical properties of selected vegetables as influenced by temperature and moisture content . Journal of Food Process Engineering , 25 : 417 – 433 .
- Mohamed , I.O. 2003 . Simultaneous estimation of thermal conductivity and volumetric heat capacity for solid foods using sequential parameter estimation technique . Food Research International , 36 : 617 – 623 .
- Sablani , S.S. and Rahman , S. 2010 . Using neural networks to predict thermal conductivity of food as a function of moisture content, temperature and apparent porosity . Food Research International , 43 : 709 – 715 .
- Sweat , V.E. 1994 . Engineering Properties of Foods , New York : Marcel Dekker .
- Mohesnin , N.M. 1980 . Thermal Properties of Food and Agricultural Materials , New York : Gordon and Breach Science Publishers .
- Woodams , E.E. and Nowrey , J.E. 1968 . Literature values of thermal conductivities of foods . Journal of Food Technology , 22 : 494 – 502 .
- Qashou , M.S. 1973 . Compilation of thermal conductivity of foods , Auburn , NY : Auburn University . Unpublished M.S. Thesis
- Duangthongsuk , W. and Wongwises , S. 2010 . Comparison of the effects of measured and computed thermophysical properties of nanofluids on heat transfer performance . Experimental Thermal and Fluid Science , 34 : 616 – 624 .
- Elam , S.K. , Tokura , I. , Saito , K. and Altenkirch , R.A. 1989 . Thermal conductivity of crude oils . Experimental Thermal and Fluid Science , 2 : 1 – 6 .
- Lee , D.-L. and Irvine , T.F. Jr. 1997 . Shear rate dependent thermal conductivity measurements of non-Newtonian fluids . Experimental Thermal and Fluid Science , 15 : 16 – 24 .
- Komiya , A. and Maruyama , S. 2006 . Precise and short-time measurement method of mass diffusion coefficients . Experimental Thermal and Fluid Science , 30 : 535 – 543 .
- Marcotte , M. , Taherian , A.R. and Karimi , Y. 2008 . Thermophysical properties of processed meat and poultry products . Journal of Food Engineering , 88 : 315 – 322 .
- Zhou , Z. , Robards , K. , Helliwell , S. and Blanchard , C. 2010 . Effect of storage temperature on rice thermal properties . Food Research International , 43 : 709 – 715 .
- Alves-Filho , O. , Strommen , I. and Tgorbergsen , E. 1997 . A simulation model for heat pump dryer plants for fruits and roots . Drying Technology , 15 : 1369 – 1398 .
- Tansakul , A. and Chaisawang , P. 2006 . Thermophysical properties of coconut milk . Journal of Food Engineering , 73 : 276 – 280 .
- Andrieu , J. , Besson , A. , Cogné , C. , Laurent , P. and Nocquet , J. 2003 . Experimental data and modeling of thermal properties of ice creams . Journal of Food Engineering , 58 : 331 – 341 .
- Kim , S.S. and Bhowmik , S.R. 1997 . Thermophysical properties of plain yogurt as functions of moisture content . Journal of Food Engineering , 32 : 109 – 124 .
- George , R.M. 1990 . A literature survey of thermal diffusivity of food products . Campden Food & Drink Research Association, Technical Bulletin , 73 : 1 – 23 .
- Telis-Romero , J. , Telis , V.R.N. , Gabas , A.L. and Yamashita , F. 1989 . Thermophysical properties of Brazilian orange juice as affected by temperature and water content . Journal of Food Engineering , 38 : 27 – 40 .
- Polley , S.L. , Snyder , O.P. and Kotnour , P. 1980 . A compilation of thermal properties of foods . Journal of Food Technology , 34 : 76 – 94 .
- 2002 . “ Thermal properties of food ” . In Refrigeration Handbook , Atlanta , GA : ASHRAE . ASHRAE (American Society of Heating, Refrigerating and Air-Conditioning Engineers
- Singh , P.R. and Heldman , N.D.R. 1993 . Introduction to Food Engineering , 2nd , New York : Academic Press .
- Van der Sman , R.G.M. 2008 . Prediction of enthalpy and thermal conductivity of frozen meat and fish products from composition data . Journal of Food Engineering , 84 : 400 – 412 .
- Peacock , S. 1995 . Predicting physical properties of factory juices and syrups . International Sugar Journal , 97 : 571 – 577 .
- Phomkong , W. , Srzednicki , G. and Driscoll , R.H. 2006 . Thermophysical properties of stone fruit . Drying Technology , 24 : 195 – 200 .
- Lescano , C.A.A. and Tobinaga , S. 2004 . Modelo codificado e real para a difusividade efetiva da secagem do resíduo do extrato hidrossolúvel de soja . Revista Brasileira de Produtos Agroindustriais , 6 : 17 – 25 . in Portuguese
- Okos , M.R. and Choi , Y. 1986 . “ Thermal properties of liquid foods ” . In Physical and Chemical Properties of Foods , Edited by: Okos , M.R. 35 – 77 . Michigan : American Society of Agricultural Engineers: St. Joseph .
- Heldman , D.R. 2001 . Prediction Models for Thermophysical Properties of Foods , New York : Marcel Dekker .
- Smith , A.K. and Circle , S.J. 1978 . Soybeans: Chemistry and Technology , Westport , CT : AVI .
- Chan , W.M. and Ma , C.Y. 1999 . Acid modification of proteins from soymilk residue (okara) . Food Research International , 32 : 119 – 127 .
- Travaglini , D.A. , Aguirre , J.M. , Travaglini , M.M.E. , Silveira , E.T.F. , Delazari , I. and Figueiredo , I.B. 1980 . Fabrication process of powder soy extract . Collectanea of the Food Technology Institute , 11 : 139 – 152 .
- Wang , H.L. and Calvins , J.F. 1989 . Yield and amino acid composition of fractions obtained during tofu production . Cereal Chemistry , 66 : 359 – 361 .
- Taruna , I. and Jindal , V.K. 2002 . Drying of soy pulp (okara) in a bed of inert particles . Drying Technology , 20 : 1035 – 1051 .
- Tatsumi , E. , Li , F.D. , Li , L.T. and Sun , J.F. 2005 . Electrohydrodynamic (EHD) drying characteristic of okara cake . Drying Technology , 23 : 565 – 580 .
- Wachiraphansakul , S. and Devahastin , S. 2005 . Drying kinetics and quality of soy residue (okara) dried in a jet spouted-bed dryer . Drying Technology , 23 : 1229 – 1242 .
- Perussello , C. A. , Amarante , A. C. C. and Mariani , V. C. 2009 . Convective drying kinetics and darkening of okara . Drying Technology , 27 : 1132 – 1141 .
- de Best , C.J.J.M. , van der Geld , C.W.M. , Roccia , A.M. and van Kemenade , H.P. 2007 . A method for the redesign of pneumatic dryers . Experimental Thermal and Fluid Science , 31 : 661 – 672 .
- Matissek , R. , Schnepel , F.M. and Steiner , G. 1998 . Análisis de los Alimentos: Fundamentos, Métodos, Aplicaciones , Zaragoza : Acribia .
- Mannaperuma , J.D. and Singh , R.P. 1998 . Prediction of freezing and thawing times of foods using a numerical method based on enthalpy . Journal of Food Science , 53 : 626 – 630 .
- Incropera , F.P. and Dewitt , D.P. 1990 . Fundamentals of Heat and Mass Transfer , 3rd , New York : J. Wiley & Sons .
- Holman , J.P. 1986 . Heat Transfer , New York : McGraw-Hill .
- Storn , R. and Price , K. Differential evolution: A simple and efficient adaptive scheme for global optimization over continuous spaces . Technical Report TR-95-012 . 1995 . International Computer Science Institute: Berkeley, CA
- Storn , R. 1997 . Differential evolution—A simple and efficient heuristic for global optimization over continuous spaces . Journal of Global Optimization , 11 ( 1 ) : 341 – 359 .
- Lescano , C.A.A. , Fraile Neto , V. and Rocha , S.C.S. “ Higroscopicidade do resíduo de leite de soja (okara) seco em leito de jorro com inertes ” . In XXXIII Encontro Brasileiro de Sistemas Particulados 17 – 19 . Aracaju, Brazil, 16–19 October, 2007in Portuguese
- Desrosier , N.W. 1963 . The Technology of Food Preservation , : 405 AVI Pub. Co.
- Axtell , B. and Bush , A. 1991 . Try Drying It. Case Studies in the Dissemination of Jray Drying Technology , London , , Brazil : Intermidate Technology Publications .
- Arrieche , L.S. 2008 . Modelagem matemática do processo de secagem, por convecção forçada, de sistemas simuladores de alimentos esféricos e deformáveis , 120 São Carlos , , Brazil : Universidade Federal de São Carlos . Programa de Pós-Graduação em Engenharia Química, Dissertação de Mestrado
- Vilela , C.A.A. and Artur , P.O. 2008 . Drying of Curcuma longa L. in different shapes . Ciência eTecnologia de Alimenos , 28 ( 2 ) : 387 – 394 .