Abstract
The solubility of proteins from quail egg white subjected to different agitation times, pH, and NaCl at 25°C was evaluated in this study. The greatest solubility achieved was obtained in the 0.05 mol/L of NaCl, pH 10.0, and under agitation for 1 h. The lowest solubility was found in the NaCl 1.0 mol/L, pH 3.0, and agitated for 2 h. Under the test conditions, aqueous solutions of NaCl 1.0 mol/L, pH 3.0, and agitated for 2 h may provide the greatest extraction index of proteins from quail egg white because these conditions promoted the protein precipitation in a large extension.
INTRODUCTION
The quail egg is a high quality food with important nutritional contribution at low cost. Small size, rapid growth, and early onset of egg production enable quail enterprises to be established with a low capital outlay, and to start generating income quite quickly, therefore making commercial quail farming more popular.[Citation1,Citation2] Egg white is an essential ingredient used in the food industry due to its excellent technological properties.[Citation3] Of the 23.7 g of proteins found in the quail egg, 6.3 g are proteins of high biological value, considered to be excellent in the composition of the human diet, and contain practically all the nutrients essential to human life.[Citation3,Citation4]
Among the functional properties of proteins, solubility is decisive in the selection of ingredients to be used in beverages, liquid foods, and emulsions under different processing conditions since this property influences other protein functional characteristics, such as emulsification, gelling, and the formation of foam, among others. Additionally, solubility data are used for the determination of optimal protein extraction and purification conditions.[Citation5–Citation8] The increased commercial value of the purified proteins allow their use, for example, in the formulation of nutritional supplements for athletes, infants, and patients who require enteral or parenteral feeding, or those with low immunity resistance.
The ways in which proteins interact with a solvent is a function of the physical and chemical properties of the protein at a given condition. A vast array of hydrophobic (protein-protein) and hydrophilic (protein-solvent) surface reactions are involved in protein solubility.[Citation7,Citation9] Various factors influence protein solubility, such as amino acid composition, protein structure (native or denatured), pH, salt concentration, and temperature among others.[Citation6,Citation10] Some physical parameters can also affect protein solubility, such as mechanical agitation, which can cause denaturation of the proteins.[Citation10]
The pH of the solution affects the distribution of liquid charges on the surface of the protein and consequently its solubility. In general, proteins are more soluble in either acid or alkaline solutions due to excess of equal charges, which produce repulsion among molecules, increase water-protein interaction and, therefore, solubility. Minimal solubility is encountered at the isoelectric point (pI), where in an aqueous system the protein presents a charge equal to zero. However, precipitation does not necessarily occur at the pI, making protein solubility dependant on other disturbances in the solvent properties, such as the addition of salt.[Citation5–Citation7,Citation10–Citation13]
Salts can also affect the electrostatic interactions between macromolecules and increase protein solubility at concentrations of 0.1 to 1.0 mol/L. However, this behavior depends on the concentration and type of salt present in the medium, protein structure, pH, and temperature.[Citation5] Salt ions interact with opposing charge groups on the protein to form a double layer of ionic groups, which diminish the electrostatic interaction between the molecules causing more protein solvation, thus increasing solubility. Solubility decreases when there is greater competition between proteins and salt ions for water.[Citation5,Citation10]
Additionally, solubility of proteins from biological systems, such as egg white, egg yolk, and whey bovine blood plasma, is also influenced by other components present in the environment (lipids, sugars, salts, etc.). This result was observed by Biasutti et al.[Citation14] in previous studies of the solubility of whole bovine plasma proteins.
Studies on solubility of proteins from chicken egg white,[Citation9,Citation13,Citation15] chicken egg yolk,[Citation8,Citation16] and quail egg white[Citation17] have described the influence of the type of salt, pH, temperature, and salt concentration on the solubility phenomenon. Due to little information referring to the solubility of quail egg white in the literature, it was the present work that provides solubility data of quail egg white proteins under the influence of variations in pH, NaCl concentration, and agitation time at 25°C.
MATERIAL AND METHODS
Material
The eggs used in the experiment were obtained from the Japanese quail (Coturnix coturnix japonica) breeding site in the Aviculture Sector of the Animal Science Department at the Universidade Federal de Viçosa, MG, Brazil. Egg white was separated manually, frozen, and lyophilized (Edwards L5KR, Tonawanda, NY, USA). Deionized water (Milli-Q device, Millipore Inc., Billerica, MA, USA) and analytical grade reagents were used in all experiments. For construction of the analytical curve, an ovalbumin standard of 98% purity was used (Sigma Chemicals, St. Louis, MO, USA).
Protein Quantification
Proteins were quantified by the Biuret reaction[Citation18] using the analytical curve constructed with ovabulmin (Sigma Chemicals, St. Louis, MO, USA) at the aqueous concentrations of 1.0 to 10.0 mg/mL. Biuret reaction is the addition of 4 mL of Biuret reagent and 1 mL of protein sample, followed by incubation at 37°C for 20 min. Absorbance was read with a spectrophotometer at 540 nm (Cary 50, Varian, Mulgrave, Australia), and the quantity of proteins (mg) in each sample was determined with the referred analytical curve.
Solubility Determination
Solubility data were obtained according to the methodology proposed by Kakalis and Regenstein[Citation9] with some modifications. To a buffer solution of 20 mL, 120 mg of egg white sample was added (6 mg protein/mL) at a predefined pH of 3.0, 4.6, 6.2, 8.0, and 10.0, a fixed NaCl concentration of 0, 0.05, 0.3, 0.5, 0.8, and 1.0 mol/L, and agitation time of 0.5, 1.0, 1.5, and 2.0 h. The buffers used were glycine–HCl (pH 3.0), citrate–citric acid (pH 4.6 and 6.2), phosphate (pH 8.0), and carbonate-bicarbonate (pH 10.0), in accordance with Mohan.[Citation19] The mixtures were agitated in a device that simulated an agitated tank under rotation of 14 rpm, at 25°C.[Citation13,Citation17] Shortly after homogenization, the samples were centrifuged at 20000× g for 20 min at 4°C (model J2-MC, Centrifuge Beckman, Brea, CA, USA). After centrifuging, a 1-mL sample was removed from the supernatant for protein analysis. Analysis of the soluble protein in the supernatant was determined according to the Biuret reaction[Citation18] with absorbance measurements at 540 nm (spectrophotometer, Cary 50, Varian, Mulgrave, Australia). Protein solubility (P.S) (g/100 g), was calculated according to the formula proposed by Morr et al.,[Citation20] in accordance with Eq. (1):
where P.S (%) is the percentage of soluble protein in the sample, A is the protein concentration in the supernatant (mg/mL), W is the sample mass (mg), and S is the total protein concentration in the sample (%).
Statistical Analysis
Experiments were setup in a factorial scheme of completely randomized design with five different pH values, six salt concentrations, and four agitation times, totaling 60 treatments with two repetitions. Each experimental unit was composed of 120 mg of lyophilized egg white. The experimental data were analyzed using the PROC GLM procedure (SAS V.9.0, SAS Institute, Cary, NC, USA).[Citation21] Repeatability of the polynomial equation model was obtained using the coefficient of determination R2, the result of the variance analysis (ANOVA), and significance level from the Fisher test (F, P < 0.05). The significance level of the regression coefficients was obtained by the student’s t-test (p < 0.05).
RESULTS AND DISCUSSION
The percentage of proteins in the lyophilized quail egg white used in the experiments was 98.5% of the total proteins. Solubility data for quail egg white, as a function of agitation time, pH, and NaCl concentration is presented in , and varied (p < 0.05) in function of these three factors. Triple interaction (p < 0.05) was observed, indicating dependence between agitation time, NaCl concentration, and pH values for each of the protein solubility samples studied.
TABLE 1 Protein solubility (g/100 g) of the quail egg white as a function of agitation time, pH, and salt concentration
At the pH levels of 3.0, 4.6, and 8.0, protein solubility diminished with the increase in agitation time. This phenomenon may have been caused by alterations in the native structure of the protein (secondary, tertiary, or quaternary) with the increase in agitation time of the medium. Breakdown of the protein structure can liberate hydrophobic amino acid residues generally found inside the protein molecules. This change in the native structure of the protein can occur during the homogenization step in the food processing process.[Citation22,Citation23] The exposure of these hydrophobic groups increases the protein-protein interactions, diminishing solubility.
Maximum solubility values were obtained at pH 6.2 with an agitation time of 1.5 h, and at pH 10.0 and agitation time of 1 h (). After these agitation times, for both pH levels, protein solubility decreased. In this case, solubility behavior can be explained by the combination of conditions in the medium and agitation time. Protein solubility is influenced by the pH of the solutions, which alters the distribution of liquid charges on the surface of the protein and, therefore, affects the water-protein and protein-protein interactions.[Citation5] Diverse physical parameters, such as operational conditions, can also influence protein solubility. Therefore, attention must be paid to agitation and other physical conditions that can denature the proteins.[Citation10]
At the pH levels of 3.0 and 10.0 (), for all agitation times analyzed, increase in salt concentration diminished protein solubility due to the salting-out effect. In this case, competition between proteins and salt ions for water molecules was verified, inducing the removal of hydrating water from the protein and, therefore, causing more hydrophobic interactions (protein-protein) then protein-water interactions. Therefore, this competition causes the aggregation of protein molecules known as precipitation.[Citation5]
At pH 4.6 for the agitation times of 1 to 2 h and salt concentrations between 0.05 and 0.3 mol/L, protein solubility increased due to the salting-in effect (). In this phenomenon, salt ions interact with opposite charged groups in the protein to form a double layer of ionic groups, which diminish the electrostatic interaction between the molecules, causing greater protein solvation and, therefore, increasing solubility.[Citation6,Citation10] When salt concentrations were greater than 0.3 mol/L (0.5 and 1.0 mol/L), reduction in solubility was observed (). In high salt ion concentrations, the majority of the water molecules are strongly bonded to the salt ions, while some water molecules are reorganized around the protein molecules, increasing the hydrophobic interactions and the protein-protein aggregations.[Citation10] At the same pH value of 4.6, for the agitation time of 0.5 h, increasing the salt concentration reduced solubility due to the salting-out behavior.
At pH 6.2, for all evaluated agitation times, increase in salt concentration to 0.3 mol/L increased protein solubility due to the salting-in effect (). At these conditions, protein-water interactions, which increased due to electrostatic forces between the molecules, were greater meaning that the repulsive forces between the molecules increased and a greater quantity of water interacted with the protein molecules. At salt concentrations greater than 0.5 mol/L, protein solubility decreased due to the salting-out effect. According to Collins,[Citation24] an explanation for this phenomenon is that the salt ions sequestered the water molecules, which solvate the protein surface. Consequently, attractive forces (hydrophobic) formed between the protein molecules increasing the quantities of protein-protein interactions and aggregations following precipitation
In the mediums in which pH was maintained at 8.0 with agitation times of 0.5 and 1 h, solubility increased with the elevation in NaCl concentration from 0.05 to 0.5 mol/L (). Salt concentrations greater than 0.8 mol/L induced a decrease in solubility. For the agitation times of 1.5 and 2 h, solubility decreased with the increase in salt concentration. Low salt concentrations generally caused an increase in solubility since low concentrations of salt ions tend to associate themselves with the proteins, contributing to greater hydration and/or repulsion of the protein molecules, provoking the salting-in effect. Contrarily, in elevated salt concentrations, salt ions form a hydration layer and compete with the protein for water, causing hydration water loss from the protein molecules, known as the salting-out phenomenon.[Citation10]
Solubility profiles for each agitation time, as a function of pH and salt concentration, are shown in –. For 0.5 h of agitation (), it was observed that in alkaline media, total solubility was not reached when comparing the results for the solubility profile during 1 h (). After 1 h of agitation, maximum solubility was achieved for the pH levels of 4.6 and 10.0. An increase in agitation time to 1.5 and 2.0 h ( and ) led to a decrease in solubility for all pH levels, indicating protein denaturation since agitation time caused modifications in the protein structure. These changes in the protein molecules release hydrophobic amino acids, which increase protein-protein interactions, thus reducing solubility. Levels of denaturation are directly related to the physical and chemical properties and functionality of the proteins since the disassociation of their structure interferes with these properties.
FIGURE 1 Protein solubility of quail egg white after 0.5 h of agitation time, as a function of NaCl concentration and pH.
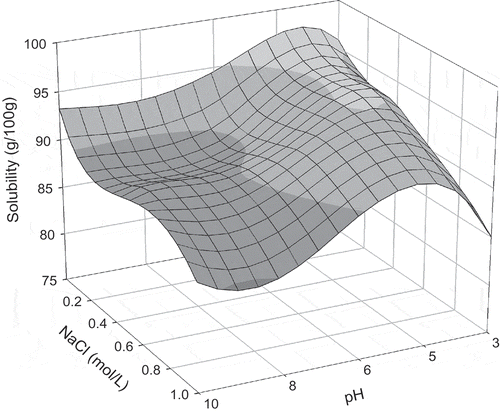
FIGURE 2 Protein solubility of quail egg white after 1.0 h of agitation time, as a function of NaCl concentration and pH.
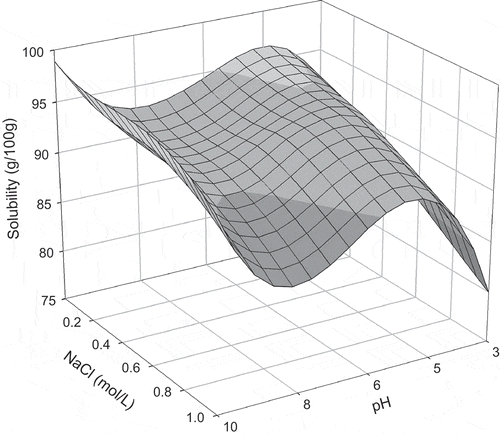
FIGURE 3 Protein solubility of quail egg white after 1.5 h of agitation time, as a function of NaCl concentration and pH.
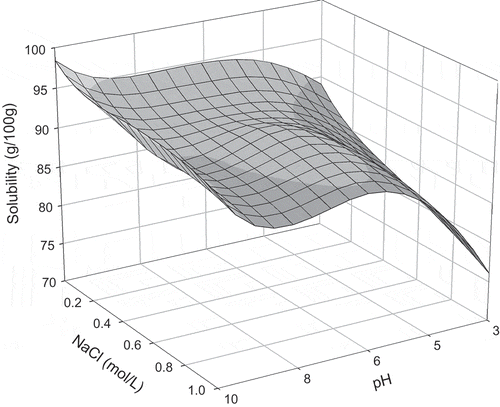
FIGURE 4 Protein solubility of quail egg white after 2.0 h of agitation time, as a function of NaCl concentration and pH.
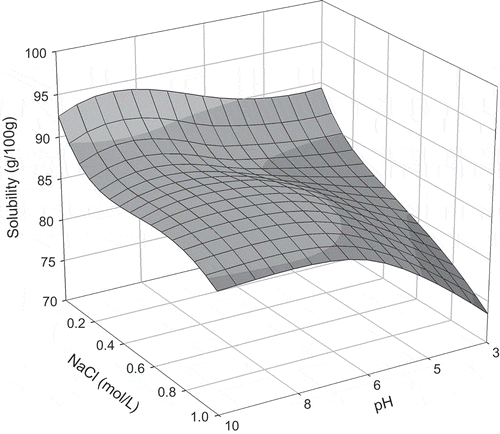
The extent to which the experimental factors (agitation time, NaCl concentration, and pH values) affected the solubility parameter was quantified by fitting the data to a second-order polynomial equation for each agitation time of 0.5, 1.0, 1.5, and 2.0 h as Eqs. (2), (3), (4), and (5), respectively.
where Cs is the NaCl concentration (mol/L), pH is the pH level, and a–e are estimated constants for the equation. The model proposed in Eqs. (2–5) was significant (p < 0.001) to illustrate the variation in solubility of quail egg white in function of pH, salt concentration, and agitation time, presenting R2 values greater than 0.89, 0.92, 0.96, and 0.98 of Eqs. (2–5), respectively. shows the estimated coefficients for Eqs. (2–5).
The sign and magnitude of the coefficients indicate the effect of the variable on the response. A negative coefficient means a decrease in response when the level of the variable is increased, whereas a positive coefficient indicates an increase in the response. A significant interaction suggests that the level of one of the interactive variables may increase, while that of the other may decrease for a constant value of the response.[Citation25] A significant interaction was observed for pH-pH and pH-Cs terms. Solubility of protein in all agitation time had a greater influence on pH parameter.
CONCLUSION
Determination of protein solubility data has shown to be relevant for the definition of food processing conditions, which contain different protein sources, protein concentration, and purification of proteins of high aggregated value. The solubility of proteins from quail egg white was influenced by variations in agitation time, pH, and salt concentration, with a distinguished behavior for each condition. The highest solubility value (98.92 g/100 g) was obtained for the sample containing 0.05 mol/L of NaCl, pH 10.0, and with 1 h of agitation. The lowest solubility value (68.35 g/100 g) was exhibited in the sample containing 1.0 mol/L of NaCl, pH 3.0, and after 2 h of agitation. These test conditions of NaCl 1.0 mol/L, pH 3.0, and 2 h of agitation, appeared to indicate the best conditions for the separation of proteins from quail egg white.
ACKNOWLEDGMENTS
The authors wish to acknowledge the National Council of Technological and Scientific Development (CNPq) and the Research Support Foundation of Minas Gerais (FAPEMIG) for their financial support.
REFERENCES
- Itoh, T.; Kobayashi, S.; Sugawara, H.; Adachi, S. Some physico-chemical changes in quail egg white during storage. Poultry Science 1981, 60, 1245–1249.
- Hertrampf, J. Quails in Asia. Poultry International 1987, 26 (5), 114–122.
- Stadelman, W.J.; Cotterill, O.J. Egg Science and Technology, 4th Ed.; AVI Publisher Company: Westport, CT, 1995; 449.
- Albino, L.F.T.; Barreto, S.L.T. Criação de Codorna Para Produção de Ovos e Carnes, 1st Ed.; Aprenda Fácil: Viçosa, Brazil, 2003; 23–24.
- Fennema, O.R. Food Chemistry, 3rd Ed.; Marcel Dekker: New York, 1996; 1067.
- Kinsella, J.E. Relationships between structure and functional properties of food proteins. In: Food Proteins, 1st Ed.; Fox, P.F.; Condon, J.J.; Eds.; Applied Science Publishers: London, UK, 1982; 51–103.
- Pelegrine, D.H.G.; Gasparetto, C.A. Whey proteins solubility as function of temperature and pH. LWT–Food Science and Technology 2005, 38, 77–80.
- Sousa, R.C.S.; Coimbra, J.S.R.; Rojas, E.E.G.; Minim, L.A.; Oliveira, F.C.; Minim, V.P.R. Effect of pH and salt concentration on the solubility and density of egg yolk and plasma. LWT–Food Science Technology 2007, 40 (7), 1253–1258.
- Kakalis, L.T.; Regenstein, J.M. Effect of pH and salts on the solubility of egg white protein. Journal of Food Science 1986, 51 (6), 1445–1447.
- Vojdani, F. Solubility. In: Methods of Testing Protein Functionality, 1st Ed.; Hall, G.M.; Ed.; Blackie Academic and Professional: London, UK, 1996; 11–46.
- Wong, W.S.; Camirond, W.M.; Pavlath, A.E. Structures and functionality of milk proteins. Critical Reviews in Food Science and Nutrition 1996, 36 (8), 807–844.
- Mann, B.; Malik, R.C. Studies on some functional characteristics of whey protein-polysaccharide complex. Journal of Food Science and Technology 1996, 33 (3), 202–206.
- Machado, F.F.; Coimbra, J.S.R.; Rojas, E.E.G.; Minim, L.A.; Oliveira, F.C.; Sousa, R.C.S. Solubility and density of egg white proteins: Effect of pH and saline concentration. LWT–Food Science Technology 2007, 40 (7), 1304–1307.
- Biasutti, E.A.R.; Vieira, C.R.; Capobiango, M.; Silva, V.D.M.; Silvestre, M.P.C. Study of some functional properties of casein: effect of pH and tryptic hydrolysis. International Journal of Food Properties 2007, 10, 173–183.
- Judge, R.A.; Johns, M.R.; White, E.T. Solubility of ovalbumin in ammonium sulfate solutions. Journal of Chemical Engineering Data 1996, 41 (3), 422–424.
- Anton, M.; Gandemer, G. Composition, solubility and emulsifying properties of granules and plasma of egg yolk. Journal of Food Science 1997, 62 (3), 484–487.
- Santos, D.O.; Coimbra, J.S.R.; Sousa, R.C.S.; Silva, C.A.S.; Barreto, S.L.T; Minim, L.A. Solubility of quail (Coturnix coturnix japonica) egg white protein. Journal of Food Process Engineering 2008, 31, 684–693.
- Gornall, A.G.; Bardawill, C.J.; David, M.M. Determination of serum proteins by means of the biuret reaction. Journal of Biological Chemistry 1949, 177, 751–766.
- Mohan, C. Buffers: A Guide for the Preparation and Use of Buffers in Biological Systems, 1st Ed.; Calbiochem–EMD: San Diego, CA, 1995; 18–30.
- Morr, C.V.; German, B.; Kinsella, J.E.; Regenstein, J.E.; Van Buren, J.P.; Kilara, A.; Lewis, B.A.; Mangino, M.E. A collaborative study to develop a standardised food protein solubility procedure. Journal of Food Science 1985, 50 (6), 1715–1718.
- SAS Institute, Inc. SAS/STAT User’s Guide, Version 9.0; SAS Institute, Inc.: Cary, NC, 2004.
- Kato, A.; Ibrahim, H.R.; Watanabe, H.; Honna, K.; Kobayashi, K. New approach to improve the gelling and surface functional properties of dried egg white by heating in dry state. Journal of Agricultural and Food Chemistry 1989, 37 (2), 433–437.
- Li-Chan, E.; Nakai, S. Biochemical basis for properties of egg white. Critical Reviews in Poultry Biology 1989, 2 (1), 21–57.
- Collins, K.D. Ions from the Hofmeister series and osmolytes: Effects on proteins in solution and in the crystallization process. Methods 2004, 34, 300–311.
- Montgomery, D.C. Design and Analysis of Experiments; John Wiley & Sons: New York, 2004.