Abstract
Starch isolated from white sorghum (nWSS) was subjected to chemical modifications to prepare acid thinned (aWSS), oxidized (oWSS), and dual modified acetylated-oxidized (aoWSS) white sorghum starch. Chemical modifications resulted in roughness on the surface of sorghum starch granules. Solubility and paste clarity improved significantly due to the three modifications. Pasting temperature, peak viscosity, and time to reach peak viscosity of modified starches was significantly less than nWSS. Reassociative tendency was curtailed in modified starches as is evident by the decline in setback viscosity. At 8% starch concentration, oWSS and aoWSS completely failed to form a proper gel network.
Keywords:
INTRODUCTION
White sorghum (Sorghum bicolor), a nonceliac cereal grain, is cultivated extensively in Asia and Africa. The grain is used as food in developing countries and as animal feed in developed countries of the world.[ Citation 1 ] Sorghum grains like millets are the toughest of all cereal crops as they can endure extremely hot and dry weather conditions. It can also tolerate salinity better than maize.[ Citation 2 ] Moreover, sorghum was found to be superior to maize under moderate irrigation conditions.[ Citation 3 ] Starch is the major component of sorghum kernel,[ Citation 4 ] and is similar to corn starch in physicochemical properties.[ Citation 5 ] Due to high drought tolerance of sorghum, this underutilized source of starch can prove to be a beneficial crop in the future as water resources are limited and world population continues to rise.
Starch is an extensively used biopolymer both in food and nonfood industries. However, native starches have limited uses owing to retrogradation, low paste clarity, and sensitivity to pH, heat, and shear.[ Citation 6 ] Therefore, starches are commonly tailored via physical and chemical modifications or both to improve native functional properties and thereby extending its applications in food industries. Modified food starches are used as an additive in sauces, spreads, coatings, films, beverages, snacks, confectionary, dairy, and bakery products.[ Citation 7 ] Acetylated, oxidized, acid thinned, cross linked, and hydroxypropylated starches are the most commonly used modified starches. Though a lot of work has been done to improve the functional properties of tapioca, potato, and corn starches, considerably less work has been done to improve the physicochemical properties of white sorghum starch via chemical modifications.
The aim of the present study was to modify the isolated white sorghum starch by the techniques of chemical modifications like acid thinning, acetylation, and oxidation and to evaluate the effects of these modifications on the morphological, physicochemical, and pasting properties of white sorghum starch.
MATERIALS AND METHODS
Cleaned and sound white sorghum grains from a single cultivar, free from molds and insects were used for isolation of starch. All chemicals used were reagent grade.
Isolation of White Sorghum Starch
Starch was isolated by combining wet milling procedures reported by Olayinka et al.[ Citation 8 ] and Wang et al.[ Citation 9 ] Starch isolation from white sorghum grains was carried out in two steps: (a) steeping and (b) wet milling. Cleaned grains were steeped in 0.5% lactic acid and 0.2% SO2 solution for 24 h at 50°C. The steeped grains were then washed twice for the complete removal of steeping solution. Grains were then coarsely ground and blended in a Waring blender for 5 min at 8000 rpm. The slurry thus obtained was adjusted to pH 8.5 using 0.5M NaOH solutions and was allowed to stand for 30 min. It was then sieved through a stack of 80, 170, and 270 wire mesh sieves from top to bottom. The overs from the sieves were reblended in a Waring blender for 2 min followed by sieving. This step was repeated twice. The starch slurry thus collected was homogenized using a homogenizer (Polytron PT 2100, Kinematica Inc., Switzerland) for 60 s and starch was allowed to sediment at 4°C. The protein layer was scraped off using a spatula. The sedimented starch was washed with water and homogenized again to remove the residual protein. The washed starch was then dried at 45°C in a forced air oven.
Chemical Modifications
Acid thinning
Acid thinning was carried out using the procedure of Gunaratne and Corke.[ Citation 10 ] A 40% (dry basis) starch slurry was acid modified using 0.1M HCl at 50°C in a water bath with constant stirring for 1.5 h. The slurry was then adjusted to a pH of 7 using 0.5M NaOH. The sedimented starch was washed at least five times with distilled water, followed by drying at 45°C in a forced air oven.
Oxidation
The method of Forssel et al.[ Citation 11 ] with some modifications was employed for preparing oxidized white sorghum starch. Briefly, 100 g of white sorghum starch was dispersed in 200 ml of distilled water. The pH of the slurry was adjusted to 9.5 using 2M NaOH. Then, 10 g of NaOCl was added dropwise to the stirred slurry while maintaining a pH between 9.0–9.5. The reaction was allowed to proceed for 10 min after complete addition of NaOCl. The pH was then adjusted to 7.0 using 1M H2SO4. The starch was then allowed to sediment. The sedimented starch was washed at least four times with distilled water followed by drying at 45°C in a forced air oven.
Dual modified acetylated oxidized starch
Oxidized white sorghum starch prepared by using the aforementioned method was further modified by acetylation using the procedure of Philips et al.[ Citation 12 ] to prepare dual modified acetylated oxidized starch.
Determination of Carboxyl Content
Carboxyl content was determined by using the modified procedure of Chattopadhyay et al.[ Citation 13 ] First, 2 g (dry basis) of oxidized white sorghum starch was dispersed in 25 ml of 0.5M HCl. The starch slurry was stirred for 30 min followed by filtration through a medium porosity fritted glass funnel. The residue obtained was washed with 400 ml of distilled water. The starch cake was then quantitatively transferred to a 500-ml glass beaker and the volume was adjusted to 300 ml with distilled water. The starch slurry was then heated with constant stirring in a boiling water bath to ensure complete gelatinization. The hot starch dispersion was immediately titrated with standardized 0.01M NaOH to phenolphthalein end point. A similar procedure for blank test was determined on unmodified white sorghum starch. Carboxyl content was calculated using the following formulas:
Determination of Carbonyl Content
Carbonyl content was determined using the procedure of Smith.[ Citation 14 ] To summarize, 2 g (dry basis) of oxidized starch dispersed in 100 ml of distilled water was gelatinized in a boiling water bath for 2 min and then cooled to 40°C. The pH of the starch slurry was adjusted to 3.2 followed by the addition of 15 ml of hydroxylamine reagent. The gelatinized starch solutions were then placed in a water bath at 40°C with slow stirring. Hydroxylamine reagent was prepared by dissolving 15 ml of reagent grade hydroxylamine hydrochloride in 100 ml of 0.5M NaOH. The volume of solution was then adjusted to 500 ml with distilled water. After 4 h, excess hydroxylamine was determined by rapid titration to pH 3.2 using standardized 0.1M HCl. A blank determination with only hydroxylamine reagent was conducted using the similar procedure. The carbonyl content was determined using the following formula:
Determination of Acetyl Content and Degree of Substitution (DS)
Acetyl content (%) and degree of substitution of acetylated oxidized starch was determined using the procedure of Wurzburg.[ Citation 15 ]
Morphological Properties
Granular morphology of native and acetylated white sorghum starches was studied through scanning electron microscopy (SEM) by sprinkling a thin layer of starch on an aluminum specimen holder by a double-sided tape. The starch was then coated with gold and examined under 1600× and 8000× magnifications.
Swelling Power and Solubility
A starch suspension (2%) w/v was prepared in centrifuge tubes and weighed (W 1). The tubes were then heated to 60, 70, 80, and 90°C for 30 min with intermittent mixing in a thermostat controlled water bath. The tubes were then allowed to cool followed by centrifugation at 1290 g for 15 min. The supernatant was decanted and tubes were reweighed (W 2). The swelling power was calculated using the following formula:
Solubility of starch was determined using the method of Bello-Perez et al.[ Citation 16 ]
Water Retention Capacity (WRC)
Water retention capacity was determined using the method of Bryant and Hamaker[ Citation 17 ] with slight modifications. A starch slurry of 5% (w/v) was prepared in preweighed centrifuge tubes. The tubes were heated to 60, 70, 80, and 90°C for 15 min in a water bath with intermittent mixing. The tubes were centrifuged for 15 min at 1684 g. After decantation of water, the tubes were allowed to drain off for 10 min by placing at a 45° angle. The gain in weight after heating was used to calculate the WRC of starches.
Micro-Viscoamylography
Pasting properties of native, acid-thinned, oxidized, and acetylated-oxidized white sorghum starches were studied using a Brabender Micro-Viscoamylograph (Model 803201, Brabender, Germany) equipped with a 300 cmg sensitivity cartridge. Briefly, 8% starch (dry basis) in 100 ml of distilled water was heated from 40 to 95°C at a heating rate of 3°C/min. The slurry was kept at this temperature for 10 min and subsequently cooled back to 50°C at a cooling rate of 3°C/min. The slurry was held at 50°C for 10 min.
Paste Clarity
The method of Perera and Hoover[ Citation 18 ] was used for determining paste clarity. Initially, 1% (w/v) starch slurry was prepared in centrifuge tubes and was heated in a boiling water bath for 1 h with constant stirring. The tubes were then cooled and (%T) of gelatinized starch solutions were measured at 650 nm against water as a blank using a JASCO V-670 spectrophotometer. The tubes were then stored at 4°C and (%T) was measured after every 24 h for 3 days.
Gel Hardness
The starch gels prepared through microviscoamylography were poured in plastic bowls having a 3.5-cm internal diameter. The gels were covered with parafilm and stored at 4°C. Gel hardness was determined using a universal testing machine (Zwick/Roell, GmbH & Co, D-89079 Ulm). The gel was compressed at a speed of 1 mm/s to a distance of 5 mm using a 5-mm cylindrical probe. The gel hardness was measured after 1, 3, and 7 days of refrigerated storage.
Statistical Analysis
Analysis of variance was used to calculate significant differences between the means, and Duncan's test at P ≤ 0.05 was used to separate means using SPSS software (version 17, SPSS Inc., USA).
RESULTS AND DISCUSSION
Morphological Properties
Scanning electron micrographs of native and modified white sorghum starches under 1600× and 8000× magnifications are presented in and , respectively. Native white sorghum starch (nWSS) granules appear to be spherical and polygonal in shape (). There is a mixed population of small and large granules. The size of nWSS granules ranged between 7–22 μm. Pores are clearly visible on the surface of nWSS (). Huber and BeMiller[ Citation 19 ] and Singh et al.[ Citation 20 ] also reported the presence of pores on the surface of sorghum starches. Similar pores are also present on the surface of maize and millet starch granules.[ Citation 21 ] The size of these pores ranged between 178–289 nm for nWSS. According to Fannon et al.,[ Citation 22 ] pores present on the periphery of starch granules are basically opening to serpentine channels that penetrate into the interior of starch granules.
Figure 1 Scanning electron micrographs of native and modified starches under 1600× magnification: (a) nWSS, (b) aWSS, (c) oWSS, and (d) aoWSS.
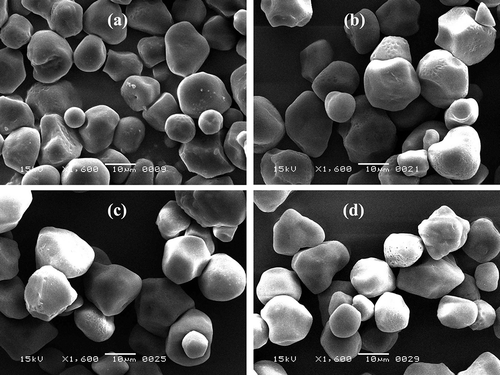
Figure 2 Scanning electron micrographs of native and modified starches under 8000× magnification: (a) nWSS, (b) aWSS, (c) oWSS, and (d) aoWSS.
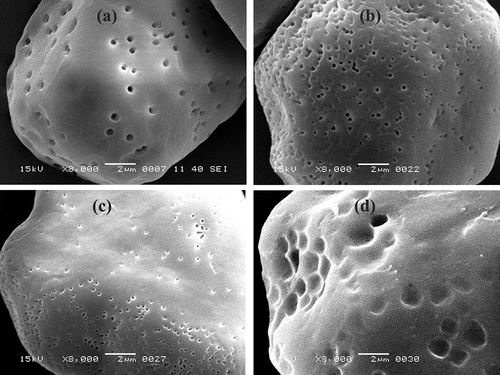
All forms of modifications retained the granular structure of starch, however, a slight distortion of granule shape is obvious in –. Concave curvatures and dents appeared as a result of all three modifications. None of the modifications significantly affected the size of the white sorghum starch granules. Starch granules observed under 8000× magnification () show the effect of modifications on the surface of starch granules. Erosion and roughness on the surface was very obvious in acid-thinned (), oxidized (), and acetylated-oxidized starches (). However, compared to oxidized white sorghum starch (oWSS), acid thinning resulted in a higher degree of surface erosion, highlighting the harshness of acid treatment. The size of pores for acid-thinned and oxidized starches ranged between 200–245 nm and 225–275 nm, respectively. Dual modification of oxidized starch via acetylation further increased the unevenness and roughness on the surface of oxidized white sorghum starch granules. Moreover, the size of pores ranged between 300–438 nm for aoWSS. These pores were larger in size compared to nWSS. The insertion of carboxyl, carbonyl, and acetyl groups in acetylated-oxidized starch (aoWSS) alter the inter and intra granular interactions within the starch granules that could have resulted in altered microstructure of starch and expansion of surface pores. Therefore, the results of scanning electron micrographs reveal an enhanced effect of dual modification on the morphology and microstructure of starch compared to single-step chemical modifications.
Swelling Power and Solubility
The results of swelling power and solubility of nWSS, oWSS, and aoWSS within a temperature range of 60–90°C is presented in . Swelling power of native and modified white sorghum starch increased linearly with the rise in temperature. Acid-thinned and oxidized starches showed considerably restricted swelling capability compared to nWSS from 70–90°C. Similar results for acid-thinned and oxidized starches have also been reported by Lawal[ Citation 23 ] for cocoyam starches and for jackbean starches by Lawal and Adebowale.[ Citation 24 ] However, swelling power of acid-thinned starches was significantly lower than oxidized starches. During acid treatment, hydroxonium ions primarily attack the amorphous region of the starch granules, which is more readily accessible compared to the crystalline region of starch granules and thereby leaves starch granules with a relatively more crystalline structure.[ Citation 25 ] The increase in relative crystallinity of starch granules after acid-thinning could be responsible for the restricted swelling power of starch granules. Also, the presence of dents and physical damage on the granule surface could have limited the swelling power of acid thinned and oxidized starches. Oxidation is also a depolymerization reaction. The hydrolysis of glycosidic linkages disintegrates the granular structure of starch, resulting in less ability to hold water. The swelling power of aoWSS was significantly higher than aWSS and oWSS within the entire temperature range of 60–90°C. The addition of acetyl groups on oxidized starch chains improves the hydrophilic tendency of starch granules. At 90°C, swelling power of aoWSS almost doubled compared to oWSS.
Table 1 Effect of chemical modifications on the swelling power and solubility of white sorghum starch.1
All forms of modifications increased solubility of white sorghum starch. Solubility of aoWSS > oWSS > aWSS > nWSS was within the entire temperature range of 60–90°C. Oxidation and acid-thinning being depolymerization reactions cause breakdown of starch chains, which eventually results in less restricted leaching of amylose chains and subsequently increases the solubility of starches. Higher solubility of oxidized starch compared to aWSS indicates a weakened granular structure of oWSS. Acetylation of starch following oxidation further weakens the starch granules due to introduction of acetyl groups, which disrupts the hydrogen bonds present between the adjacent starch chains resulting in increased solubility of aoWSS granules. The enlargement of pores in aoWSS due to dual modification could have resulted in quicker penetration of water into the granule and subsequent solubilization of amylose chains, as pores are hollow channels that provide access deep into the interior of starch granules. Solubility, like swelling power, was found to be temperature dependent. The solubility of nWSS, aWSS, oWSS, and aoWSS increased linearly with the rise in temperature with Pearson correlation coefficients of 0.974, 0.970, 0.931, and 0.982, respectively. An increase in temperature unfolds the granular structure of starch due to commotion of hydrogen bonds present between the starch chains.
Table 2 Effect of chemical modifications on the WRC of white sorghum starch.1
Water Retention Capacity (WRC)
The water retention capacity of native and modified starches is shown in . WRC is the measure of the availability of water binding sites.[ Citation 26 ] Acid-thinned starch showed significantly higher WRC compared to nWSS. The acid hydrolysis of starch chains exposes more hydroxyl groups due to breakdown of high molecular weight polymer chains to low molecular weight dextrins, thereby increasing the availability of water binding sites. However, oxidized starches showed reduced WRC compared to nWSS from 80 to 90°C. Hydroxyl groups present on starch chains are the major water binding sites. Oxidation of hydroxyl groups to carbonyl and carboxyl groups could be responsible for the reduced WRC of oWSS. Acetylation of oxidized starches significantly improved WRC from 60–70°C. A rise in temperature progressively increased the WRC of both native and modified starches with Pearson correlation coefficient of 0.960, 0.963, 0.952, and 0.881 for nWSS, aWSS, oWSS, and aoWSS, respectively. A similar time dependent increment in WRC is also reported by Zhang et al.[ Citation 27 ] A rise in temperature disrupts hydrogen bonds owing to higher mobility of polymer chains, which subsequently improves the overall availability of water binding sites.
Table 3 Effect of chemical modifications on the pasting profile of white sorghum starch.1
Pasting Properties
The pasting profile of nWSS, aWSS, oWSS, and aoWSS is presented in . The pasting profile measured through micro-viscoamylography reflects the functional properties of starches. The temperature at which viscosity of starch begins to develop is called pasting temperature. Oxidation of white sorghum starch led to reduced pasting temperature, indicating weakening of starch granules. Acetylation of oxidized starch further reduced the pasting temperature of oxidized starches. The substitution of bulky functional groups interfere with the native inter-molecular binding forces present between the starch chains and thereby weakens the starch granule. Acid-thinning reduced the pasting temperature of starch due to hydrolysis of polymer chains. Also, surface erosion of starch granules after modification may further ease the process of gelatinization as granules are already deformed. All three modifications reduced the peak viscosity of starch. The peak viscosity of nWSS > aWSS > aoWSS > oWSS. Acid-thinning and oxidation being depolymerization reactions reduce chain length of starch chains primarily in amorphous region of starch granules resulting in lower peak viscosity of starch. However, acetylation of oxidized starches increased its peak viscosity owing to increased swelling power (). Time to reach peak viscosity reduced both after single and dual modifications, providing evidence to the fact that aWSS, oWSS, and aoWSS allowed easy percolation of water into the starch granules that led to early development of peak viscosity in these chemically tailored starches. However, the results revealed that acetylation of oxidized white sorghum starch further reduced time to reach peak viscosity. Insertion of acetyl groups create electrostatic repulsion between the starch chains, which subsequently result in less restricted water percolation. The significant increment in pore size of aoWSS, as evident in , could also have eased water percolation into the granules with subsequent decline in time to reach peak viscosity. Hot paste viscosity of modified starches was significantly less than nWSS. Breakdown viscosity reflects the resistance of starch granules against heat and shear. Acid-thinning increased breakdown viscosity of starch compared to nWSS. Acid degradation of amylose chains within amorphous region of starch granules result in increased solubilization (higher exudation of amylose chains) of starch polymer. Similar results are also reported by Lawal and Adebowale.[ Citation 24 ] However, oxidation being a partial depolymerization reaction too, resulted in significantly reduced breakdown viscosity of white sorghum starch, which is contradictory to the results reported by Adebowale and Lawal[ Citation 28 ] and Lawal et al.[ Citation 29 ] While, Kuakpetoon and Wang[ Citation 30 ], Sandhu et al.,[ Citation 31 ] and Adebowale et al.[ Citation 32 ] also reported reduction in breakdown viscosity of starch after NaOCl derived oxidation. Kuakepetoon et al.[ Citation 30 ] attributed this phenomenon to the formation of hemi-acetal and hemi-ketal crosslinks among amylopectin molecules. Formation of these cross links is assumed to overcome the negative effects of depolymerization and, thus, stabilize the swollen granules. aoWSS was found to have higher breakdown viscosity than that of oWSS indicating weakening of granules due to introduction of acetyl groups. Cold paste viscosity and setback viscosity being the measure of reassociative tendency of starch granules were drastically reduced post modifications. The short chains produced via acid hydrolysis possess reduced tendency to reassociate compared to long starch chains as evident by reduction in setback viscosity. The setback viscosity of oWSS was even lower than aWSS. The presence of carbonyl and carboxyl functional groups curtail the reassociation of leached out amylose chains. aoWSS showed a higher setback compared to oWSS, though the setback viscosity was still significantly less than nWSS and aWSS. The increase in setback viscosity due to addition of acetyl groups in oxidized starches could be attributed to increased solubility of aoWSS () that resulted in higher exudation of amylose chains.
Table 4 Effect of chemical modifications on the paste clarity (%T) of white sorghum starch.1
Paste Clarity
The results of paste clarity are presented in . Both single and dual modifications significantly improved the paste clarity of white sorghum starches. However, the effect was more pronounced for dual modifications compared to single modifications. The pastes of acid-thinned starches contained low molecular weight short chains, which facilitate better transmittance of light. Substitution of carbonyl and carboxyl groups combined with depolymerization resulted in higher paste clarity of oWSS. Dual modified aoWSS showed the highest increase in (%T) owing to introduction of acetyl groups in addition to carboxyl and carbonyl groups. Paste clarity decreases on refrigerated storage due to molecular realignment of solubilized starch chains. After 24 h of refrigerated storage, the extent of decrease in (%T) of nWSS, aWSS, oWSS, and aoWSS was 56.2, 72.4, 76.9, and 86.76%, respectively. The high degree of reduction in (%T) of modified starches after 24 h of cold storage could be attributed to considerably higher solubility of modified starches resulting in higher exudation of amylose chains and subsequently increased reassociation of this linear component of starch. A strong positive correlation (R = 0.905) was found between solubility at 90°C and extent of reduction in (%T) after 24 h of cold storage. But thereafter, from 24 to 72 h, the extent of decline in (%T) was curtailed in modified starches. Percent decrease in paste clarity from 24 to 72 h for nWSS, aWSS, oWSS, and aoWSS was found to be 30.4, 27.05, 23.0, and 10.1%, respectively. The presence of bulky functional groups in oWSS and aoWSS creates repulsion between starch chains and thwarts the reassociation of solubilized chains and, therefore, reduces retrogradation tendency of modified starches. Also, depolymerized low molecular weight chains in aWSS reassociate less closely compared to high molecular weight chains in nWSS thus reducing opacity of white sorghum starch.
Gel Hardness
Gel hardness values after 1, 3, and 7 days of refrigerated storage are presented in . At 8% starch concentration, oWSS and aoWSS completely lacked the ability to form gels. Gels are usually formed via entanglement or reassociation of leached out amylose chains via hydrogen bonds together with entrapment of water between the chains. The presence of substituent carboxyl, carbonyl, and acetyl groups on exuded amylose chains perhaps interfered with the reassociation of starch chains resulting in failure to form a proper gel network. This is also evident by low cold paste viscosity () of oWSS and aoWSS. Such starches usually form gel at higher concentration and, thus, could be used in food products where high solid contents are required. Therefore, high concentration of these starches could be incorporated without excessive thickening. The gel strength of aWSS was significantly lower than nWSS after 1, 3, and 7 days of cold storage. Gunaratne and Corke[ Citation 10 ] also reported a similar decline in gel hardness of wheat starch after acid-thinning. The reduced strength of acid thinned starches could be the result of less numerous intermolecular binding forces between the low molecular weight starch chains. Gel strength of nWSS and aWSS increased on cold storage. A similar time dependent increment in gel strength is also reported by Kaur et al.[ Citation 33 ] Retrogradation is amplified when gels are cooled. This is due to the progressive close association of amylose chains. However, even after 7 days of refrigerated storage, the gel strength of aWSS was significantly less than nWSS.
Table 5 Effect of chemical modifications on the gel hardness (N) of white sorghum starch.1
CONCLUSION
The results revealed significant changes in morphological, physicochemical, and pasting properties of white sorghum starch after chemical modifications. Modified starches demonstrated reduced retrogradation tendency as evident by a marked decline in setback viscosity and extent of reduction in (%T) on refrigerated storage, improving the application of these tailored starches in a wide variety of food products. Also, oWSS and aoWSS could find applications in food products where high solid contents without excessive thickening are required.
REFERENCES
- International Crops Research Institute for the Semi-Arid Tropics (ICRISAT) . “ Food and Agriculture Organization (FAO) ” . In The World Sorghum and Millet Economies , FAO: Rome , Italy : ICRISAT: Patan cheru, India . 1996.
- National Research Council . 1996 . Lost Crops of Africa; National Academy Press: Washington 127 – 213 .
- Singh , B.R. and Singh , D.P . 1995 . Agronomic and physiological responses of sorghum, maize and pearl millet to irrigation . Field Crop Research , 42 : 57 – 67 .
- Serna-Saldivar , S. and Rooney , L.W. 1995 . “ Structure and chemistry of sorghum and millets ” . In Sorghum and Millets: Chemistry and Technology , Edited by: Dendy , D.A.V. 69 – 124 . St. Paul , MN : American Asssociation of Cereal Chemists .
- Perez Sira , E.E. , Lares , M. and Gonzalez , Z.M. 1997 . Characterization of starch isolated from white and dark sorghum . Starch-Starke , 49 : 103 – 106 .
- Sajilata , M.G. and Singhal , R.S. 2005 . Specialty starches for snack foods . Carbohydrate Polymers , 59 : 131 – 151 .
- Singh , J. , Kaur , L. and McCarthy , O.J. 2007 . Factors influencing the physico-chemical, morphological, thermal and rheological properties of some chemically modified starches for food applications—A review . Food Hydrocolloids , 21 : 1 – 22 .
- Olayinka , O.O. , Adebowale , K.O. and Olu-Owolabi , B.I. 2008 . Effect of heat-moisture treatment on physicochemical properties of white sorghum starch . Food Hydrocolloids , 22 : 225 – 230 .
- Wang , F.C. , Chung , D.S. , Seib , P.A. and Kim , Y.S. 2000 . Optimum steeping process for wet milling of sorghum . Cereal Chemistry , 77 : 478 – 483 .
- Gunaratne , A. and Corke , H. 2007 . Influence of prior acid treatment on acetylation of wheat, potato and maize starches . Food Chemistry , 105 : 917 – 925 .
- Forssel , P. , Hamunen , A. , Autio , K. , Suortti , T. and Poutanen , K. 1995 . Hypochlorite oxidation of barley and potato starch . Starch-Starke , 47 : 371 – 377 .
- Philips , D.L. , Liu , H. , Pan , D. and Corke , H. 1999 . General application of Raman spectroscopy for the determination of level of acetylation in modified starches . Cereal Chemistry , 76 : 439 – 443 .
- Chattopadhyay , S. , Singhal , R.S. and Kulkarni , P.R. 1997 . Optimisation of conditions of synthesis of oxidized starch from corn and amaranth for use in film-forming applications . Carbohydrate Polymer , 34 : 203 – 212 .
- Smith , R.J. 1967 . “ Characterization and analysis of starches ” . In Starch: Chemistry and Technology , Edited by: Whistler , R.L. and Paschall , E.F. 620 – 625 . New York : Academic Press .
- Wurzburg , O.B. 1978 . “ Starch, modified starch and dextrin ” . In Products of the Corn Refining Industry, Seminar Proceedings , 23 – 32 . Inc.:Washington : Corn Refiners Association .
- Bello-Perez , L.A. , Aparicio-Saguilan , A. , Mendez-Montealvo , G. , Solorza-Feria , G. and Flores-Huicochea , E. 2005 . Isolation and partial characterization of Mango (Magnifera indica L.) starch: Morphological, physicochemical and functional studies . Plant Foods for Human Nutrition , 60 : 7 – 12 .
- Bryant , C.M. and Hamaker , B.R. 1997 . Effect of lime on gelatinization of corn flour and starch . Cereal Chemistry , 74 : 171 – 175 .
- Perera , C. and Hoover , R. 1999 . Influence of hydroxypropylation on retrogradation properties of native, defatted and heat- moisture treated potato starches . Food Chemistry , 64 : 361 – 375 .
- Hubber , K.C. and BeMiller , J.N. 2000 . Channels of maize and sorghum starch granules . Carbohydrate Polymer , 41 : 269 – 276 .
- Singh , H. , Sodhi , N.S. and Singh , N. 2010 . Characterisation of starches separated from sorghum cultivars grown in India . Food Chemistry , 119 : 95 – 100 .
- Fannon , J.E. , Hauber , R.J. and BeMiller , J.N. 1992 . Surface pores of starch granules . Cereal Chemistry , 69 : 284 – 288 .
- Fannon , J.E. , Shull , J.M. and BeMiller , J.N. 1993 . Interior channels of starch granules . Cereal Chemistry , 70 : 611 – 613 .
- Lawal , O.S. 2004 . Composition, physicochemical properties and retrogradation characteristics of native, oxidized acetylated and acid-thinned new cocoyam (Xanthosoma sagittifolium) starch . Food Chemistry , 87 : 205 – 218 .
- Lawal , O.S. and Adebowale , K.O. 2005 . Physicochemical characteristics and thermal properties of chemically modified jack bean (Canavalia ensiformis) starch . Carbohydrate Polymer , 60 : 331 – 341 .
- Thomas , D.J. and Atwell . 1999 . W.A. Starch modifications , 31 – 48 . St. Paul , MN : Starches; Eagan Press, AACC .
- Wotton , M. and Bamunuarachchi , A. 1978 . Water binding capacity of commercial produced native and modified starches . Starch-Starke , 30 : 306 – 309 .
- Zhang , P. , Whistler , R.L. , BeMiller , J.N. and Hamaker , B.R. 2005 . Banana starch: Production, physicochemical properties, and digestibility—A review . Carbohydrate Polymer , 59 : 443 – 458 .
- Adebowale , K.O. and Lawal , O.S. 2003 . Functional properties and retrogradation behaviour of native and chemically modified starch of mucuna bean (Mucuna pruriens) . Journal of the Science and Food Agriculture , 83 : 1541 – 1546 .
- Lawal , O.S. , Adeobowale , K.O. , Ogunsanwo , B.M. , Barba , L.L. and Ilo , N.S. 2005 . Oxidized and acid thinned starch derivatives of hybrid maize: functional characteristics, wide angle X-ray diffractometry and thermal properties . International Journal of Biological Macromloecules , 35 : 71 – 79 .
- Kuakpetoon , D. and Wang , Y. 2006 . Structural characteristics and physicochemical properties of oxidized corn starches varying in amylose content . Carbohydrate Research , 341 : 1896 – 1915 .
- Sandhu , K.S. , Kaur , M. , Singh , N. and Lim , S. 2008 . A comparison of native and oxidized normal and waxy corn starches: Physicochemical, thermal, morphological and pasting properties . Food Science and Technology , 41 : 1000 – 1010 .
- Adebowale , K.O. , Adeniyi Afolabi , T. and Olu-Owolabi , B.I. 2006 . Functional, physicochemical and retrogradation properties of sword bean (Canavalia gladiata) acetylated and oxidized starches . Carbohydrate Polymer , 65 : 93 – 101 .
- Kaur , L. , Singh , J. and Singh , N. 2005 . Effect of glycerol monostearate on the physico-chemical, thermal, rheological and noodle making properties of corn and potato starches . Food Hydrocolloids , 19 : 839 – 849 .