Abstract
The objective of this work was to study the effect of sample oil concentration and volume on hydroperoxide determination in n-3 PUFA-rich sardine-anchovy oil. Oil samples were stored at −20°C, 6 d, air-free (T1); and 20°C, 2 d in air (T2). Significant differences between storage conditions were observed on hydroperoxide concentration. High reproducibility was obtained even in sample concentrations as low as 5 mg oil/mL of propan-1-ol. A high correlation was observed between the modified ferrous oxidation-xylenol orange method (FOX2) and AOAC peroxide value analysis methods, although FOX2 was faster, more sensitive, and precise when analyzing small hydroperoxide concentrations.
INTRODUCTION
Marine lipids are the main source of polyunsaturated fatty acids (PUFA), especially n-3, such as eicosapentaenoic (EPA, C20:5) and docosahexaenoic (DHA, C22:6) acids,[ Citation 1 ,Citation 2 ] having beneficial effects for human health.[ Citation 2 ,Citation 3 ] However, lipid oxidation reactions limit fish oil utilization in processed foods.[ Citation 4 ,Citation 5 ] Intermediate unstable compound production starts free radical and hydroperoxide formation;[ Citation 6 ] cyclic peroxides are further produced by the reaction between hydroperoxides and oxygen.[ Citation 7 ] Aldehydes, ketones, and alcohols responsible for off-odors and flavors form at this stage.[ Citation 8 ] Analysis of hydroperoxide degradation products is usually carried out by gas chromatography or HPLC, or by titration and colorimetric techniques; the main drawback of these methods is the low specificity, mainly due to interference of non-hydroperoxide compounds, present in low concentrations.
Lipid oxidation analytical methods are based on quantifying compounds generated during the initiation, propagation, or termination phases, or on oxygen or substrate consumption. The most widely used method is based on peroxide value (PV) that quantifies total peroxide and hydroperoxide concentrations, formed during the initial oxidation phase. However, its main disadvantage is its low accuracy; the higher the PV value the more unspecific the titration end point. On the other hand, methods based on 2-thiobarbituric acid-reactive substances (TBARS) quantify several aldehydes, malonaldehyde among them. Low TBARS numbers are not a reliable oil quality index as aldehyde generation time can be longer than expected or aldehyde reactivity may undergo modifications during sample storage or analysis time, hence, aldehydes are not completely detected.[ Citation 9 ]
As the ferrous oxidation-xylenol orange method (FOX) is based on analyzing hydroperoxide oxidation, it is possible to adapt it to a simpler, reproducible, and accurate approach. The FOX method is based on quantifying hydroperoxide radicals capacity to change ferrous to ferric ions, with subsequent formation of a complex with xylenol orange (XO) able to be spectrophotometrically quantified. Total hydroperoxide concentration and oxidation susceptibility can be rapidly analyzed. It has been reported that the FOX method is accurate and selective for a variety of materials.[ Citation 10 ] A modified ferrous oxidation-xylenol orange method, or FOX2, allows low hydroperoxide concentration detection in the presence of non-peroxidated lipids.[ Citation 11 ] The objective of the present work was to adapt the FOX2 method to analyze hydroperoxide concentrations in sardine-anchovy commercial oil, rich in n-3 PUFA, and to study the effect of oil concentration and volume of solubilized oil on hydroperoxide content.
MATERIALS AND METHODS
Materials
Refined fish oil containing high EPA and DHA concentrations (9.8 and 9.6 g/100 g oil, respectively) obtained from South American anchovy (Engraulis ringens) and sardine (Sardinops sagax) was kindly supplied by Ocean Nutrition Canada Limited (Halifax NS, Canada). Stearic and myristic acid standards were obtained from Fluka (Buchs, Switzerland). Other standards (EPA, DHA, linoleic, oleic, and palmitoleic acids) and reagents (boron trifluoride-methanol, palmitic acid methyl ester, cumene hydroperoxide CHP, xylenol orange tetrasodium salt, and hexahydrated ammonium ferrous sulfate) were obtained from Sigma (St. Louis, MO, USA).
Methods
Fatty acid methyl esters were derivatized according to the method described by Firestone.[ Citation 12 ] Twenty milligram samples were methylated; 6 mL 0.5 N sodium hydroxide in methanol was added to the sample and the mixture boiled for approximately 10 min or until the fat globules dispersed; 7 mL 12% boron trifluoride-methanol (BF3/MeOH) was added and the mixture boiled for 5 min, followed by 1.5 mL hexane addition; boiling continued for another minute. Samples were then placed in an ice bath and saturated NaCl solution added. The organic phase was decanted with a Pasteur pipette placed in 1-mL test tubes, closed tightly, and stored at 4°C for no more than 6 h before analysis by gas chromatography. All reagents were analytical grade.
Methyl ester analysis was carried on in a Varian CP 3800 gas chromatographer (Walnut Creek, CA, USA) equipped with a flame ionization detector, and attached with a Varian Chrompack capillary column WCOT CP WAX 52CB (30 m × 0.25 mm id × 0.25 μm). The operation conditions were: carrier gas (nitrogen) 1.8 mL/min flow; split radio 1:10, 10 psi; 150°C for 2 min oven temperature, up to 240°C, 2°C/min; injector and detector temperatures: 250 and 270°C, respectively. One microliter sample was injected; retention times and peak areas were calculated with a Varian Star Chromatography Workstation program. Fatty acid-methyl ester identification and quantification was carried out by comparing previously methylated standard retention times, and interpolating the peak areas to the corresponding fatty acid standard curve. The results were expressed as fatty acid concentration in the oil sample (g/100 g oil). All analyses were carried out in duplicate.
Two milliliter commercial oil samples were placed in 5-mL amber flasks; the samples were divided into two batches. N2 was injected in flasks of one batch to eliminate air and avoid oil oxidation; the flasks were then closed tightly and stored at −20°C for 6 d (Treatment T1). In the second batch, samples were stored in air at 20°C for 2 d (Treatment T2).
Ferrous oxidation-xylenol orange reagent was prepared according to the method reported by Eymard and Genot.[ Citation 13 ] Hexahydrated ammonium ferrous sulfate [(NH4)2Fe(SO4)2·6H2O] (2.5 mM) was dissolved in 250 mM sulfuric acid; 10 mL of this solution was mixed with 7.6 mg xylenol orange tetrasodium salt (C31H28N2Na4O13S) previously dissolved in 20 mL methanol (HPLC grade). The mixture was taken to 100 mL final volume with methanol (HPLC grade). Xylenol orange, hexahydrated ammonium ferrous sulfate, and sulfuric acid final concentration in the reagent were: 100 μM, 0.25 mM, and 25 mM, respectively. This reagent was prepared immediately before using.
Sardine-anchovy oil (0.75, 1, 2, 5, 10, 20, 40, 80 mg) was dissolved in 1 mL propan-1-ol; 150 μL aliquots were mixed with 1350 μL FOX2 reagent in Eppendorf tubes, incubated at room temperature (approximately 18°C) for 50 min avoiding direct light[ Citation 13 ] and centrifuged at 12000× g for 5 min. The supernatant was carefully decanted and the absorbance read at 560 nm.[ Citation 14 ] The analysis was carried out in triplicate for each oil concentration and storage conditions (T1 and T2).
Linear response intervals for oil content and absorbance in samples stored at T1 and T2 were established. Once the intervals were defined, the aliquot volume effect of oil in propan-1-ol on hydroperoxide was studied. Five and 20 mg of oil was dissolved in 1 mL of propan-1-ol; 25, 50, 75, 100, 125, and 150 μL aliquots were placed in Eppendorf tubes and taken to 150 μL with propan-1-ol; 1350 μL FOX2 reagent was then added. Samples were incubated at room temperature (approximately 18°C) for 50 min, avoiding direct light,[ Citation 13 ] and centrifuged at 12000× g for 5 min. The supernatant was decanted and read at 560 nm[ Citation 14 ] against a blank (150 μL propan-1-ol + 1350 μL FOX2 reagent). A standard curve was plotted from 0 to 15 μM CHP-methanol.[ Citation 13 ] Absorbance was interpolated into a standard curve and hydroperoxide concentration reported as mmol CHP equivalents/kg oil (mmol cumene equivalents/kg oil). All analyses were carried out in triplicate for each oil volume and storage condition (T1 and T2).
PV was expressed as peroxide milliequivalents per kg oil (meq peroxide/kg oil), and analyzed in 5.00 ± 0.05 g oil samples, according to AOAC Official Method 965.33, as described by the Association of Official Analytical Chemists.[ Citation 12 ] Samples were dissolved in glacial acetic acid-chloroform solution (3:2); the obtained mixture reacted with saturated potassium iodide and the formed free iodine was titrated with 0.01 N sodium thiosulfate.
Oxidation of sardine-anchovy oil was carried out by placing oil samples in amber glass flasks under conditions accelerating oxidation reactions (storage in air at 20°C for 30 h). Hydroperoxide concentration was analyzed after 12 h of storage and every 6 h thereafter. Each sample was analyzed by FOX2, as reported in this work, and by the official PV method (AOAC 965.33). All analyses were carried out in triplicate.
Experimental Design and Statistical Analysis
Data were subjected to one-way ANOVA to analyze differences among hydroperoxide concentrations as an effect of oil volume solubilized in propan-1-ol (25, 50, 75, 100, 125, and 150 μL aliquots) analyzed by FOX2. If differences P < 0.05, they were considered significant and Fisher's Least Square Difference (Fisher LSD) mean comparison test was applied. The analysis was carried out separately to each one of the following conditions: (1) 5 mg fish oil/mL propan-1-ol, T1; (2) 5 mg fish oil/mL propan-1-ol, T2; (3) 20 mg fish oil/mL propan-1-ol, T1; and (4) 20 mg fish oil/mL propan-1-ol, T2. Once obtaining reproducibility for hydroperoxide concentrations, these data were allocated to a nested two-way analysis of variance to detect possible differences (mmol cumene equivalents/kg oil) in 5 mg oil/mL propan-1-ol (P < 0.05), as an effect of solubilized oil volume at a given storage condition on hydroperoxide concentration. The main block was the storage condition (T1: −20°C, 6 days, no air; T2: 20°C, 2 days, with air) and the sub-blocks were oil volume aliquots solubilized in propan-1-ol at T1 (100, 125, and 150 μL), and at T2 (75, 100, and 125 μL). Storage conditions, oil aliquot volume solubilized in propan-1-ol, and replicates were compared. Hydroperoxide concentrations, as obtained by AOAC's PV method and FOX2 were subjected to analysis of variance and linear regression (P < 0.01). FOX2 sensitivity to detect small hydroperoxide concentrations was analyzed by a correlation analysis, using Minitab Statistical Software for Windows release 11 (Minitab Inc.).
RESULTS AND DISCUSSION
Fatty Acid Composition of the Commercial Sardine-Anchovy Oil
The most abundant fatty acids were (g/100 g oil): palmitic (15.8), EPA (9.8), DHA (9.6), oleic (9.6), palmitoleic (8.9), and stearic (8.1) (). Total saturated fatty acids (SFA) content was 31.2 g/100 g oil. Higher SFA concentration was reported by Gámez-Meza et al.[ Citation 15 ] in sardine caught in the Gulf of California (31.9%). Turan et al.[ Citation 16 ] found 33.25 to 33.61% total SFA in anchovy, palmitic acid being the most abundant (19.96 to 20.66%).
Table 1 Fatty acid composition of the analyzed commercial sardine-anchovy oil
Total monounsaturated fatty acids (MUFA) in our samples was 18.5 g/100 g oil, the most abundant were oleic and palmitoleic (9.6 and 8.9 g/100 g oil, respectively). Turan et al.[ Citation 16 ] reported MUFA content in anchovy oil ranging from 20.23 to 21.62%, oleic acid showing the highest concentration (12.29 to 13.44%). PUFA were more concentrated in our samples (21.4 g/100 g oil) than MUFA (18.5 g/100 g oil), conversely to the results reported by Kartal et al.[ Citation 17 ] who pointed out that, in general, MUFA are more abundant than PUFA in sardine, mackerel, and commercial marine oil mixtures. However, PUFA content in the studied oil was similar to the results reported by other authors (19.095 to 42.484%).
Total n-6 and n-3 fatty acid concentration in the analyzed oil was 2.0 g and 19.4 g/100 g oil, respectively. Zlatanos and Laskaridis[ Citation 18 ] reported 2.72 g/100 g total n-6 fatty acid concentration in sardine and 2.34 g/100 g in anchovy oil. EPA (9.8 g/100 g oil) and DHA (9.6 g/100 g oil) in the studied samples was 91% total PUFA. A similar amount was reported by Gámez-Meza et al.[ Citation 15 ] in Gulf of California sardine oil (86.5%); Kartal et al. [ Citation 17 ] also found higher n-3 and lower n-6 PUFA concentrations in anchovy oil. EPA concentration reported in sardine, anchovy, and picarel by other authors[ Citation 18 ] were 10.67, 7.75, and 9.26 g/100 g, respectively, whereas DHA found by the same authors were 20.8, 21.08, and 18.44 g/100 g, respectively. Differences in n-3 concentrations may be due to ambient and seasonal factors,[ Citation 19 ] as well as weather fishes were wild or cultivated.[ Citation 17 ,Citation 18 ]
Oil Concentration
Absorbance increased with oil concentration in samples (); a linear relationship was observed between 0.75 to 10 mg, and 10 to 80 mg in samples stored at T1, and between 0.75 to 10 mg for T2. Martínez Téllez et al.[ Citation 20 ] found a linear relationship between ozone-treated sunflower oil concentration and absorbance at 560 nm within 2 to 17 μg oil. A positive-slope (R Citation 2 = 0.9926) was observed at T1; conversely, absorbance above 10 mg at T2 conditions deviate from linearity. As oil was in excess, it did not completely solubilized in methanolic FOX2 reagent, at 91/9 methanol/water ratio (v/v).[ Citation 13 ] Our results agreed with those reported by Nourooz-Zadeh et al.,[ Citation 14 ] who found deviations from linearity when analyzing olive oil by FOX2 if oil concentrations were above 20 mg.
Figure 1 Effect of oil concentration on absorbance linear response by the adapted FOX2 method in sardine-anchovy commercial oil: (T1) −20°C, 6 d, air-free; (T2) 20°C, 2 d, air.
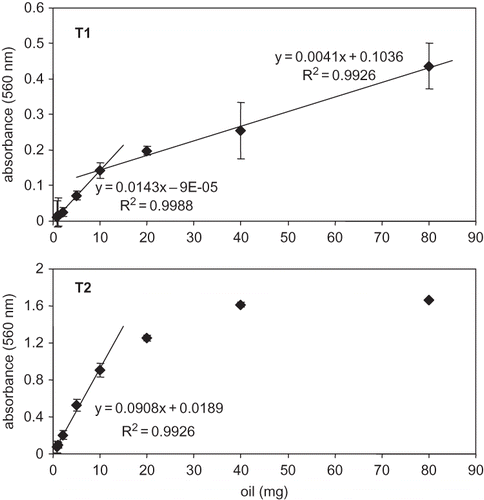
As the studied sardine-anchovy oil is rich in palmitic acid and n-3 PUFA, especially EPA and DHA, it is expected that samples have hydrophobic properties and high miscibility in low polarity solvents. Okuyama et al.[ Citation 21 ] reported that DHA and arachidonic acid-containing phospholipids provide more compact structures as compared to acylglycerides esterified with other PUFA. Due to steric hindrance, less fatty acid molecules are esterified to glycerol. In addition, membranes containing PUFA-esterified phospholipids are able to form highly hydrophobic interfaces. For this reason, miscibility was reduced if oil concentration was too high. As a result, plots of oil concentration versus absorbance deviate from linearity.
Effect of Oil/Propan-1-ol Volume
Propan-1-ol is more hydrophobic than methanol and miscible in water; its high hydrophobicity facilitates solubility of DHA and EPA-containing phospholipids.[ Citation 22 ,Citation 23 ] Due to the fact that FOX2 reagent contains methanol, lipid hydroperoxides are more efficiently recovered, as peroxide polarity is higher than non-oxidized lipids.[ Citation 10 ] The effect of soluble oil volume in hydroperoxide analysis is shown in . Absorbance is increased with volume from 25 to 150 μL in 5 mg oil/mL propan-1-ol samples, stored at T1 and T2 (). Grau et al.[ Citation 24 ] reported that poultry oxidation products followed a linear relationship between absorbance and meat extract volume (50 to 150 μL).
Figure 2 Effect of soluble oil sample aliquot on hydroperoxide analysis linear response in sardine-anchovy commercial oil by the adapted FOX2 method. (a) 5 mg oil/mL propan-1-ol; (b) 20 mg oil/mL propan-1-ol.
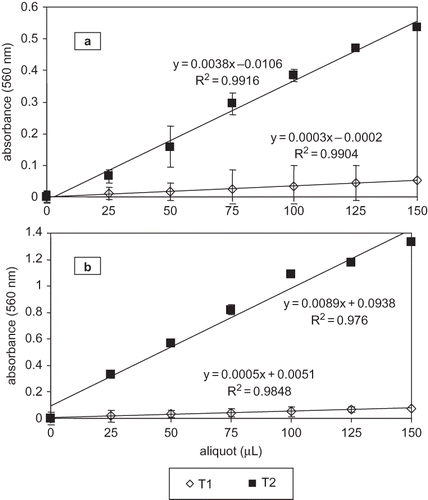
Linearity was also observed in our study when analyzing 20 mg oil/mL propan-1-ol samples, stored at T1 and T2 (). However, conversely as in 5 mg oil/mL propan-1-ol samples, R Citation 2 was lower for T1 (0.984) and T2 (0.976). FOX2 accurately quantified (P < 0.05) hydroperoxide concentrations in 5 mg oil/mL propan-1-ol samples in 100, 125, and 150 μL stored at T1, and 75, 100, and 125 μL stored at T2 (). Hydroperoxide concentrations were significantly different in samples stored at T1 and T2 (P = 0.007); also Fisher LSD showed that if aliquots were lower than 100 μL for T1, and 75 μL for T2, hydroperoxide concentrations were underestimated. This analytical error did not occur in higher volumes.
Figure 3 Effect of soluble oil sample aliquot on hydroperoxide concentration (mmol cumene equivalents/kg oil) in sardine-anchovy commercial oil by the adapted FOX2 method. (a) 5 mg oil/mL propan-1-ol; (b) 20 mg oil/mL propan-1-ol.
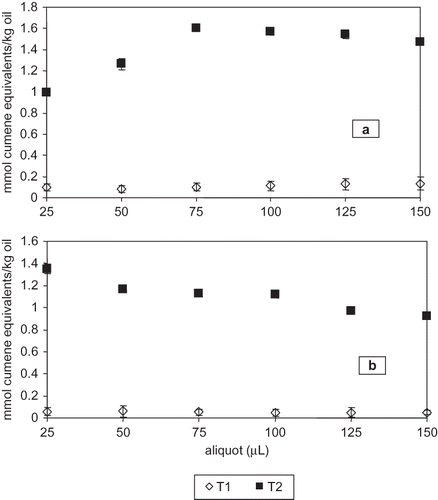
Hydroperoxide concentrations, expressed as mmol cumene equivalents/kg oil, for T1 and T2 are shown in . No significant difference (P = 0.978) was observed at T1 for 20 mg oil/mL propan-1-ol and 25, 50, 75, 100, 125, and 150 μL, as an effect of soluble oil volume (). Conversely, a significant difference (P < 0.0001) was observed at T2 when the soluble oil volume was 25 to 150 μL; Fisher LSD showed significant differences for 25, 125, and 150 μL.
When analyzing 20 mg oil/mL propan-1-ol (), hydroperoxide concentration results were considerably lower as compared to 5 mg oil/mL propan-1-ol, due to incomplete oil solubilization in methanolic FOX2 reagent. Therefore, hydroperoxides did not exhaustively react; as a result, hydroperoxide concentration was underestimated. However, at T1 and T2 absorbance versus hydroperoxide concentration within 25 to 150 μL also follows a linear relationship. Our results agreed with those obtained by Eymard and Genot,[ Citation 13 ] who analyzed hydroperoxide concentrations in methanol extracts of a pelagic fatty fish by FOX2. The authors found that extract volumes lower than 100 μL underestimate hydroperoxide concentrations in recently caught fish.
Comparison Between Analytical Methods
Once FOX2 analytical conditions for sardine-anchovy oil were established to produce accurate and reproducible results, the method was validated by comparing the data to results obtained from the same samples by AOAC 965.33 method for PV determination.[ Citation 12 ] This comparison was carried out using, as an indicator, hydroperoxide concentrations in oxidized samples. Data were subjected to ANOVA and linear regression. FOX2 sensitivity to detect small hydroperoxide concentrations was analyzed by correlation analysis. Significance was established at P < 0.01.
The analytical response for both methods was described by the regression adjusted model Y = a + bX. Statistical analysis showed a high correlation (R = 0.9809) between PV and FOX2. ANOVA's P-value was less than P < 0.01 (P = 0.0032), showing that the relationship between both methods was statistically significant when applied to sardine-anchovy oil (99% confidence interval). The standard deviation difference, or residual (SDD), was 0.0790 meq peroxide/kg oil (or mmol cumene equivalents/kg oil).
Similar correlation studies between analytical methods were carried out by Talpur et al.[ Citation 25 ] These authors reported a high correlation (R = 0.9952) between a simplified spectrophotometry UV method for PV in canola oil, thermally previously oxidized, and the official hydroperoxide quantification method by titration, reported by AOCS. Although hydroperoxide concentrations obtained by FOX2 were expressed as mmoles CHP equivalent/kg oil (mmol cumene equivalents/kg oil), these results can also be expressed as CHP milliequivalent/kg oil, as ferrous ions oxidize to ferric by CHP in acid medium at room temperature.[ Citation 10 ] In our study, there was no significant difference among replicates on the PV analysis carried out by the AOAC official method (P = 0.3606), or hydroperoxide concentration analyzed by FOX2 (P = 0.9118). Therefore, both methods gave reproducible results. Significant differences were observed with respect to time (P < 0.0001) for hydroperoxide concentration in oil subjected to oxidizing conditions, analyzed by PV and FOX2.
One-way ANOVA followed by Fisher's LSD comparison test (P < 0.05) showed that PV cannot detect small hydroperoxide concentration changes due to oxidation. This lack of sensitivity was not observed for FOX2, as shown in . Thus, a significant increase (P < 0.0001) in oxidation can be detected as oil undergoes oxidation if FOX2 is applied. The increased sensitivity allowed the detection of very small hydroperoxide concentrations, providing information on sardine-anchovy oil susceptibility to oxidation.[ Citation 24 ] According to Talpur et al.,[ Citation 25 ] although the AOCS method is simple and reproducible, in practice it has low sensitivity in quantifying low PV in canola oil, as compared to the UV hydroperoxide analysis.
Table 2 Hydroperoxide concentration in oxidated sardine-anchovy oil, analyzed by the official AOAC (PV) and adapted FOX2 methods
In general, it was observed that hydroperoxide concentrations, as obtained by the PV official AOAC method and expressed as meq peroxide/kg oil, were much higher than results obtained by FOX2. The two main error sources in iodometric analysis, when applied to peroxide determination, are iodine absorption by lipid unsaturated bonds, and liberation of potassium iodide promoted by oxygen in the titratable solution.[ Citation 24 ,Citation 26 ] The reaction rate increases with factors, such as light incidence or presence of peroxide, resulting in unrealistic high hydroperoxide concentrations. Subtracting a “blank” from the results does not cancel the error, as the oxygen effect is catalyzed by peroxides.[ Citation 26 ] This error does not occur in the FOX2 method because it is practically non-sensitive to oxygen.[ Citation 10 ]
CONCLUSIONS
Although storage conditions affect hydroperoxide production in n-3 PUFA-rich oils, analysis accuracy mainly depends on a combination of conditions that promote oxidation. The adapted FOX2 method allows a simple, fast, sensible, and accurate way to carry out oxidation analysis of primary products in sardine-anchovy oil, rich in n-3 PUFA, mainly EPA and DHA. This method highly correlates with AOAC's hydroperoxide analysis (PV) and can be validated for other marine oils, although oil mass and soluble oil volume added to the reaction mixture must be defined for each sample type.
ACKNOWLEDGMENTS
Violeta Ugalde-Benítez wishes to thank the National Council of Science and Technology (CONACYT, Mexico) for a graduate scholarship.
REFERENCES
- Shiota , M. , Konishi , H. and Tatsumi , K. 1999 . Oxidative stability of fish oil blended with butter . Journal of Dairy Science , 82 : 1877 – 1881 .
- Narayan , B. , Miyashita , K. and Hosakawa , M. 2006 . Physiological effects of eicosapentaenoic acid (EPA) and docosahexaenoic acid (DHA)—A review . Food Reviews International , 22 : 291 – 307 .
- Givens , D.I. and Gibbs , R.A. 2006 . Very long chain n-3 polyunsaturated fatty acids in the food chain in the UK and the potential of animal-derived foods to increase intake . Nutrition Bulletin , 31 : 104 – 110 .
- Frankel , E.N. , Satué-Gracia , T. , Meyer , A.S. and German , J.B. 2002 . Oxidative stability of fish and algae oils containing long-chain polyunsaturated fatty acids in bulk and in oil-in-water emulsions . Journal of Agricultural and Food Chemistry , 50 ( 7 ) : 2094 – 2099 .
- Kolawnoski , W. 2010 . Omega-3 LC PUFA contents and oxidative stability of encapsulated fish oil dietary supplements . International Journal of Food Properties , 13 ( 3 ) : 498 – 511 .
- Punta , C. , Rector , C.L. and Porter , N.A. 2005 . Peroxidation of polyunsaturated fatty acid methyl esters catalyzed by n-methyl benzohydroxamic acid: A new and convenient method for selective synthesis of hydroperoxides and alcohols . Chemical Research in Toxicology , 18 : 349 – 356 .
- Pierini , M. and Punta , C. 2006 . New developments in peroxidation of polyunsaturated fatty acids . Letters in Organic Chemistry , 3 : 91 – 97 .
- Ladikos , D. and Lougovois , V. 1990 . Lipid oxidation in muscle foods: a review . Food Chemistry , 33 : 295 – 314 .
- Kolanowski , W. , Jaworska , D. and Weibrodt , J. 2007 . Review importance of instrumental and sensory analysis in the assessment of oxidative deterioration of omega-3 long-chain polyunsaturated fatty acid-rich foods . Journal of the Science of Food and Agriculture , 87 : 181 – 191 .
- Bou , R. , Codony , R. , Tres , A. , Decker , E.A. and Guardiola , F. 2008 . Determination of hydroperoxides in foods and biological samples by ferrous oxidation-xylenol orange method: A review of the factors that influence the method's performance . Analytical Biochemistry , 337 : 1 – 15 .
- DeLong , J.M. , Prange , R.K. , Hodges , D.M. , Forney , C.F. , Bishop , M.C. and Quilliam , M. 2002 . Using a modified ferrous oxidation-xylenol orange (FOX) assay for detection of lipid hydroperoxides in plant tissue . Journal of Agricultural and Food Chemistry , 50 : 248 – 254 .
- Firestone , D. 1995 . “ Methods of Analysis of AOAC International ” . In AOAC International: Maryland Edited by: Cunniff , P. 17 – 18 . Oils and Fats
- Eymard , S. and Genot , C. 2003 . A modified xylenol orange method to evaluate formation of lipid hydroperoxides during storage and processing of small pelagic fish . European Journal of Lipid Science and Technology , 105 : 497 – 501 .
- Nourooz-Zadeh , J. , Tajaddini-Sarmadi , J. , Birlouez-Aragon , I. and Wolff , S.P. 1995 . Measurement of hydroperoxides in edible oils using the ferrous oxidation in xylenol orange assay . Journal of Agricultural and Food Chemistry , 43 : 17 – 21 .
- Gámez-Meza , N. , Higuera-Ciapara , I. , Calderón , de la Barca , Vázquez-Moreno , A.M. , Noriega-Rodríguez , L. and Angulo-Guerrero , J. 1999 . O. Seasonal variation in the fatty acid composition and quality of sardine oil from Sardinops sagax caeruleus of the Gulf of California . Lipids , 34 : 639 – 642 .
- Turan , H. , Kaya , Y. and Erkoyuncu , I. 2007 . Protein and lipid content and fatty acid composition of anchovy meal produced in Turkey . Turkish Journal of Veterinary and Animal Sciences , 31 ( 2 ) : 113 – 117 .
- Kartal , M. , Kurucu , S. , Aslan , S. , Özbay , Ö. , Ceyhan , T. , Sayar , E. and Cevheroğlu , Ş. 2003 . Comparison of ω-3 fatty acids by GC-MS in frequently consumed fish and fish oil preparations on the Turkish market . FABAD Journal of Pharmaceutical Sciences , 28 : 201 – 205 .
- Zlatanos , S. and Laskaridis , K. 2007 . Seasonal variation in the fatty acid composition of three Mediterranean fish-sardine (Sardina pilchardus), anchovy (Engraulis encrasicholus) and picarel (Spicara smaris) . Food Chemistry , 103 : 725 – 728 .
- Karaçalı , M. , Bulut , S. , Konuk , M. and Solak , K. 2011 . Seasonal variations in fatty acid composition of different tissues of mirror carp, Cyprinus carpio, in Orenler Dam Lake, Afyonkarahisar, Turkey . International Journal of Food Properties , 14 : 1007 – 1017 .
- Martínez Téllez , G. , Hernández Tápanez , R. and Díaz Gómez , M. 2005 . Determinación de hidroperóxidos totales en aceite de girasol ozonizado mediante el método de oxidación ferrosa en xilenol naranja . Grasas y Aceites , 56 ( 2 ) : 147 – 152 .
- Okuyama , H. , Orikasa , Y. and Nishida , T. 2008 . Significance of antioxidative functions of eicosapentaenoic and docosahexaenoic acids in marine microorganisms . Applied and Environmental Microbiology , 74 ( 3 ) : 570 – 574 .
- Harpaz , S. , Eshel , A. and Lindner , P. 1994 . Effect of 1-propanol on the activity of intestinal proteolytic enzymes of the European sea bass Dicentrarchus labrax . Journal of Agricultural and Food Chemistry , 42 : 49 – 52 .
- Takahashi , K. and Takeuchi , T. 1989 . Extraction of phospholipid-containing feed oil by propylalcohol . Nippon Suisan Gakkaishi , 55 ( 6 ) : 1059 – 1066 .
- Grau , A. , Codony , R. , Rafecas , M. , Barroeta , A.C. and Guardiola , F. 2000 . Lipid hydroperoxide determination in dark chicken meat through a ferrous oxidation-xylenol orange method . Journal of Agricultural and Food Chemistry , 48 : 4136 – 4143 .
- Talpur , M.Y. , Sherazi , S.T.H. , Mahesar , S.A. and Bhutto , A.A. 2010 . A simplified UV spectrometric method for determination of peroxide value in thermally oxidized canola oil . Talanta , 80 : 1823 – 1826 .
- Crowe , T.D. and White , P.J. 2001 . Adaptation of the AOCS Official Method for measuring hydroperoxides from small-scale oil samples . Journal of the American Oil Chemists’ Society , 78 : 1267 – 1269 .