Abstract
Noodles were produced by blending varying proportions (20, 30, 40, 50, and 60% levels) of taro flour with remaining equal proportions of rice and pigeon pea flour, which were evaluated for anti-nutritional, cooking, textural, and sensory properties and possible correlations between various noodles' properties were established using principal component analysis. Anti-nutritional evaluation of noodles revealed a decrease in phytic acid content, as the percentage of taro flour in the noodles increased. Pasting properties of the blends were also measured, which differed significantly (P < 0.05). Taro flour addition produced noodles with decreased gumminess, adhesiveness, b* value, and increased a* value as compared to the control sample (100% wheat flour). Significant correlations among various noodle properties were established using principal component analysis. Texture and color can be adopted as distinguished parameters for analyzing the noodle samples using a principal component analysis loading plot. Noodles containing 50% taro flour, with remaining equal proportions of rice and pigeon pea flour, resulted in the highest scores for color, taste, firmness, and overall acceptability.
INTRODUCTION
Taro (Colocasia esculenta) is a member of the Arum family (Aracea) and is a tropical tuber crop largely produced for its underground corms and is mainly consumed in tropical areas of the world. The different varieties of taro available in the world include Cyrtosperma chamissonis (giant swamp taro), Xanthosoma sagittifolium, Colocasia esculenta (taro), and Alocasia macrorrhiza (giant taro). It is rich in gums (mucilage) and has been reported to have 70–80% starch with small granules (diameter between 1.4–5 μm).[Citation1] Because of the small sizes of its starch granules, taro was highly digestible and was reported to be used for the preparation of various foods. Taro consumption has been affected by the presence of acridity factors, which cause sharp irritation and a burning sensation in the throat and mouth on ingestion.[Citation2] Removal of the thick layer of skin and a long period of cooking was required to remove acridity.[Citation3] Taro's digestibility, because of small starch grains, and its hypoallergenic qualities are other qualities that make it a unique product.
Rice (Oryza sativa L.), being a major cereal crop, is an important source of energy, hypoallergenic, easily digested, providing protein with higher nutritional quality, and has versatile functional properties. When rice is to be processed into various traditional products, snacks, breakfast cereals, and other cooked or extruded products, it is first milled and ground into flour and sieved into different sizes.[Citation4] The particle size distribution of rice flour is known to play an important role in its functional properties and the quality of end products.[Citation5] Rice flour has a soft taste, is colorless, has hypoallergenic properties, low levels of sodium, and an easily digestible carbohydrate, which makes it the most suitable cereal to make gluten free products.[Citation6] As consumers have become more health conscious, there has been greater emphasis on improving the nutritional profile of foods or being able to deliver other health benefits.[Citation7] Modifying the rice flour can change its functional properties in such a way that it may also be used as a substitute in other value-added products.
The pigeon pea, also known as toor dal (India), Congo Pea or Gungo Pea (in Jamaica), Gandulo (in Puerto Rico), is a perennial member of the family Fabaceae. Pigeon pea significantly contributes to meet the requirements of crude fiber, ash, fat, magnesium, manganese, and copper.[Citation8] It contains high amounts of vitamin B, carotene, ascorbic acid, high levels of protein, and the important amino acids: methionine, lysine, and tryptophan. In combination with cereal, pigeon peas make a well-balanced human food.
Noodles are one of the extruded products, which are popular due to their sensory appeal, low cost, ease of preparation, storage stability, and consumer interest in the food; they are often named after the raw material source. The utilization of different substrates for starch noodles that have been studied includes rice flour,[Citation9] legume starches,[Citation10] and tuber and tuber-legume starch blends.[Citation11] Rice, taro, and pigeon pea flour have very unique attributes. The benefits of these composite flours are largely centered on nutritional considerations.[Citation12] The method of preparation of noodles from rice, wheat flour, and their combination with other flours have been reported, but the reports on the utilization of taro flour with other flours are scarce. Therefore, the present work was undertaken to standardize the method for the preparation of noodles based on combination of rice, taro, and pigeon pea flour and to study the anti-nutritional, textural, and sensory attributes of taro, rice, and pigeon pea flour-based noodles and to establish possible correlations between various properties using PCA (principal component analysis). PCA can be used to visualize the variation in the properties of noodles. With this technique, a large number of variables are reduced to a few variables called principal components (PCs), which describe the greatest variance in the data analyzed; therefore, it is considered beneficial to identify the similarities and differences between the samples and the interrelationships among the measured properties can be judged.
MATERIALS AND METHODS
Procurement of Raw Material
Raw taro corms (commercial variety) were purchased from a local market in Sangrur, Punjab, India. The raw materials were physically examined to ensure they were disease-free and then they were stored in a cool (10°C) temperature and used within 24 h. Rice brokens were purchased from a rice sheller (located at Dhuri, Punjab, India). These rice brokens were milled in chakki to obtain rice flour. The pigeon pea flour was obtained from a mill located in Parbhani, Maharashtra, India and after this it was passed through mesh no. 72 sieve (0.26 mm). The taro flour was made from taro cormels by following the different processing operations as given in Fig. 1.
Figure 1 Flow chart for the preparation of taro flour. Data from Kaur et al.[Citation26]
![Figure 1 Flow chart for the preparation of taro flour. Data from Kaur et al.[Citation26]](/cms/asset/0a3b81df-a6c6-49c0-b2a4-bf8f16fe0905/ljfp_a_665405_o_f0001g.gif)
Preparation of blends
Blends were prepared by mixing rice, pigeon pea, and taro flour in different combinations. The different ratio of taro flour at 20, 30, 40, 50, and 60% levels with the blends consisting of equal proportions of rice flour and pigeon pea flour were taken. The appropriate amounts of rice, pigeon pea, and taro flour were weighed using an electronic balance to give the various ratios of the composite flour, which were mixed and stored in airtight containers for use.
Chemical Composition
The control sample (100% wheat flour) and blends of taro, rice, and pigeon pea flours were estimated for their moisture, ash, fat, crude fiber, and protein contents by employing the standard methods of analysis.[Citation13] The amylose content was determined as per the standard method.[Citation14]
Pasting Properties
Pasting properties of blends were studied by using a rapid Visco analyzer (RVA Starch Master 2, Model no. N13713, Newport Scientific Pvt. Ltd., Australia). Viscosity profiles of blends were recorded using blend suspensions (10% w/w; 28 g total weight). The sample was heated from 50 to 95°C at 6°C per min (after an equilibrium time of 1 min at 50°C and a holding time of 5 min at 95°C. The cooling was carried out from 95 to 50°C at 6°C per min with a holding for 2 min at 50°C. Parameters recorded were pasting temperature (PT), peak viscosity (PV), trough viscosity (minimum viscosity at 95°C) (TV), final viscosity (viscosity at 50°C) (FV), breakdown viscosity (peak viscosity–trough viscosity) (BV), and set back viscosity (final viscosity–trough viscosity) (SV).
Noodle Making
Dough formation
Dough was prepared by mixing 200 g of flour with a measured amount of distilled water (50%). Mixing of dough was carried out for 5 min. After preparation of the dough, it was kept for 10 min at room temperature (28°C).
Extrusion
Noodles were prepared by extruding the prepared dough through a hand-extruding machine (Anjali, New Delhi, India) fitted with a 1.5-mm die. Immediately after extrusion, the noodle strands were cut with a sharp knife to the appropriate length (12 cm). After cutting, the noodle strands were dried at 50°C.[Citation15]
Antinutritional Evaluation of Noodles
Determination of oxalate
The titration method[Citation16] was used to determine the oxalate content. One gram of the sample was weighed into a 100-ml conical flask where 75 ml of 3N H2SO4 was added and stirred intermittently with a magnetic stirrer for 1 h. It was then filtered using Whatman No. 1 filter paper. From the filtrate, 25 ml was taken and titrated while hot (80–90°C) against 0.1 N KMnO4 solution until a faint pink color persisted for at least 30 s.
Determination of phytic acid (or phytates)
The phytic acid (or phytate) content was measured as per the method.[Citation17,Citation18] The ground sample (2 g) was extracted with 40 ml of 0.5 M HNO3 for 2 h and then filtered. The solution (1 ml) was used for the total phosphorous determination.[Citation19] Ferric chloride was added to another 10 ml of solution and heated in boiling water for 75 min. After centrifugation at 12,000× g for 15 min, the supernatant was used to determine the soluble phosphorous. Total and soluble phosphorous levels were determined colorimetrically using 0.05 M ammonium thio-cyanate and were estimated using a phosphorous standard curve. The difference between total and soluble phosphorous was insoluble phosphorous. The phytic acid content was calculated from the insoluble phosphorous, assuming 1 mol of phytic acid contains 6 mol of insoluble phosphorous:
Insoluble phosphorous (mg/100 g) = Total phosphorous − Soluble phosphorous.
Determination of total polyphenol content
Total polyphenol content of noodles was calculated as per the standard procedure.[Citation20] First, take a suitable aliquot (10 ml) of sample and place it in a test tube. Make up the volume to 0.5 ml with distilled water and add 0.25 ml of the Folin-Ciocalteu reagent and then 1.25 ml of the sodium carbonate solution. Vertex the tubes and record absorbance at 725 nm after 40 min. Calculate the amount of total phenols as gallic acid equivalent from the calibration curve equation obtained from the standard curve. The regression equation was evaluated between different concentrations and O.D., which was found as: y = 0.008x + 0.099.
Characteristics of Color
Color measurements of the noodles were carried out by using a Hunter colorimeter (Model D 25, Hunter Associates Laboratory Inc., Reston, VA, USA) on the basis of L*, a*, and b* values. A glass cell containing the sample was placed above the light source, covered with a white plate, and L*, a*, and b* values were recorded. The instrument was calibrated against a standard red-colored reference tile (Ls = 25.54, as = 28.89, bs = 12.03). Total color difference (ΔE) was calculated by applying the equation:
Instrumental Textural Properties of Noodles Using Texture Profile Analysis (TPA)
TPA of cooked noodles was conducted as per the method.[Citation21] Noodles (10 g) were cooked in water (120 ml) for 3 min and subjected to texture analysis using a texture analyzer (Model TA-HDi, Stable Microsystems). A noodle strand (30–35 mm) laid in a straight line on a plate was compressed twice at 2.0 mm/s with a small flat-faced cylinder probe (20 mm diameter) at a compression ratio of 70% without inducing surface fractures. Hardness, gumminess, chewiness, etc. were measured from the TPA.
Cooking Characteristics of Noodles
Cooking time
Cooking time was determined as per the method.[Citation22] In this method, 5 g of the noodle samples were placed in 300 ml of boiling water in a 500-ml beaker. Noodle strands were removed at regular intervals and pressed between two glass plates. Time required for the opaque part in the strand to be gelatinized was considered as cooking time.
Cooked weight
To determine the cooked weight, the following method[Citation23] was followed. The dry noodles (25 g) were cooked in 300 ml of boiling water. Noodles were cooked till disappearance of the white core as judged by squeezing between glass plates. The beaker was covered with aluminum foil to minimize the evaporation losses. The cooked weight of the noodles was determined by weighing the wet mass after the cooked noodles were drained for 2.5 min.
Gruel solids loss
Noodles (10 g) were cooked to optimum time (defined as the time till there is disappearance of white core in the noodles; 4–5 min) in 300 mL of distilled water in a beaker, rinsed in cold water, and drained for 15 min before being weighed. Gruel solid loss was determined by drying overnight at 105°C.[Citation22]
Sensory Evaluation of Noodles
The sensory quality and overall acceptability of noodles was carried out on a 9-point hedonic rating scale where the samples were rated on the basis of the following criteria: 9—liked extremely, 8—liked very much, 7—liked moderately, 6—liked slightly, 5—neither liked nor disliked, 4—disliked slightly, 3—disliked moderately, 2—disliked very much, and 1—disliked extremely. The noodles were cooked and served to 10 semi-trained panelists formed from the Department of Food Engineering and Technology, SLIET, Longowal, Punjab, India. Panelists evaluated the samples for their color, flavor, appearance, and overall acceptability.
Statistical Analysis
Statistical analysis of the results was done using Minitab Statistical Software, version 15.0 (Minitab Inc., State College, PA, USA). Principal component analysis (PCA) was used for finding the relationships between various noodle properties. Data was further analyzed using one-way ANOVA (analysis of variance). Mean separation was carried out by Duncan's multiple range test using STATISTICA software, version 16.0.
Table 1 Proximate composition of blends consisting of taro, rice, and pigeon pea flour used in the preparation of noodles
RESULTS AND DISCUSSION
Proximate Composition
The proximate composition of blends used in noodle making varied significantly (P < 0.05) (). Results showed that carbohydrates, composed mainly of starch, were the most important chemical component in the blends, while the protein (except in pigeon pea flour) and fat were very limited. Total carbohydrate content of different blends ranged between 70.95 to 73.70%, which were found to be highest for the control sample followed by sample E containing 60% taro flour (71.92%). An increase in the levels of taro flour in the blends resulted in increased carbohydrate content. This was expected as taro was composed of mainly carbohydrate-rich materials. Amylose content has an important effect on its pasting properties due to quick retrogradation, association, and interaction to lipids and amylopectin to form a helical complex giving strong gel structures. It ranged between 3.55 to 4.87% for the different blends used in the study. Amylose content was found to be highest for sample A containing 20% taro flour (4.87%) (). An increase in the levels of taro flour in the blends resulted in a decrease in the amylose content.
Besides carbohydrate, ash represents another important group of components in blends (). Ash content of different blends ranged between 2.38 to 3.39%. It was observed that with an increase in the amount of taro flour, the ash content of the noodles increased. This entails that incorporation of taro flour in the process of noodle making could enhance the mineral intake, as ash is indicative of the amount of minerals contained in any food sample.[Citation23]
The protein content of blends varied between 12.9 to 15.25%. Increase in the levels of taro flour in the blends resulted in a decrease in the protein content progressively from 15.25 to 13.37% in the blends with 60% taro flour. As a result of the low level of proteins in the taro flour, their incorporation into wheat flour is expected to reduce the protein content of the composite and, thus, has a significant effect on the rheology of dough made from such composites.[Citation24]
The fat content of blends ranged from 1.29 to 1.83%. It was observed that with an increase in the amount of taro flour, the fat content of the blends decreased. Crude fiber content of blends ranged between 0.2 to 0.5%, whereas for the control sample, the crude fiber content comes out to be 0.9%. The mean crude fiber content of the blends was increased with an increase in amounts of taro flour. Studies have shown that crude fiber is best obtained from foods than supplements and can reduce the symptoms of chronic constipation, heart diseases associated with high cholesterol, diverticular disease, and can reduce the risk of colon cancer. Hence, taro flour-based noodles could be acceptable in places where crude fiber diets and lower fatty foods are desired.
Table 2 Variables examined with PCA ( and )
Figure 2 Principal component analysis: score plot of the first principal component (PC1) and second principal component (PC2) describing the overall variation among control sample and different blends. Sample A: 20% taro flour, 40% rice flour, 40% pigeonpea flour; Sample B: 30% taro flour, 35% rice flour, 35% pigeonpea flour; Sample C: 40% taro flour, 30% rice flour, 30% pigeonpea flour; Sample D: 50% taro flour, 25% rice flour, 25% pigeonpea flour; Sample E: 60% taro flour, 20% rice flour, 20% pigeonpea flour; WF: 100% wheat flour. (Color figure available online.)
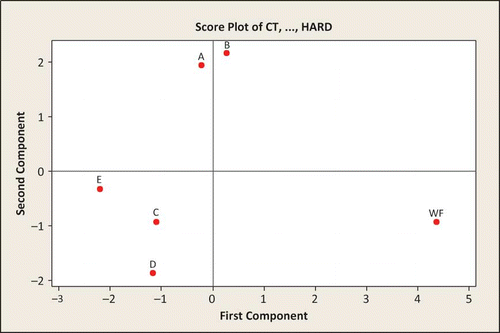
Figure 3 Principal component analysis: loading plot of the first principal component (PC1) and second principal component (PC2) describing the variation among different properties of noodles. CT: Cooking time; GL: gruel loss; CW: cooked weight; del E: total color difference; GUMM: gumminess; HARD: hardness; SPRING: springiness; RESIL: resilience. (Color figure available online.)
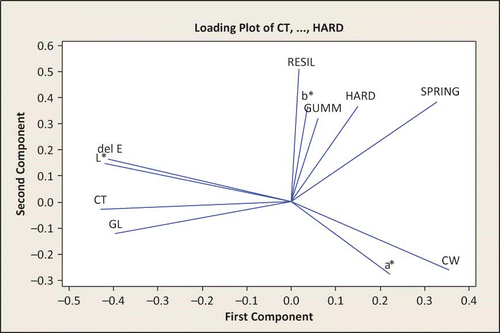
Figure 4 Principal component analysis: Loading plot of PC1 and PC2 describing the variation among the properties of noodles. A heavy solid line and a second line very close to it indicate two properties that are highly correlated. GL: Gruel loss; PT: pasting temperature; PV: peak viscosity; Cook W: cooked weight; Cook T: cooking time; Carbo: carbohydrate; Chew: chewiness; Hard: hardness; BV: breakdown viscosity; PV: peak viscosity. (Color figure available online.)
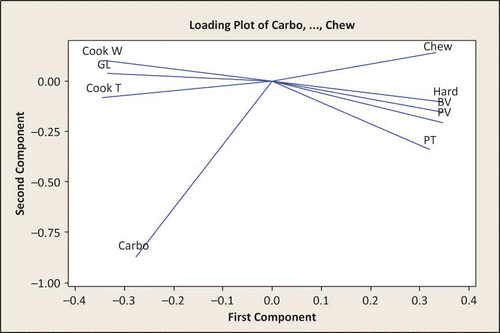
Principal Component Analysis of Noodles
PCA was used to visualize the variation in the properties among different noodle samples containing varying proportions of taro, rice, and pigeon pea flour as compared to the control sample. The variables subjected to PCA are listed in . The results of the analysis are shown in –. With this statistical method, a large number of variables are reduced to a few variables called principal components (PCs) that describe the greatest variance in the data analyzed. An overview of the similarities, differences, and the interrelationships between the measured properties was provided by score and loading plot of PCA, respectively. The distance between the locations of any two samples on the score plot is directly proportional to the degree of difference or similarity between them (). PCA showed that with an increase in the concentration of taro flour, blends move toward the left negative score of the first principal component (PC1) (). It is clearly indicated that admixtures containing 50% taro (Sample D) and 60% taro (Sample E) were clearly differentiated from the score plot. The control sample (100% WF) was located at a positive score of PC1. The score plot () reflects that the noodles (sample E) containing 60% taro flour blended with 20% rice and 20% pigeon pea flour has the highest degree of variance, and therefore, can be judged for cooking, color, and textural properties on the basis of which of its quality can be predicted. The loading plot () shows the correlation of various parameters, like L*, a*, cooked weight, etc., out of which textural and color parameters are closely correlated. Hence, texture and color can be taken as two distinguished parameters for analyzing noodle samples.
Pasting Properties
When starch-based foods are heated in an aqueous suspension, they undergo a series of changes known as gelatinization and pasting, which greatly influence the quality and aesthetic considerations in the food industry because these changes affect texture and digestibility as well as the end use of starchy foods. The pasting characteristics play an important role in the selection of a variety for use in the industry as a thickener, binder, or for any other use. The viscosity of the gel formed during and after heating is an important factor in the selection process. Pasting properties are dependent on the rigidity of starch granules, which in turn affect the granule swelling potential and the amount of amylose leaching out in the solution.[Citation25]
The results from the RVA of different blends are summarized in . PT is the temperature at which the first detectable increase in the viscosity is measured and is an index characterized by the initial change due to the swelling of starch. PT has been reported to relate to water binding capacity. PT of different blends varied from 92.8 to 94.7°C, whereas it comes out to be 90°C for the control sample. As the percentage of taro flour in the blends increased, PT decreased indicating the presence of starch that is highly resistant to swelling and rupturing. The highest value of PT was observed for the sample containing 20% taro flour indicating higher water binding capacity, higher gelatinization, and lower swelling property of starch, which may be due to a higher degree of association between starch granules.
Table 3 Pasting properties of blends consisting of taro, rice, and pigeon pea flours used in the preparation of noodles
PV is the ability of starches to swell freely before their physical breakdown. The PV for control sample was observed to be 1620 cP, whereas it varied between 2070.8 to 2200.5 cP for the different blends (). As the percentage of taro flour in the blends increased, PV decreased. The highest PV was observed for a sample containing 20% taro flour, indicating the presence of a high amount of starch content. The high PV indicated that the blend may be suitable for products requiring high gel strength and elasticity. Swelling power of blends is related to their protein and starch contents. High protein content in the flours may cause the starch granules to be embedded within a stiff protein matrix, which may limit the access of the starch to water and subsequently restrict the swelling power. Changes in viscosity during the cooking period give indications of the stability, and the changes occurring during cooling show the consistency of the product when consumed.[Citation26]
TV, being the minimum viscosity attained in the constant temperature phase of RVA profile, is influenced by the rate of amylose exudation, granule swelling, and amylose–lipid complex formation. TV of different blends ranged between 1830 to 1900 cP (). The TV was the lowest for the control sample (1069 cP). BV of blends ranged between 242 to 292 cP () with the lowest being observed for a sample containing 60% taro flour and the highest for the control sample (292 cP). As the percentage of taro flour in the blends increased, BV decreased indicating its paste stability. PV and BV are positively correlated with each other in PCA loading plot ().
FV, which indicates the ability of the material to form a viscous paste, is largely determined by the retrogradation of soluble amylose upon cooling and it is one of the most commonly used parameter to define the quality of a particular starch-based sample, as it indicates the ability of the material to form a gel after cooking and cooling as well as the resistance of the paste to shear force during stirring. SV is the recovery of the viscosity during cooling of the heated starch suspension and is a measure of the syneresis of starch upon cooling of cooked starch pastes. FV and SV of different blends showed significant variation, ranging from 1950.20 to 2699.40 cP and 750.20 to 1050.50 cP, respectively (). Final viscosity is mainly affected by amylose exudation and competition between exudated amylose and the remaining granules. Therefore, the possible reason for variation in the final viscosity after incorporation of taro flour in the blends may be the aggregation of amylose molecules. It was observed that, with the increase in the percentage of taro flour in the blends, SV decreased indicating its lower tendency to retrograde. The lower tendencies to retrograde are advantageous in food products, such as soups and sauces, which undergo loss of viscosity and precipitation,[Citation27] whereas a higher value of SV indicates the lower tendency to undergo retrogradation, thereby lowering the staling rate of the products made from the flour.
Antinutritional Evaluation of Noodles
Antinutrients and plant toxins are known to interfere with the bioavailability of some nutrients. Among various antinutrients and natural plant toxins, trypsin inhibitor (TI), phytic acid, oxalic acid, and cyanide are found in root crops. Antinutritional evaluation of different noodle samples is presented in . Phytic acid varied from 80.10 to 93.71 mg/100 g, the highest for sample A and lowest for sample E was observed. The phytic acid content in the noodles prepared from 100% taro flour was observed to be 66.51 mg/100 g. It was observed that as the percentage of taro flour in the noodles increased, there was reduction in the phytate content. This was because the level of phytate is higher in legumes.[Citation28]
Table 4 Antinutritional evaluation of noodles
The oxalate content in noodles ranged between 21.16 to 24.99 mg/100 g (); the highest for sample E and the lowest for sample A was obtained. Oxalate content in the noodles containing 100% taro flour was observed to be 26.31 mg/100 g. Too much of the soluble oxalate in the body prevents the absorption of soluble calcium ions as the oxalate binds the calcium ions to form insoluble calcium oxalate complex. On the other hand, oxalate-rich foods help to reduce blood cholesterol.[Citation29] But the risk for calcium deficiency, due to the consumption of oxalate containing plants, is very minor because human beings are able to efficiently use a very low amount of calcium in the food.[Citation30] Total polyphenol content in noodles ranged from 310.45 to 577.21 mg/100 g (). The highest total polyphenol content was observed for a sample containing 100% taro flour followed by sample E, while the lowest was observed for sample A.
Cooking Properties of Noodles
Noodle quality could be estimated from cooking attributes, such as cooking loss, gruel loss, and cooking time. The effect of taro flour addition on the cooking properties of noodles is presented in . PCA was used to correlate cooking properties, pasting, and textural parameters. PCA loading plot () provides the interrelationship between various parameters.
Table 5 Cooking properties and color characteristics of noodles
Cooking time
Time required for the opaque part in the noodle strand to be gelatinized was considered as the cooking time, which ranged between 5.17 to 6.59 min for the different noodle samples. The results showed () that cooking time increased with an increase in level of taro flour substitution. Maximum cooking time was observed for sample E (6.59 min), whereas a minimum was observed for the control sample (3.04 min). PCA plot () indicated a negative correlation of cooking time with breakdown viscosity and peak viscosity. Amylose content is believed to have a marked influence on the breakdown viscosity and setback viscosity.[Citation31]
Cooked weight
The cooked weight increase is a measure of the amount of water absorption characteristics of the product. The cooked weight increase should be 3.0 to 3.5 times the dry weight. The product should hold its shape and should not stick together during or after cooking.[Citation32] As the percentage of taro flour in blends increased, the cooked weight significantly increased. The cooked weight was maximum for noodles made from control sample (15.02 g) followed by 60% taro flour (11.1 g), TF–50% (10.7 g), TF–40% (10.3 g), TF–30% (10.1 g), and TF–20% (9.7 g) as shown in . The results on cooked weight in the present study were corroborated to those who observed that, as the percent of taro flour increased, cooked weight of noodles increased.[Citation33] The aim of using the control sample in the study was to compare the properties of taro-based noodles with the control sample. It was observed that the noodles made from 60% taro flour were sticky and prone to breakage. Cooked weight was positively correlated with cooking time and gruel loss ().
Gruel loss
Gruel solid loss is the percent of dry solids lost to the cooking water and is an indicator of a noodle's resistance to cooking. Low levels of cooking loss (<9%) are generally preferable.[Citation32] A high cooking loss is undesirable because it represents high solubility of starch, resulting in turbid cooking water, low cooking tolerance, and sticky mouth feel.[Citation33] All noodle samples containing a different level of taro flour had losses all under 2%. The highest gruel solid loss in the sample was observed with a sample containing 60% taro flour, which lost 0.75% solids (). Cooking loss could be attributed to weak protein-starch interaction and destroyed protein matrix.[Citation34] Taro flour addition in noodles would have resulted in a similar effect. Moreover, high cooking loss of noodles as compared to control may result from the presence of high water soluble protein fraction and minerals in the taro flour. Cooking loss of noodles in this study was <10%, which is within the acceptable range by Chinese and Thai standards for starch noodles.[Citation35] Among the noodles, sample E showed the highest cooked weight (11.1 g) and highest gruel loss (0.75%). PCA loading plot indicated a negative correlation of amylose content and cooking loss but positively correlated with cooking time and cooked weight (). PV and gruel loss are negatively correlated. A high peak viscosity represents low cooking loss.[Citation11]
Color Characteristics of Noodles
The color of the food products is the first attribute that affects the decision of the consumer for purchasing or consuming any food products. Noodle color and discoloration can depend on several factors, such as intrinsic flour color, flour extraction rate, flour particle size, sprout damage, protein content, and enzyme activity. The results depicted in indicated that the addition of taro flour significantly affected the color of noodles. The L* values for different noodle samples varied from 65.74 to 85.88. A significant increase in L* value was observed with the addition of taro flour. The highest values for sample E (85.88) and the lowest of the control sample (65.74) were observed. These results corroborated well with the sensory analysis data. Hunter a* and b* values for different noodle samples ranged between 0.14 to 2.43 and 13.02 to 16.68, respectively (). The variation in b* values among different noodle samples is due to the difference in their protein and carbohydrate content due to their role in development of non-enzymatic browning. ΔE value decreased with an increase in level of taro flour addition. The lowest value (36.49) was observed for the control sample. Similar results were observed by Donnelly,[Citation32] who blended taro flour to wheat flour for the production of noodles.
Table 6 Instrumental textural parameters of noodles
Instrumental Textural Parameters of Cooked Noodles
The main criterion for assessing the overall quality of cooked noodles is based on the evaluation of texture. The texture of a cooked noodle is perceived as the resistance of the noodle to chewing and the mouth feel of its surface.[Citation36] Several textural parameters of cooked noodles from a texture profile analyzer (TPA) are summarized in . The hardness, which is defined as the force necessary to attain a given deformation, was obtained from peak force value corresponding to the first two successive compressions. Sample A showed a higher value of hardness. This result was similar to starch noodles prepared from bean starches, which showed a higher value for hardness than those prepared from potato starches, although amylose content has been considered to be an important factor affecting noodle hardness. The results showed that, as the amount of taro flour increased in noodles, the hardness of noodles decreased. Gumminess in noodles ranged between 1.850–3.222 g (). Gumminess decreased with the increase in the percentage of taro flour in the noodles. Hardness and gumminess are positively correlated with each other in PCA loading plot (). Adhesiveness of noodles varied from 1.50–2.79 g × mm. These results corroborated well with the sensory analysis (), where it was observed that stickiness and adhesiveness of noodles decreased with the increase in the percentage of taro flour. Springiness of noodles varied from 0.017 to 0.287 mm (). The results of springiness (which indicates the percentage recovery of noodles), and resilience (which express the ability or speed of material to return to its original shape after a stress) indicated that when the substitution level of taro flour increased, the noodles required more time to recover its shape. Resilience of noodles varied from 0.041 and 0.151 (). The highest value of resilience and springiness was observed for the control sample. Resilience and springiness are positively correlated with each other (). Chewiness is the most indicative characteristic of noodles. Chewiness of noodles varied from 0.031 to 0.805 g × mm. The results showed that as the amount of taro flour increased in noodles, the chewiness of noodles decreased.
Table 7 Sensory parameters of noodles
Sensory Evaluation of Noodles
shows the result of the sensory evaluation of the different noodle samples. The control sample was rated best (8.3) followed by sample E (7.5), while sample A had the least color rating (5.2). The control sample had the best taste rating (8.2), followed by sample D (8.0), while sample E received the least rating (5.1). The firmness rating ranged between 5.2 to 8.1, the highest being the control sample, followed by sample A (7.6). The effect of taro flour was also found to be significant on other attributes like stickiness. The results showed that as the level of taro flour increased (up to 50% substitution) the sensory scores for sensory color, taste, firmness, and overall acceptability increased. Thus, the results indicated that the panelist accepted the noodles prepared from a different level of taro flour with the highest acceptability at 50% taro flour level followed by 20% taro flour level. Control noodles were highly acceptable followed by noodles prepared from 50% taro flour substitution. Texture of cooked noodles is an important factor that influences the overall quality and acceptability of the noodles.[Citation37] The texture of cooked noodles should be firm, elastic, or springy with a smooth surface.
CONCLUSIONS
The noodles produced from the increased proportion of taro flour in different proportions revealed decreased gumminess, adhesiveness, b* value, phytic acid content and increased a* value and oxalate content as compared to the control. All the viscosity parameters decreased with an increase in proportions of taro flour addition to rice and pigeon pea flour in RVA analysis. Cooking time and cooked weight increased with an increase in level of taro flour substitution; however, cooking losses of all the noodle samples were below 6%. The PCA score reflects the noodles (Samples D and E) containing 50 and 60% taro flour, respectively, have the highest degree of variance, and thus, can be judged for cooking, color, and textural properties on the basis of which its quality can be predicted. The sensory panelists rated the noodles prepared from 50% taro flour addition to rice and pigeon pea flour with acceptability scores close to the control sample.
REFERENCES
- Sugimoto , Y. , Nishihara , K. and Fuwa , H. 1986 . Some properties of taro (Ishikawa-wase and Takenokoimo) and yam (Iseimo and Nagaimo) starch . Journal of the Japanese Society of Starch Science , 34 : 1
- Akpan , E. and Umoh , I.B. 2004 . Effect of heat and tetracycline treatments on the food quality and acridity factors in cocoyam [Xanthosoma sagittifolium (L.) . Schott]. Pakistan Journal of Nutrition , 3 : 240 – 243 .
- Crabtress , J. and Baldry , J. 1982 . Technical note: The use of taro products in bread making . Journal of Food Technology , 17 : 771 – 777 .
- Juliano , B.O. and Hicks , P.A. 1996 . Rice functional properties and rice food products . Food Research International , 12 : 71 – 103 .
- Chen , J.J. , Lu , S. and Lii , C.Y. 1999 . Effect on the physicochemical characteristics by milling methods of waxy rice in Taiwan . Cereal Chemistry , 76 : 796 – 799 .
- Lopez , A.C.B. , Pereira , A.J.G. and Junqueira , R.G. 2004 . Flour mixture of rice flour, corn and cassava starch in the production of gluten-free white bread . Brazilian Archives of Biology and Technology , 47 : 63 – 70 .
- Pordesino , L.O. , Onwulata , C.I. and Carvalho , C.W.P. 2009 . Food powder delivery through a feeder system: Effect of physicochemical properties . International Journal of Food Properties , 12 : 570
- Faris , D.G. and Singh , U. 1990 . “ Pigeon pea: Nutrition and products ” . In The Pigeon pea , Edited by: Nene , Y.L. , Hall , S.D. and Sheila , V.K. 401 – 434 . UK : CAB International: Wallingford, Oxon .
- Juliano , B.O. 1993 . “ Major processed rice products ” . In Rice in Human Nutrition , 101 – 123 . Rome : FAO Food and Nutrition Series No. 26. Food and Agriculture Organization (FAO) .
- Xu, X.; Hu, G.; Zhang. In: Proceedings of the International Symposium on New Approaches in the Production of Foodstuffs and Intermediate Products from Cereal Grains and Oilseeds. Chinese Cereal and Oil Association: Beijing, 1994, 808–811.
- Collado , L.S. and Corke , H. 1997 . Properties of starch noodles as affected by sweet potato genotype . Cereal Chemistry , 74 : 182 – 187 .
- Fenna , D. , Lukow , O.M. , Humphreys , G. , Fields , P.G. and Boye , J.I. 2010 . Wheat-legume composite flours quality . International Journal of Food Properties , 13 : 381 – 393 .
- AOAC . 2000 . Official Methods of Analysis of the Association of the Analytical Chemists , 17th , Gaithersburg, MD : AOAC .
- Scott , G. and Gilead , D. 1998 . Degradable Polymers; , London , UK : Chapman & Hall .
- Surojanametakul , V. , Tungtakul , P. , Varanyanond , W. and Supasri , R. 2002 . Effect of partial replacement of rice flour with various starches on the physicochemical and sensory properties of “Sen Lek” noodle . Kasetsart Journal (Natural Science) , 36 : 55 – 62 .
- Day , R.A. Jr. and Underwood , A.L. 1986 . Quantitative Analysis , 5th , Prentice-Hall Publication: Upper Saddle River, NJ .
- Griffiths , D.W. 1982 . The phytate content and iron-binding capacity of various field bean (Vicia faba) preparations and extracts . Journal of the Science of Food and Agriculture , 33 : 347 – 351 .
- Wang , C.R. , Chang , K.C. and Grafton , K. 1988 . Canning quality of pinto and navy beans . Journal of Food Science , 53 : 772
- Thompson , D.B. and Erdman , J.W. 1982 . Structural model for ferric phytate: Implications for phytic acid analysis . Cereal Chemistry , 59 : 525 – 528 .
- Ayurvedic Pharmacopoeia of India . 2007 . Book of Standards for Compound Formulations under the Drugs and Cosmetics Act, Part II, Vol. I., Govt. of India, Ministry of Health and Family Welfare , New Delhi , India : Department of Ayurveda, Yoga and Naturopathy, Unani, Siddha (Ayush) .
- Lee , S.Y. , Woo , K.S. , Lim , J.K. , Kim , H.L. and Lim , S.T. 2005 . Effect of processing variables on texture of sweet potato starch noodles prepared in a non freezing process . Cereal Chemistry , 82 ( 4 ) : 475 – 478 .
- AACC . Approved Methods of the AACC , 9th , 1995 St. Paul , MN : AACC .
- Olaoye , O.A. , Onilude , A.A. and Oladoye , C.O. 2007 . “ Breadfruit flour in biscuit making: Effects on product quality ” . In African Journal of Food Science 20 – 23. .
- Njintang , Y.N. , Mbofung , C.M. , Balaam , F. , Kitissou , P. and Scher , J. 2007 . Effect of taro (Colocasia esculenta) flour addition on the functional and alveographic properties of wheat flour and dough . Journal of the Science of Food and Agriculture , 88 ( 2 ) : 273 – 279 .
- Morris , V.J. 1990 . Starch gelation and retrogradation . Trends in Food Science and Technology , 1 : 2 – 6 .
- Kaur , M. , Kaushal , P. and Sandhu , K.S. 2010 . Studies on physicochemical and pasting properties of taro (Colocasia esculenta L.) flour in comparison with a cereal, tuber and legume flour . Journal of Food Science and Technology , doi: 10.1007/s13197-010-0227-6.
- Adebowale , K.O. and Lawal , O.S. 2003 . Microstructure, physicochemical properties and retrogradation behavior of Macuna bean (Macuna pruriens) starch on heat moisture treatments . Food Hydrocolloid , 17 : 265 – 272 .
- Eka , O.U. and Osagie , A.U. 1998 . Nutritional Quality of Plant Foods; , 20 – 25 . Benin City , Nigeria : Department of Biochemistry, University of Benin .
- Savage , M. 2000 . Effect of cooking on the soluble and insoluble oxalate content of some New Zealand foods . Journal of Food Composition and Analysis , 13 ( 3 ) : 201 – 206 .
- Liener , I.E. 1989 . “ Oxalate ” . In Toxic Constituents of Plants Foodstuff , Ed , Edited by: Liener , I.E. New York : Academy Press .
- Lee , M.H. , Hettiarachchy , N.S. , McNew , R.W. and Gnanasambandam , R. 1995 . Physicochemical properties of calcium-fortified rice . Cereal Chemistry , 72 : 352 – 355 .
- Donnelly , B.J. 1986 . A Manual on Objective Tests for Spaghetti Products , Fargo , N.D. : N. Dakota State University .
- Rosarlo , M.D. , Vinas , A. and Lorenz , K. 1999 . Pasta products containing taro (Colocasia esculenta) and chaya (Cnidoscolus Chavamansa) . Journal of Food Processing and Preservation , 23 ( 1 ) : 1 – 20 .
- Izydorczyk , M.S. , Lagasse , S.L. , Hatcher , D.W. , Dexter , J.E. and Rossnagel , B.G. 2004 . The enrichment of Asian noodles with fiber-rich fractions derived from roller milling of hull-less barley . Journal of the Science of Food and Agriculture , 85 : 2094 – 2104 .
- Sisawad , S. and Chatket , I. 1987 . “ Transparent noodles from other bean starches ” . In Trends in Food Biotechnology Edited by: Ghee , A.H. , Hen , N.B. and Kong , L.K. Singapore
- Oh , N.H. , Seib , P.A. , Deyoe , C.W. , Ward , A.B. and Noodles , I. 1983 . Measuring the textural characteristics of cooked noodles . Cereal Chemistry , 60 : 433 – 438 .
- Miskelly , D.M. and Moss , H.J. 1985 . Flour quality requirements for Chinese noodle manufacture . Journal of Cereal Science , 3 : 379 – 387 .