Abstract
In this work, astaxanthin nanodispersions were prepared using selected three component stabilizer system through a solvent-diffusion technique, with the particle size of 98.3 nm. The stability of produced nanodispersions against pH, salts, and heating were then evaluated. The produced nanodispersions exhibited good physical stability under wide ranges of pH (except around isoelectric point), sodium ion concentrations, and relatively high-temperature treatments (up to 60°C). However, formation of large particles was observed in either presence of calcium ions or higher thermal treatments (more than 60°C).
INTRODUCTION
Carotenoids are natural pigments with essential biological effects including antioxidant activities.[Citation1] Astaxanthin is a carotenoid compound that is found in aquatic animals, such as shrimp, crabs, and salmon. It acts as both radical scavenger and singlet oxygen quencher due to its conjugated double bonds and both hydroxyl and ketonic end groups on each ionone ring.[Citation2] Recently, astaxanthin has gained popularity as a nutraceutical and a medicinal ingredient for the prevention and treatment of various diseases, including cancer, age-related macular degeneration, inflammation, Helicobacter pylori infections, and cardiovascular oxidative stress, and for the general enhancement of immune responses.[Citation2 − Citation5] However, as with other carotenoids, its limited water solubility/dispersibility has hampered its usefulness.[Citation6] Incorporation of these functional lipid compounds into emulsions or nanosized carriers such as nanoemulsions and nanodispersions are some of the structure modification based strategies which recently have been performed to increase their water-solubilities and consequently their bioavailabilities, according to in vivo dissolution models.[Citation6 − Citation10] Emulsions or dispersions are produced by homogenizing two immiscible phases together in the presence of stabiliser molecules.[Citation11] During homogenization, stabilizers or emulsifiers adsorb to the surfaces of freshly created droplets and reduce the interfacial tension, which facilitates further droplet disruption.[Citation12,Citation13] In addition, the stabilizers form a protective layer around the droplets, inhibiting droplet aggregation by generating repulsive interactions.[Citation14 − Citation15] The most common stabilizers used in the food industry are amphiphilic proteins, polysaccharides, and small-molecule emulsifiers, individual or in different combinations.[Citation11,Citation16] Because single stabilizer molecules exhibit different limitations in functionality, developing multi-component stabilizer systems can be an attractive means for improving a particular emulsion's physicochemical and stability characteristics, depending on its specific composition, formulation and processing, and storage methods.[Citation17 − Citation19] Therefore, in the previous research (unpublished data), a three-component stabilizer mixture composed of a small molecular emulsifier (Polysorbate 20, PS20), a protein (sodium caseinate, SC), and a polysaccharide (gum Arabic, GA) was developed to prepare the astaxanthin nanodispersions with the best physicochemical and storage stability characteristics.
During the production, storage, and utilization of nanodispersions, either stabilizer and emulsifier or functional lipid bioactive molecules undergo pressure and thermal processing;[Citation11,Citation20,Citation21] in addition, they encounter a wide range of salt types at varying concentrations, different pH levels and other environmental stresses, altering their structure, interfacial coverage, surface active functionality, and consequently the stability of the resultant nanodispersions.[Citation13,Citation20] Therefore, there is an increasing emphasis on developing a more fundamental understanding of the influence of solution conditions and environmental stresses on the functionality of stabilizer system and as a result on stability of nanodispersion system.[Citation13,Citation16,Citation22] In present study the astaxanthin nanodispersions were prepared through solvent diffusion technique and then the performance of selected three component stabilizer system in stabilizing the astaxanthin particles against heating, pH, and ionic strength were evaluated.
MATERIALS AND METHODS
Materials
Astaxanthin (>90%) was purchased from Kailu Ever Brilliance Biotechnology Co., Ltd. (Beijing, China). Polyoxyethylene sorbitan monolaurate (Polysorbate 20, PS20), phosphate buffer (pH 7), SC, sodium azide, monobasic potassium phosphate, sodium chloride, calcium chloride, analytical grade dichloromethane, and acetone were purchased by Fisher Scientific (Leicestershire, UK). Gum Arabic was donated by Merck Co. (Darmstadt, Germany). Citric acid and sodium phosphate were purchased from Sigma (MO, USA).
Preparation of Astaxanthin Nanodispersions
0.08% w/w astaxanthin was dissolved in mixture of 38% w/w dichloromethane and 62% w/w acetone to produce the organic phase.[Citation10] Aqueous phase was composed of dissolved 2.5% w/w stabilizer mixture (29% w/w PS20, 65% w/w SC, and 6% w/w GA), 0.02% w/w sodium azide in 0.05 M phosphate buffer with pH = 7.0. This aqueous solution was magnetically stirred for overnight to get fully hydrated and then was centrifuged at 3000 rpm for 5 min using a KOBOTA 2010 (Tokyo, Japan) centrifuge at room temperature to remove un-dissolved particles. While mixing the aqueous phase using a high-shear homogenizer (Silverson L4R, Buckinghamshire, UK) for 1 min at 5000 rpm, the organic phase was gradually dispersed into the water phase to provide an initial coarse emulsion, with the particle size of 934.7 ± 25.9 nm and astaxanthin content of 878 ± 28 mg/L. Fine emulsification (185.6 ± 13.4 nm) was achieved by homogenizing the coarse emulsion using a high-shear homogenizer for 5 min at 5000 rpm and then passing the emulsion three times through a high-pressure homogenizer with the pressure of 30 MPa (APV, Crawley, UK). Approximately 7.2 ± 1.6% w/w of astaxanthin loss was recorded for produced nanodispersions at the end of this preparation process. The ratio of organic phase to aqueous phase was set at 11.5% (w/w). The organic solvent was then removed from the fine emulsion using a rotary evaporator (Eyela NE-1001, Tokya Rikakikai Co. Ltd., Tokyo, Japan) under low pressure (0.15 atm) at 47°C and 100 rpm.[Citation9, Citation10] The astaxanthin content of nanodispersions after the removal of organic phase (fresh sample) was 779 ± 9 mg/L. The DCM and ACT residuals of 0.008 ± 0.004 and 0.017 ± 0.005 ppm, respectively, were recorded. These levels of residual solvents are within the acceptable level of International Conference of Hamonization (ICH) and thus showed the suitability of the produced nanodispersions for use in different food and pharmaceutical formulations.
Effects of pH
Fresh astaxanthin nanodispersion (1 mL) that was prepared with a plain aqueous phase (deionised distilled water without buffer and sodium azide) was added separately to various citrate-phosphate buffer solutions (4 mL each) with pH values ranging from 2 to 10. The pH of the mixture was adjusted using either 0.2 N sodium hydroxide or 0.2 N hydrochloric acid, if necessary. The samples were stored at room temperature for 24 h prior to analysis.[Citation16]
Effects of Na+ and Ca2+
The effect of Na+ and Ca2+ ions on the stability of the nanodispersions was studied by adding NaCl or CaCl2 solution to a 5 mL sample (freshly prepared astaxanthin nanodispersions). The salt concentration in the sample ranged from 0–200 mmol/L. The nanodispersion samples were then stored at room temperature for 24 h prior to analysis.[Citation16]
Effects of Thermal Treatments
Different sets of astaxanthin nanodispersions were placed in 50, 60, 70, 80, 90, 100, and 120°C water baths to investigate the effect of applied temperatures on mean particle sizes. The samples were removed from the water baths at 1 h intervals, cooled to room temperature by placing under running tap water, and then analysed for particle size on the following day.[Citation16]
Particle Size and PDI Measurement
Mean particle size and polydispersity index (PDI) of astaxanthin nanodispersions were evaluated by using a dynamic light-scattering particle size analyzer (Malvern series ZEN 1600, Malvern Instruments Ltd., Worcester, UK). The experiments were performed with sample preparations diluted (1:10) with deionized water to avoid multiple scattering effects in the measurements. The PDI was calculated as the best fit between the practical scattered pattern and the one predicted by the light-scattering theory.[Citation23] It is a measure of the width of the distribution ranging from 0 (monodispersion) to 1 (broad distribution).[Citation24] The absorbance of the nanodispersion particles was set at 0.3, and the temperature was 25°C. The measurement was reported as an average of two individual injections, with three readings made per injection for each sample.[Citation9]
Zeta-Potential Measurement
The zeta-potential values of the astaxanthin nanodispersions were measured using a Zetasizer Nano ZS (Malvern Instruments Ltd., Worcestershire, UK). The distribution of the electrophoretic mobility of particles is measured based on laser doppler velocity technique. The Smoluchowski equation was employed to calculate the zeta-potential values from the measured velocity.[Citation25] Measurements were carried out at pH 7.
Statistical Analysis
Statistical analysis of the experimental data was performed by a one-way analysis of variance procedure. All analyses were performed using the Minitab v. 14 Statistical Package (Minitab Inc., PA, USA) D. The signicance of differences (p < 0.05) between means was evaluated by Tukey's multiple-range tests. All the reported values were the means of at least four measurements from two experimental replications.
RESULTS AND DISCUSSION
Astaxanthin nanodispersions were produced using a bottom-up technique, known as solvent diffusion method. In this process, the water-saturated solvent phase containing astaxanthin and the solvent-saturated aqueous phase containing stabilizer mixture, which are in thermodynamic equilibrium, are emulsified using high several emulsification procedures.[Citation12,Citation26] The diffusion equilibrium is disturbed by removing the solvent, which causes the solubility limit for astaxanthin until nanoparticle formation starts.[Citation12] The mean particle size and PDI of freshly produced plain astaxanthin nanoparticles were 98.3 and 0.348 nm, respectively. The particle size distribution of nanodispersions was shown in .
Figure 1 Particle size distribution of astaxanthin nanodispersions prepared with selected three component stabilizer system. (Color figure available online.)
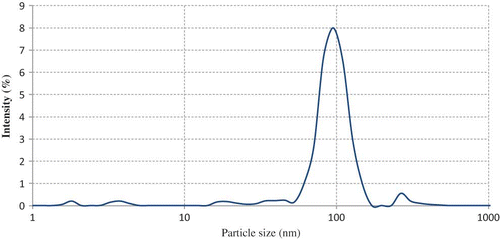
Effects of pH
The particles have a propensity to aggregate due to attractive interfacial forces, but aggregation is simultaneously resisted by repulsive like-charge interactions.[Citation11,Citation12,Citation22] The net charge of the adsorbed interfacial layer, and therefore, the magnitude of particle-particle repulsion, is thus highly dependent on pH.[Citation11] The mean particle size, PDI and zeta-potential of the prepared astaxanthin nanodispersions at different pH values are shown in .
Table 1 Mean particle size, PDI, and zeta-potential of astaxanthin nanodispersions at different pH values
The astaxanthin nanodispersion had a smaller mean particle size at neutral and alkaline pH than at acidic pH. Because the particles carry zero net surface charge when the pH equals the isoelectric point of the adsorbed stabilizer, the largest particle size was observed at pH 4–5, which was roughly the isoelectric point of SC (which composed the majority of the stabilizer mixture of produced nanodispersions).[Citation11,Citation16] The isoelectric pH of GA was approximately two, resulting in a large mean particle size for nanodispersions produced at this pH.[Citation27] The small mean particle size observed at pH values between the isoelectric points of GA and SC can be related to attractive interactions between these stabilizers.[Citation27,Citation28] Electrostatic interactions between opposite charges on GA and SC molecules lead to formation of inter-polymer complexes, increasing surface activity compared to either stabilizer alone.[Citation29] At a lower pH, the particles generally had a relatively high positive surface charge because the pH was below the isoelectric point of SC.[Citation15,Citation16] The amino groups of SC (or GA) were positively charged, whereas the carboxyl groups were neutral. Increasing the pH decreases the magnitude of positive charge on SC and GA as some amino and carboxyl groups became deprotonated.[Citation16] At alkaline pH, the magnitude of the surface charge did not change significantly (p > 0.05), suggesting that protein conformational changes due to burial of uncharged ionisable groups did not considerably affect the astaxanthin nanodispersions.[Citation16,Citation20] The dependence of the interaction potential between two astaxanthin nanoparticles on the inter-particle separation can be modelled by summing the van der Waals attraction and electrostatic repulsion potentials.[Citation11,Citation16] When the particles have a high absolute zeta-potential value, the maximum interaction potential (energy barrier), which inhibits particle aggregation, increases. Correspondingly, as the absolute zeta-potential decreases, the height of the energy barrier decreases, until it is too low to prevent the droplets from aggregating.[Citation30]
Charoen et al.[Citation28] reported a good pH-stability for GA-stabilized emulsions due to a coating of lipid droplets by a relatively thick layer of hydrophilic polysaccharide molecules and a prevalent steric repulsive stabilization, rather than an electrostatic repulsion.[Citation27] However, because the interfacial layers formed by globular proteins tend to be relatively thin, protein emulsifiers tend to stabilize emulsions mainly by electrostatic rather than steric repulsion.[Citation11,Citation22] Consequently, the addition of GA to protein-based stabilizing mixtures could improve the pH stability of astaxanthin nanodispersions near the isoelectric point of the adsorbed protein.
The presence of PS20 in the stabilizing system also altered the pH dependence of certain nanodispersion characteristics. Addition of PS20 in stabilizing mixture caused a decrease in the maximum amount of droplet flocculation near the isoelectric pH and a narrowing of the unstable pH range.[Citation31] Different physical phenomena can be considered responsible for this observation; the emulsifier could displace fully or partially the protein from the droplet interface, or the emulsifier could form a complex with the protein.[Citation20,Citation32] Either of these phenomena could change the colloidal interactions between the droplets in a manner that could inhibit droplet flocculation and consequently reduce the mean particle size in the nanodispersions.[Citation20] The displacement of some of the protein from a droplet interface by PS20 would also help inhibit aggregation near the SC and/or GA isoelectric points because PS20 acts primarily via steric repulsion, which is relatively insensitive to pH.[Citation20,Citation33 − Citation35]
Effect of Na+ and Ca2+
depicts the changes in the mean particle size in the astaxanthin nanodispersions as functions of the NaCl and CaCl2 concentrations. Different ions have different effects on dispersion stability, depending on their concentrations and valences.[Citation36] As reported by Chu et al.,[Citation16] in protein-stabilized carotenoid nanodispersions, the mean particle size increased with increasing Na+ and Ca2+ concentrations. Mineral ions increase the ionic strength of the aqueous phase, leading to a reduction in electrostatic repulsion between particles, promoting aggregation.[Citation11,Citation16,Citation36] Changes in ionic strength and specific binding of ions may also alter the conformation of the adsorbed protein, modifying the steric interactions between nanoparticle surface layers.[Citation11] Chu et al.[Citation16] have also concluded that SC-stabilized nanodispersions were moderately stable against NaCl at low concentrations (<100 mmol/L), but the mean particle size increased considerably at high NaCl concentration. They also found that presence of CaCl2 in any concentration in a nanodispersion system enlarged the mean particle size of their studied system.
Figure 2 Mean particle size in the optimum astaxanthin nanodispersions in the presence of the indicated concentrations of Na+ and Ca2+. Values are means ± standard deviations (n = 6). a, α–ϵ Different letters show statistically significant differences between values of particle size (p < 0.05). (Color figure available online.)
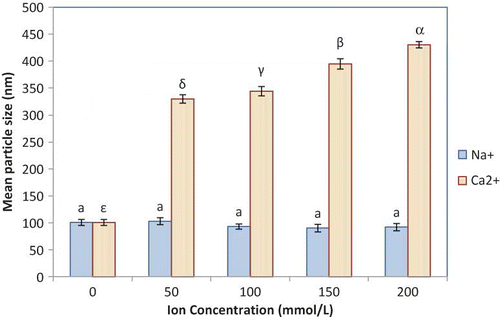
In astaxanthin nanodispersions, when NaCl salts were added, the presence of GA and PS20 could diminish the particle aggregation due to occurred steric stabilization.[Citation37,Citation38] However, as SC is mainly composed of calcium-sensitive proteins, the produced nanodispersions were easily flocculated by calcium ions. The calcium ions could destabilize the nanodispersion systems by decreasing the electrostatic repulsion due to a reduction of the net negative charge of the adsorbed protein and by the decrement of the steric repulsion due to a flattening of the adsorbed protein layer.[Citation16,Citation36] Furthermore, the divalent calcium ion may bridge protein molecules on different droplets, leading to further destabilization of the nanodispersion. Flocculation due to bridging by calcium–casein complexes or aggregates could also further destabilise the nanodispersions in the presence of calcium ions.[Citation16,Citation28,Citation39 − Citation41] The addition of cations to emulsions or dispersions can alter the isoelectric point of the system, shifting it to higher pH in a concentration-dependent manner.[Citation30] Therefore, since the NaCl did not alter the mean particle size of the nanodispersions in the present study, it seems that its addition in acidic food systems can increase the isoelectric pH of system and consequently, it can decrease the aggregation and growth of astaxanthin nanoparticles in these food systems.
Effect of Heat Treatment
Nanodispersion-containing food products may experience thermal extremes during manufacture or end use, such as during sterilization, pasteurization, or cooking. Therefore, it is essential to characterise the influence of heat treatment on astaxanthin nanodispersions. illustrates the change in mean particle size with time for the nanodispersions at the various temperatures. SC is well known for its thermal stability due to its intrinsically disordered structure.[Citation42] However, growth in particle size was observed at temperatures higher than 60°C, further particle growth at higher temperatures. The observed aggregation at high temperatures may result from the temperature-induced conformational change for the adsorbed SC molecules and subsequently expose the non-polar groups of SC molecules to the surrounding aqueous phase, increasing the surface hydrophobicity of the droplets and promoting aggregation through the hydrophobic effect.[Citation28] Bridging flocculation may also occur in the studied system by adsorbing the unfolded SC to the surfaces of partially covered oppositely charged dispersed.[Citation43] Furthermore, dephosphorylation of serine phosphate groups in the caseinate, which occurred during lengthy heating conditions, can reduce the negative charge on the caseinate and promote the casein–casein interactions.[Citation16,Citation44,Citation45]
Figure 3 Mean particle size of optimum nanodispersions during thermal treatment at (♦) 50°C; (▪) 60°C; (▴) 70°C; (×) 80°C; (Ӿ) 90°C; (•) 100°C; and (+) 120°C. (Color figure available online.)
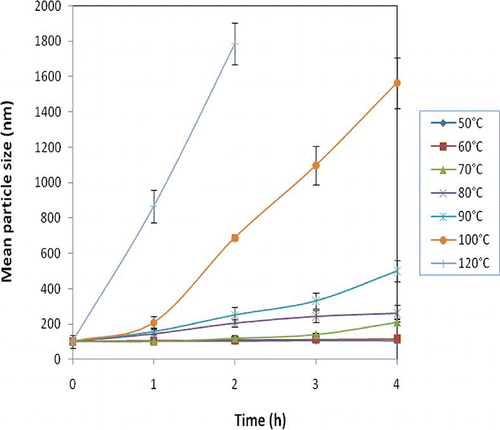
The rate of aggregation for the heated emulsions or dispersions is expected to depend on the initial particle size or on the relative partitioning of protein between the surface and the aqueous phase.[Citation46] It was reported that decreasing the total emulsion protein content by partial replacement of protein with other surface active molecules, increased their heat stability.[Citation47] Consequently, the produced astaxanthin nanodispersions showed higher thermal stability compared to nanodispersions stabilised with SC alone, as polysaccharide emulsifiers do not unfold to expose non-polar groups at high temperatures.[Citation45] Furthermore, in addition to protein, the presence of small molecular emulsifiers and/or polysaccharides in the stabilizing mixture could keep the surface active functionality of stabilizer despite the unfolding (or decrease) in the amphiphilic structure of the protein; in addition, their existence could afford for additional steric repulsion at interface of nanoparticles. Notably, the concentration of non-adsorbed protein should be as low as possible to minimise bridging across the adsorbed layers of the particles, leading to an increased aggregation rate.[Citation44,Citation45,Citation47] Therefore, the heat stability of dispersion/emulsion systems can be improved considerably by using different combinations of small-molecule emulsifiers, proteins and polysaccharides in stabilizer mixtures.[Citation20]
CONCLUSIONS
The aggregation of astaxanthin nanoparticles has a considerable effect on the appearance, shelf life, and texture of dispersions. The production of high-quality formulated food dispersions requires a good understanding of the factors that promote or inhibit aggregation of the dispersed particles and consequently affect their physical stability. The results of present study showed that unlike the most protein-stabilized nanodispersions, the produced astaxanthin nanodispersions using a three component stabilizer system (optimum combination of a small molecular emulsifier, a protein, and a polysaccharide) exhibited good physical stability under wide ranges of pH, ionic strength against Na+ ions, and relatively high-temperature treatments (up to 60°C). However, more modifications on formulation parameters of nanodispersions are needed to overcome the observed instability problems in the cases of presence of Ca2+ ions and intensive thermal treatments.
ACKNOWLEDGEMENTS
Financial support of this work by the Ministry of Science, Technology and Innovation of Malaysia through Science Fund (05-01-04-SF0384) and the Ministry of Higher Education through Fundamental Research Grant Scheme (02-11-08-0619FR) is gratefully acknowledged.
REFERENCES
- Martini , S. , D'Addario , C , Bonechi , C , Leone , G , Tognazzi , A , Consumi , M , Magnani , A and Rossi , C . 2010 . Increasing photostability and water-solubility of carotenoids: Synthesis and characterization of (beta)-carotene-humic acid complexes . Journal of Photochemistry and Photobiology B: Biology , 101 ( 3 ) : 355 – 361 .
- Guerin , M. , Huntley , M.E. and Olaizola , M. 2003 . Haematococcus astaxanthin: Applications for human health and nutrition . Trends in Biotechnology , 21 ( 5 ) : 210 – 216 .
- Chew , B.P. , Park , J.S. , Wong , M.W. and Wong , T.S. 1999 . A comparison of the anticancer activities of dietary β-carotene, canthaxanthin and astaxanthin in mice in vivo . Anticancer Research , 19 ( 3A ) : 1849 – 1853 .
- Fassett , R.G. and Coombes , J.S. 2009 . Astaxanthin, oxidative stress, inflammation, and cardiovascular disease . Future Cardiology , 5 ( 4 ) : 333 – 342 .
- Higuera-Ciapara , I. , Felix-Valenzuela , L. and Goycoolea , F.M. 2006 . Astaxanthin: A review of its chemistry and applications . Critical Reviews in Food Science and Nutrition , 46 ( 2 ) : 185 – 196 .
- Anarjan , N. , Mirhosseini , H. , Baharin , B.S. and Tan , C.P. 2010 . Effect of processing conditions on physicochemical properties of astaxanthin nanodispersions . Food Chemistry , 123 ( 2 ) : 477 – 483 .
- Ribeiro , H.S. , Guerrero , J.M.M. , Briviba , K. , Rechkemmer , G. , Schuchmann , H.P. and Schubert , H. 2006 . Cellular uptake of carotenoid-loaded oil-in-water emulsions in colon carcinoma cells in vitro . Journal of Agricultural and Food Chemistry , 54 ( 25 ) : 9366 – 9369 .
- Ribeiro , H.S. , Rico , L.G. , Badolato , G.G. and Schubert , H. 2005 . Production of O/W emulsions containing astaxanthin by repeated premix membrane emulsification . Journal of Food Science , 70 ( 2 ) : E117 – E123 .
- Anarjan , N. , Mirhosseini , H. , Baharin , B.S. and Tan , C.P. 2011 . Effect of processing conditions on physicochemical properties of sodium caseinate-stabilized astaxanthin nanodispersions . LWT-Food Science and Technology , 44 ( 7 ) : 1658 – 1665 .
- Anarjan , N. , Tan , C.P. , Ling , T.C. , Lye , K.L. , Malmiri , H.J. , Nehdi , I.A. , Cheah , Y.K. , Mirhosseini , H. and Baharin , B.S. 2011 . Effect of organic-phase solvents on physicochemical properties and cellular uptake of astaxanthin nanodispersions . Journal of Agricultural and Food Chemistry , 59 ( 16 ) : 8733 – 8741 .
- McClements , D.J. 2005 . Food Emulsions: Principles, Practice, and Techniques; , Boca Raton : CRC Press .
- Horn , D. and Rieger , J. 2001 . Organic nanoparticles in the aqueous phase-Theory, experiment, and use . Angewandte Chemie-International Edition , 40 ( 23 ) : 4330 – 4361 .
- Jafari , S.M. , He , Y. and Bhandari , B. 2006 . Nano-emulsion production by sonication and microfluidization—A comparison . International Journal of Food Properties , 9 ( 3 ) : 475 – 485 .
- Firebaugh , J.D. and Daubert , C.R. 2005 . Emulsifying and foaming properties of a derivatized whey protein ingredient . International Journal of Food Properties , 8 ( 2 ) : 243 – 253 .
- Rolim , Augusta , Biasutti , E. , Regina Vieira , C. , Capobiango , M. , Medeiros , Dias , Silva , V. and Pinto Coelho , Silvestre, M. 2007 . Study of some functional properties of casein: Effect of ph and tryptic hydrolysis . International Journal of Food Properties , 10 ( 1 ) : 173 – 183 .
- Chu , B.S. , Ichikawa , S. , Kanafusa , S. and Nakajima , M. 2008 . Stability of protein-stabilised β-carotene nanodispersions against heating, salts, and pH . Journal of the Science of Food and Agriculture , 88 ( 10 ) : 1764 – 1769 .
- Fernandez-Garcia , E. , Rincon , F. and Perez-Galvez , A. 2008 . Developing an emulsifier system to improve the bioaccessibility of carotenoids . Journal of Agriciculture and Food Chemistry , 56 ( 21 ) : 10384 – 10390 .
- HadjSadok , A. , Moulai-Mostefa , N. and Rebiha , M. 2010 . Rheological properties and phase separation of xanthan-sodium caseinate mixtures analyzed by a response surface method . International Journal of Food Properties , 13 ( 2 ) : 369 – 380 .
- Silva , H. , Cerqueira , M. and Vicente , A. 2011 . “ Nanoemulsions for food applications: Development and characterization ” . In Food and Bioprocess Technology 1 – 14 .
- Demetriades , K. and McClements , D.J. 1998 . Influence of pH and heating on physicochemical properties of whey protein-stabilized emulsions containing a nonionic surfactant . Journal of Agricultural and Food Chemistry , 46 ( 10 ) : 3936 – 3942 .
- Dickinson , E. 2010 . Flocculation of protein-stabilized oil-in-water emulsions . Colloids and Surfaces B: Biointerfaces , 81 ( 1 ) : 130 – 140 .
- McClements , D.J. 2004 . Protein-stabilized emulsions . Current Opinion in Colloid & Interface Science , 9 ( 5 ) : 305 – 313 .
- Mirhosseini , H. , Tan , C.P. , Hamid , N.S.A. and Yusof , S. 2008 . Optimization of the contents of Arabic gum, xanthan gum, and orange oil affecting turbidity, average particle size, polydispersity index, and density in orange beverage emulsion . Food Hydrocolloids , 22 ( 7 ) : 1212 – 1223 .
- Cheong , J.N. , Tan , C.P. , Man , Y.B.C. and Misran , M. 2008 . α-Tocopherol nanodispersions: Preparation, characterization, and stability evaluation . Journal of Food Engineering , 89 ( 2 ) : 204 – 209 .
- Yin , L.J. , Chu , B.S. , Kobayashi , I. and Nakajima , M. 2009 . Performance of selected emulsifiers and their combinations in the preparation of β-carotene nanodispersions . Food Hydrocolloids , 23 ( 6 ) : 1617 – 1622 .
- Ribeiro , H.S. , Chu , B.S. , Ichikawa , S. and Nakajima , M. 2008 . Preparation of nanodispersions containing β-carotene by solvent displacement method . Food Hydrocolloids , 22 ( 1 ) : 12 – 17 .
- Chanamai , R. and McClements , D.J. 2002 . Comparison of gum arabic, modified starch, and whey protein isolate as emulsifiers: Influence of pH, CaCl2, and temperature . Journal of Food Science , 67 : 120 – 125 .
- Charoen , R. , Jangchud , A. , Jangchud , K. , Harnsilawat , T. , Naivikul , O. and McClements , D.J. 2011 . Influence of biopolymer emulsifier type on formation and stability of rice bran oil-in-water emulsions: Whey protein, gum arabic, and modified starch . Journal of Food Science , 76 ( 1 ) : E165 – E172 .
- Anal , A.K. , Tobiassen , A. , Flanagan , J. and Singh , H. 2008 . Preparation and characterization of nanoparticles formed by chitosan-caseinate interactions . Colloids and Surfaces B: Biointerfaces , 64 ( 1 ) : 104 – 110 .
- Kulmyrzaev , A. , Chanamai , R. and McClements , D.J. 2000 . Influence of pH and CaCl2 on the stability of dilute whey protein stabilized emulsions . Food Research International , 33 ( 1 ) : 15 – 20 .
- Chen , J. and Dickinson , E . 1995 . interfacial interactions Part 3 . Competitive adsorption of protein + surfactant in emulsions. Colloids and Surfaces A: Physicochemical and Engineering Aspects , 101 ( 1 ) : 77 – 85 . Protein/surfactant
- Feng , S.S. and Huang , G. 2001 . Effects of emulsifiers on the controlled release of paclitaxel (Taxol®) from nanospheres of biodegradable polymers . Journal of Controlled Release , 71 ( 1 ) : 53 – 69 .
- Dalgleish , D.G. 2004 . “ Food emulsifiers: Their structures and properties ” . In Food emulsions Edited by: Friberg , S.E. , Larsson , K. and Sjoblom , J. 1 – 43 . New York Marcel Dekker
- Riscardo , M.A. , Franco , J.M. and Gallegos , C. 2003 . Influence of composition of emulsifier blends on the rheological properties of salad dressing-type emulsions . Food Science and Technology International , 9 ( 1 ) : 53 – 63 .
- Whitehurst , R.J. 2004 . Emulsifiers in Food Technology , U.K : Blackwell Publishing: Northampton .
- Dickinson , E. , Semenova , M.G. and Antipova , A.S. 1998 . Salt stability of casein emulsions . Food Hydrocolloids , 12 ( 2 ) : 227 – 235 .
- Bouyer , E. , Mekhloufi , G. , Potier , I.L. , Kerdaniel , T.D.F.D. , Grossiord , J.L. , Rosilio , V. and Agnely , F. 2011 . Stabilization mechanism of oil-in-water emulsions by β-lactoglobulin and gum arabic . Journal of Colloid and Interface Science , 354 ( 2 ) : 467 – 477 .
- Ercelebi , E.A. and Ibanoglu , E. 2007 . Influence of hydrocolloids on phase separation and emulsion properties of whey protein isolate . Journal of Food Engineering , 80 ( 2 ) : 454 – 459 .
- Dickinson , E. 2008 . Interfacial structure and stability of food emulsions as affected by protein-polysaccharide interactions . Soft Matter , 4 ( 5 ) : 932 – 942 .
- Guzey , D. and McClements , D.J. 2006 . “ Formation, stability, and properties of multilayer emulsions for application in the food industry ” . In Advances in Colloid and Interface Science 128 – 130 . 227–248
- Qian , C. , Xiao , H. , Decker , E.A. and McClements , D.J. 2011 . Comparison of biopolymer emulsifier performance in formation and stabilization of orange oil-in-water emulsions . Journal American Oil Chemists' Society , 88 : 47 – 55 .
- Chu , B.S. , Ichikawa , S. , Kanafusa , S. and Nakajima , M. 2007 . Preparation of protein-stabilized β-carotene nanodispersions by emulsification–evaporation method . Journal of the American Oil Chemists' Society , 84 ( 11 ) : 1053 – 1062 .
- Biggs , S. , Habgood , M. , Jameson , G.J. and Yan , Y.D. 2000 . Aggregate structures formed via a bridging flocculation mechanism . Chemical Engineering Journal , 80 ( 1–3 ) : 13 – 22 .
- Euston , S.R. , Finnigan , S.R. and Hirst , R.L. 2000 . Aggregation kinetics of heated whey protein-stabilized emulsions . Food Hydrocolloids , 14 ( 2 ) : 155 – 161 .
- Euston , S.R. , Finnigan , S.R. and Hirst , R.L. 2002 . Kinetics of droplet aggregation in heated whey protein-stabilized emulsions: Effect of polysaccharides . Food Hydrocolloids , 16 ( 5 ) : 499 – 505 .
- Yuan , Y. , Gao , Y. , Zhao , J. and Mao , L. 2008 . Characterization and stability evaluation of β-carotene nanoemulsions prepared by high pressure homogenization under various emulsifying conditions . Food Research International , 41 ( 1 ) : 61 – 68 .
- Euston , S.R. , Finnigan , S.R. and Hirst , R.L. 2001 . Aggregation kinetics of heated whey protein-stabilised emulsions: Effect of low-molecular weight emulsifiers . Food Hydrocolloids , 15 ( 3 ) : 253 – 262 .