Abstract
The aim of this work was to investigate the quality and microstructure of different frozen mango cultivars. Since freezing can cause severe textural damage to fruit, the proper selection of a ripening stage and cultivar can reduce such damage. Two mango cultivars (Nam Dok Mai and Chok Anan) were allowed to ripen for four ripening stages. During ripening, titratable acidity, alcohol-insoluble residues, and firmness significantly decreased while total soluble solids, total pectin, and water-soluble pectin levels increased. Mangoes from the three final ripening stages were frozen until the temperature of the samples reached –25°C. After thawing, the partially ripe mango samples exhibited the highest firmness, lowest drip loss, lowest water-soluble pectin, and the highest sensory firmness scores. The microstructure found using light microscopy and transmission electron microscopy showed that all frozen-thawed mangoes exhibited a swelling and folding of the cell wall due to freezing damage. The cell walls of fully ripe mangoes were dramatically damaged, which explained the low firmness values. Tissue and cell wall damage in Nam Dok Mai were the greatest, which contributed to the large drip loss. The cheaper Chok Anan cultivar was more resistant to freezing damage than Nam Dok Mai.
INTRODUCTION
Mango is an important fruit from the tropics and subtropical regions. It is a good source of nutrients, particularly rich in vitamin A and contains reasonable amounts of vitamins B and C and dietary fiber.[Citation1] Many mango cultivars are commercially grown in Thailand. Nam Dok Mai cultivar is a famous export mango of Thailand.[Citation2] It is a fiberless mango with smooth flesh and a deliciously unique flavor. In contrast to most mango cultivars, the Chok Anan cultivar has the ability to produce off-season flowering without chemical induction.[Citation3] This generates additional income for farmers by reducing the labor peak at harvest.
The ripening process of the mango fruit involves both physical and biochemical changes, which alters respiration, ethylene production, flavor, texture, aroma, and nutritional values.[Citation4] The change in color during the ripening process is caused by the degradation of chlorophyll and the accumulation of carotenoids. Medlicott et al.[Citation5] observed a rapid destruction of chlorophyll in the Tommy Atkins cultivar. Carotenoid levels increased during ripening whereas anthocyanin levels gradually decreased. Also, carbohydrates and starch converted into sugars, resulting in an increase in sweetness. Increases in the total soluble solids and total soluble sugars have also been reported in ripening Alphonso mangoes,[Citation6] which correlated with a similar observation in ripening Keitt mangoes.[Citation7] The cells in the pulp of the fruit are large and can lose their integrity due to cell wall hydrolysis during fruit ripening. The most common changes in the cell wall structure during ripening are related to pectins, which are the major components of primary cell wall and middle lamella. Softening of the fruit observed during ripening is due to the hydrolytic change of protopectin to pectin. The enzymatic demethylation and depolymerization of protopectin, such as pectin methylesterases and polygalacturonase, lead to the formation of low molecular weight compound with less methoxyl groups.[Citation8] For some tropical fruits, the alcohol-insoluble residue (AIR) and texture declined rapidly during ripening.[Citation9] It is known that fruit softening generally is accompanied by a decrease in the content of insoluble pectic substances with a concomitant increase in soluble polyuronides.[Citation6] The increase in total pectin, reported for ripe mangoes, was a consequence of higher free carboxylic groups due to de-esterification of pectins.[Citation6] In raspberries, although the proportion of total pectin in the AIR measurements did not change dramatically during ripening, there were modifications in the relative solubility of the pectin polymers. The water-soluble pectin (WSP) increased as the fruit ripening progressed.[Citation10]
Mango is a highly perishable fruit that possesses a limited shelf life. Mangoes can be stored without perishing for 4 to 8 days at room temperature and 2–3 weeks in cold storage at 13°C and 85–90% relative humidity.[Citation11] This short shelf life seriously limits the ability to commercially transport this fruit long distances. Therefore, preservation of the fruit could help to extend shelf life and support the growing popularity of off-season consumption.
Freezing is one of the most commonly used processes for preserving a wide range of foods both commercially and domestically. It delays spoilage and keeps foods safe by preventing microorganisms from growing and by slowing down enzyme activity. However, this process can cause texture degradation by causing freezing injuries to cell tissues. This tissue damage can be due to the presence of large amounts of water in fresh fruits, which turn into ice crystals after freezing. Freezing has been shown to induce significant changes in the texture, color, and soluble solids of mango and apple. The values of the studied texture parameters also decreased significantly after freezing.[Citation12] To limit these problems, some research projects have attempted to improve the texture quality of frozen mango by using various pre-treatments. One previous research indicated that initially less ripe mangoes could be softened somewhat by osmotic treatment, with firmness and cohesiveness maintained through frozen storage.[Citation13] Recently, Sriwimon and Boonsupthip[Citation14] reported that the use of partially ripe mango with impregnation of mango juice and sugar were better able to tolerate freezing damage than in ripe mangoes. Partially ripe mangoes better resisted the texture damage caused by ice crystals as a result of a stronger structure.
Microscopy is increasingly being used to study the influence of processing conditions and ingredients on food structure and structural research on some fruits affected by the freezing process has been reported in many studies. Shomer et al.[Citation15] observed ultrastructural injuries in both the exocarp and the mesocarp cells of thawed Madjhoul dates as viewed by transmission electron microscopy (TEM). The damaged cell walls appeared torn or crushed resulting in the formation of intracell-wall spaces. Fava et al.[Citation16] also found ultrastructural cell changes in blueberries during processing. These results from light microscopy (LM) and TEM demonstrate that folding and compression of the cell wall of the epicarp can occur during the freeze-thaw process. Furthermore, frozen-thawed apple slices presented the highest microstructure damage as compared to unfrozen and the other pre-treatments studied. LM results showed that the apple cells appeared irregular in shape and tissue distortion was observed due to the growing of ice crystals with cell separation.[Citation17] Otero et al.[Citation18] also found major damage in mango tissues due to the formation of ice crystals. Their LM micrographs indicated that there was also damage in the surface zone of the samples, which presented as broken cells.
Although several studies have focused on the ultrastructural changes in fruit tissues as a result of the freezing process and some researchers have attempted to improve the texture quality of frozen fruits by using various ripening processes, there have been no combination studies on how cultivar type, ripening stage, and freezing affect the microstructure and textural changes of mango tissues. Therefore, the objective of this study was to investigate the effect of cultivar and ripening stage on the quality and microstructure of frozen mangoes. Cell wall damage was examined using complementary microscopic techniques, i.e., LM and TEM. In this study, two cultivars (Nam Dok Mai and Chok Anan) and three ripening stages of mango were used to determine the conditions most resistant to freezing damage.
EXPERIMENTAL
Material
Two mango (Mangifera indica Linn.) cultivars Nam Dok Mai #4 and Chok Anan were purchased from a commercial orchard in Nakorn Ratchasima and Suphan Buri province, respectively. Mangoes were harvested at 16 weeks after fruit set. The experiment was carried out in March–April of 2011. The fruits were immediately transported to the laboratory at Kasetsart University, Bangkok, Thailand. The fruit weight ranges were 400–450 g (cv. Nam Dok Mai) and 290–340 g (cv. Chok Anan). Nam Dok Mai was 19–20 cm in length and 9–10 cm in width, while Chok Anan was 14–15 cm in length and 9–10 cm in width. The uniform maturity index was tested by floating mangoes in a NaCl solution. The mangoes, which sank in 10 g Kg−1 NaCl solution and floated in 30 g Kg−1 NaCl solution, were allowed to ripen at room temperature (32 ± 2°C, 70–75% relative humidity). The characteristics of mango fruit were observed after 0, 3, 4, and 5 days of ripening and 10–15 fruits were randomly sampled. The ripening stage was divided into four stages by visual characteristics, which were mature green (newly harvested, 100% green), partially ripe (30–60% yellow), ripe (60–99% yellow), and fully ripe (100% yellow) stages. Ranges of visual color index were based on peel color of six fruits at each ripening stage. The color was measured at the surface of the peel and pulp of mangoes in terms of L* (lightness), a* (greenness–redness), and b* (blueness–yellowness) using an UltraScan XE (HunterLab, Reston, VA, USA) based on the CIELAB color system. The L*, a*, and b* values were measured on six fruits at three different positions (stem, equatorial, and blossom end) of each fruit.
Total Soluble Solids (TSS) and Total Titratable Acidity (TA)
Total soluble solids (°Brix) were measured using a pocket digital refractometer (Type PAL-1, Atago, Tokyo, Japan). Total titratable acidity was determined according to the AOAC methods.[Citation19]
Freezing and Thawing
Mangoes at three different ripening stages (partially ripe, ripe, and fully ripe) were used in the freezing process. Approximately 20% from both the stem and the blossom end of the fruit were discarded, since those parts tend to be highly diverse in terms of fruit properties. Only the central parts (60%) of the fruits were used to minimize any variations within the samples. The samples were then washed, peeled, and cut into cubes of 1.5 cm in length. Eight cubes were then packed and sealed in each plastic pouch (NY/LLDPE, 70 μm, 100 × 150 mm). The weight of samples in each pouch was 28–30 g. The initial temperature of the samples was controlled at 23 ± 2°C. Samples were frozen at −40°C in a cryogenic freezer (Minibatch 1000L, Bangkok Industrial Gas Co., Thailand) until the temperature of the samples reached −25°C. In this study, the approximate freezing time of frozen mango was 30 min. Frozen mangoes were kept at −18°C in a chest freezer (Sanyo refrigerator, model SF-C1497, Sanyo Electric Co., Ltd., Osaka, Japan) for 15 days. Thawing was performed at 4°C after 5 h in a low-temperature incubator (Memmert, model IPP 400, Germany). The experiments were done in two trials.
Instrumental Textural Analysis
The firmness of fresh and frozen−thawed mango cubes was measured using a compression test Texture Analyzer (TA.XT. plus, Stable Micro Systems, UK). Firmness was performed using a cylindrical probe of 36 mm diameter with a 50 Kg load cell and 50% strain. The speed of the pre-test, test, and post-test were 1.5, 1.5, and 10 mm s−1, respectively. Firmness was determined by the maximum peak force. At least 12 mango cubes were measured for each stage analyzed.
Alcohol-Insoluble Residue and Cell Wall Fractions
The alcohol-insoluble residue (AIR) was extracted from approximately 30 g of fresh and frozen tissue (mesocarp) using 95% ethanol, 70% ethanol and acetone, respectively.[Citation20] The AIR was dried overnight at 40°C and weighed. Ten milligrams of AIR was solubilized in H2SO4 and then were assayed colorimetrically for total pectin (TP).[Citation21] Absorbance readings were evaluated against a standard curve of galacturonic acid. The results were expressed as micrograms of galacturonic acid per milligram of AIR.
Approximately 50 mg of AIR from each sample was suspended in 25 mL of water and stirred at room temperature for 30 min. The samples were then centrifuged at 7000× g and 4°C for 15 min, the supernatant was filtered through Whatman no. 1 filter paper. The procedure was repeated three times. The filtrate was designated the water-soluble fraction and assayed for water-soluble pectin (WSP) as described above.
Drip Loss
Frozen samples were placed over absorbent paper in zip-lock plastic bags and thawed at 4°C for 5 h. Drip loss was determined by a method adapted from Lowithun and Charoenrein.[Citation22]
Microstructure
For light and transmission electron microscopy observations
Sections of fresh and frozen mango mesocarp (1 × 1 × 2 mm) were obtained from the inner part of the fruit at a distance of ˜1.5 cm from the center of the seed with a razor blade. Samples were prefixed with 2.5% glutaraldehyde in a 0.1 M phosphate buffer (pH 7.2) overnight at 4°C. After washing with a phosphate buffer, the samples were then postfixed with 2% osmium tetroxide for 2 h at room temperature. The fixed tissues were washed three times with distilled water and dehydrated stepwise with an ethanol series (30–100%) and propylene oxide. The dehydrated tissues were infiltrated with propylene oxide: Spurr's resin (2:1, 1:1 and 1:2) and 100% Spurr's resin, each for overnight, respectively. Then tissues were polymerized in Spurr's resin for 7 h at 80°C.
Sections were cut using an ultramicrotome (Leica Ultracut UCT, Vienna, Austria) at a 1.5 μm thickness and stained with 1% toluidine blue for observation under a light microscope (Leica DME, Leica Microsystems Inc., NY, USA). For the transmission electron microscope, ultra-thin sections (60 nm) were obtained using an ultramicrotome, stained with uranyl acetate and lead citrate, and examined using a JEOL−JEM−1220 TEM (Tokyo, Japan) at 80 kV. Five specimens from each ripening stage of the two cultivars were examined. At least 500 cells of each sample were viewed under a light microscope. Mean cell size in length and diameter were measured on 50 cells of partially ripe mango in both cultivars. During TEM examination, six cells were randomly chosen to measure the thickness of cell walls for each ripening stage of both cultivars. The thickness of cell walls was measured from the outer part of cell to cell using three points. The average thickness was calculated as the average value of three points measurement divided by two.
Sensory Evaluation
Sensory attributes were evaluated using a liking score (appearance, color, flavor, taste, texture, and overall acceptability) on a 9-point hedonic scale (1 = dislike extremely, 5 = neither like nor dislike, and 9 = like extremely) by 30 untrained panelists.
Statistical Analysis
A completely randomized design was used in this experiment. Analysis of variance (ANOVA) and Duncan's new multiple range test were used to determine statistically significant differences (P ≤ 0.05) of the mean values.
RESULTS AND DISCUSSION
Mango Ripening Characteristic
The four ripening stages of the two cultivars (Nam Dok Mai and Chok Anan) were categorized using a visual characteristic and color measurement as mature green, partially ripe, ripe, and fully ripe stage (). The visual skin color of the two different cultivars changed noticeably during fruit ripening. The peel color changed from green to yellow due to the degradation of chlorophyll and the presence of carotenoids.[Citation6] The pulp color changed from a light white-green to yellow or orange due to carotenoids synthesis. Color changes in the peel and pulp are presented as L*, a*, and b* values. The data are shown in . During the peel color development of both cultivars, the peel a* and b* values were found to increase from mature green to fully ripe stage. The L* value of Chok Anan was revealed to increase from mature green to partially ripe stage, with no increase afterward. In contrast, the L* value of Nam Dok Mai was not significantly different (P > 0.05) between ripening stages. Chok Anan cultivar had higher peel a* and b* values than that of Nam Dok Mai cultivar throughout ripening, whereas the peel L* value was not significantly different (P > 0.05) between cultivars.
Table 1 Changes in mango peel and pulp color during ripening
Figure 1 Change in mango peel and pulp color during ripening: (a and b) Nam Dok Mai; (c and d) Chok Anan. (Color figure available online.)
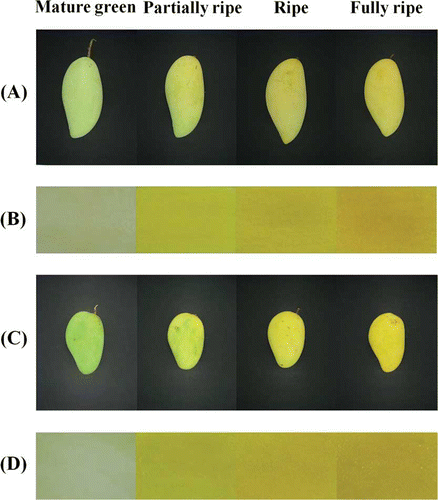
In both cultivars, the L* value of mango pulp was found to decrease slightly during mango ripening, while the a* and b* value of mango pulp increased (). There was a gradual increase in the a* value through the ripening process, while the b* value revealed a rapid increase from mature green to partially ripe stage with no increase afterward. These results indicated that the mango pulp potentially developed a full yellow color during the early stages of ripening, however continued to accumulate red pigments (carotenoids) until the full ripe stage is attained, as indicated by the deep orange color of the mango pulp.[Citation5] The increases in the a* and b* values appeared to correlate well with the visual color development as shown in . In addition, Chok Anan had a lower L* value and higher b* value of mango pulp than Nam Dok Mai during ripening, while the a* value was not significant difference (P > 0.05) between cultivars.
The amount of %TA significantly decreased although the TSS increased during the fruit ripening process (). In both cultivars, fruit firmness decreased with each ripening stage. Similar results were found in different mango cultivars during fruit ripening.[Citation23] Chok Anan had a significantly higher firmness value than Nam Dok Mai at all ripening stages except the ripe stage (P ≤ 0.05). In addition, Jarimopas and Kitthawee[Citation24] also found that the Chok Anan cultivar was firmer than the Nam Dok Mai throughout the development period. Although fruit firmness can be affected by several factors, predominantly fruit textural changes are thought to be a consequence of changes in the composition of the cell wall.[Citation12]
Table 2 Changes in fruit properties of two mango cultivars during ripening
To determine the changes in cell wall composition, the AIR, WSP, and TP values were analyzed (). The AIR values decreased markedly from the mature green to the fully ripe stage of Nam Dok Mai and Chok Anan from 513.01 to 93.37 and 640.32 to 120.76 mg g−1 dry basis, respectively. These values may indicate that large alcohol-insoluble polymers are being degraded to shorter alcohol-soluble polymers during fruit ripening. In addition, the decrease in AIR may be due to the conversion of starch to soluble sugars and conversion of fiber to alcohol-soluble solids.[Citation9] The degradation of starch during ripening Keitt mangoes involved with enzymes, such as α–amylase and β–amylase. The soluble sugars found in the fruit are glucose and fructose, but in lower amounts than sucrose.[Citation25] An apparent decrease in AIR during fruit ripening was also reported in the Dashehari mango,[Citation26] Zebda and Baladi mango,[Citation9] and the Tommy Atkins and Keitt mangoes.[Citation27] In this study, we also found that the softening of mangoes during ripening () was accompanied by a rapid decrease in AIR. Accordingly, a recent study on the blueberry reported that fruit firmness and AIR decreased as blueberry ripening progressed.[Citation28] TP increased markedly from the mature green to fully ripe stage in both mango cultivars, while the fruit firmness decreased. Pectin is the major carbohydrate of ripe fruits, whereas starch constituted the bulk of the AIR of mature green fruits. The increase in pectin content with ripening was a consequence of the generation of a higher proportion of free carboxylic groups due to de-esterification of pectins.[Citation6] A clear increase in WSP fraction was observed in the Nam Dok Mai and Chok Anan cultivars through the different ripening stages. A similar observation in the ripening of Alphonso mangoes showed that WSP had undergone a drastic depolymerization during mango ripening, which contributed to the tissue softening and textural changes.[Citation6] However, Chok Anan retained a significantly higher firmness than Nam Dok Mai at all ripening stages, except the ripe stage. These textural differences between the two cultivars may be the result of differences in cell wall composition and a greater amount of the AIR in the Chok Anan variety.
Quality of Frozen Mangoes
In this study, the freezing rate by cryogenic freezer was approximately 1.5°C min−1. The drip loss of the frozen-thawed Nam Dok Mai and Chok Anan samples increased with increasing ripening stage (). The fully ripe mango samples had a lower firmness value than the ripe and partially ripe samples, which likely contributed to the increased drip loss. Similar results have also been found in the thawing process of thornless blackberries for which drip loss is more extensive in fully ripe than in slightly under-ripe fruit.[Citation29] Wenzel et al.[Citation30] also reported that drip losses of thawing strawberries increased in riper fruits. Furthermore, Sistrunk[Citation31] observed greater drip losses with over-ripe than with ripe strawberries, which he attributed to the release of pectic enzymes in the over-ripe fruit. However, the Nam Dok Mai cultivar had a higher drip loss than the Chok Anan at all ripening stages. At the fully ripe stage, which has the highest drip loss, many of the thawed mango pieces had collapsed, giving these samples an unattractive appearance. This result could be due to tissue damages during freezing as a result of the higher moisture content and lower firmness value of fresh Nam Dok Mai. These results are supported by microstructure results in which Nam Dok Mai appeared to have more cell wall damage than in the Chok Anan samples after thawing.
Figure 2 Changes in frozen-thawed mango drip loss (a) and fruit firmness (b) of Nam Dok Mai and Chok Anan at various ripening stages. Means with the different letters (a–c) are significantly different (DMRT's test: P ≤ 0.05) between ripening stages. Mean with the different letters (A–B) are significantly different (ANOVA test: P ≤ 0.05) between cultivars.
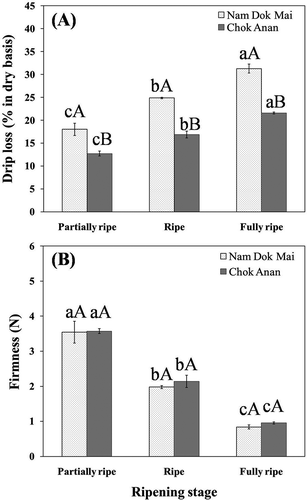
A texture measurement of the frozen-thawed mangoes showed that the firmness value decreased with the increasing ripening stage (). The firmness value of both cultivars at the fully ripe stage was extremely low. These results suggest that partially ripe mangoes can maintain a higher firmness value for frozen-thawed samples than for any of the other ripening stages. Although the firmness value of Nam Dok Mai and Chok Anan were relatively similar between cultivars, drip losses were significantly different (P ≤ 0.05) at all ripening stages.
After freezing and thawing, the decrease in AIR of the ripe and fully ripe mangoes was more pronounced than that of the partially ripe mangoes (). This suggests that changes were taking place in the cell wall material during the frozen-thawed process. The changes in AIR were also associated with a loss of firmness. It has been reported in green beans, peaches, and strawberries that changes in pectic material was a consequence of freezing and frozen storage.[Citation32] TP values were relatively similar in the fresh samples for all ripening stages, whereas WSP values increased. This may indicate that no changes occurred in TP content. However, this does not mean that there were no changes in the cell wall composition. The increase in WSP contents of Nam Dok Mai at all ripening stages were significantly different (P ≤ 0.05) between fresh and frozen samples, while the increase in WSP contents of Chok Anan mango were not significantly different (P > 0.05). The increase in WSP may be due to the solubilization or depolymerization of the cell wall composition caused by ice crystal formation during freezing and ice melting during the thawing process. Frozen-thawed mango Nam Dok Mai and Chok Anan at the fully ripe stage had the highest WSP values. These results correspond with the texture results, i.e., lower firmness values correlate with higher WSP values. Although, Chok Anan had higher WSP values than Nam Dok Mai at the partially ripe and the ripe stage, the fruit firmness of the frozen-thawed mangoes had no significant differences (P > 0.05) in either cultivar. In frozen cherries, texture changes have been associated with the composition of the pectin fractions and not with the quantity of pectin in the fruit.[Citation33] Further study of the composition of pectin fractions in frozen mango is in progress.
Table 3 Alcohol insoluble residue (AIR), water soluble pectin (WSP), and total pectin (TP) of frozen-thawed mangoes
Microstructure of Fresh and Frozen Mangoes
The microstructure of frozen and unfrozen mango tissues was observed using LM and TEM. The observation using LM showed that during the ripening of Nam Dok Mai and Chok Anan mango, the initially round shaped cells became wavy ( and ) with the fully ripe stage exhibiting the most wavy wall surface (-3, -3). The structural changes, which occurred in the middle lamella and primary cell wall during ripening, lead to cell separation and softening of the tissues.[Citation34] The mean cell sizes of Nam Dok Mai were 141 ± 26 μm in length and 101 ± 18 μm in diameter while the mean cell sizes of Chok Anan were 124 ± 18 μm in length and 89 ± 16 μm in diameter. The cell sizes were measured at the partially ripening stage in both cultivars. Chok Anan cultivar, which had smaller cell sizes and cells that were relatively more compact than Nam Dok Mai cultivar, also showed a higher firmness value.
Figure 3 Light microscopy images of fresh and frozen mangoes. (a) Fresh Nam Dok Mai, (b) fresh Chok Anan, (c) frozen Nam Dok Mai, and (d) frozen Chok Anan. (1) Partially ripe, (2) ripe, and (3) fully ripe stage. Arrows indicate the swelling of cell walls caused by freezing damage. T = torn cell wall. Bar = 50 μm. (Color figure available online.)
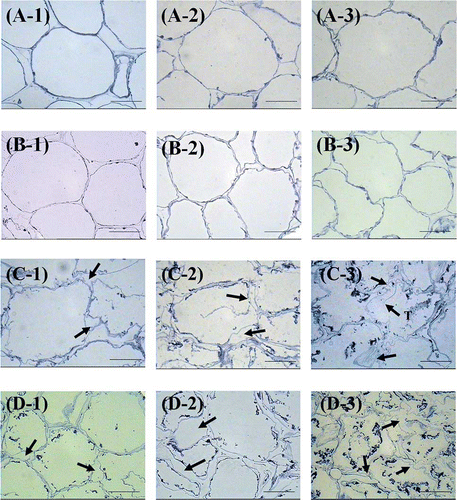
After the freezing process, the swelling and folding of the cell wall appeared in both mango cultivars ( and ). These irregular shapes can be observed by light microscope in the frozen tissue. Chok Anan sample at the ripe and fully ripe stages showed a larger swelling of the cell wall than in the Nam Dok Mai sample. This dramatic cell wall damage (i.e., cell separation in the middle lamella and cell wall rupture) was found in the fully ripe stage of both frozen mango cultivars (-3, -3) resulting in a reduction in the firmness values. This can be explained by the ice crystals that formed in the extracellular medium propagating via the cytoplasm when the cell membrane lost permeability. The decompartmentalization caused by these ice crystals prevented water from returning to the intracellular medium during thawing, which caused a loss of turgidity and, hence, the change in fruit texture.[Citation35]
TEM micrographs of the fresh and frozen mango samples are shown in . In the unfrozen tissue, the cell wall and middle lamella are obviously intact ( and ). However, after freezing, cell wall damage can be observed in both Nam Dok Mai and Chok Anan samples ( and ). This cell wall damage can be explained by the dissolution of middle lamella, which was caused by ice crystals. It was correlated with an increase in WSP content of frozen mango. The dissolution of the middle lamella led to the loss of cell adhesion and cell-to-cell contact, which resulted in the formation of intercellular spaces. The swelling and folding of cell walls were observed in the frozen tissue, which were possibly due to cell wall loosening and cell wall expansion. TEM observation revealed that the cell wall thickness of fresh Nam Dok Mai and Chok Anan samples ranged from 0.5 to 1.0 and 0.8 to 1.6 μm, respectively. After freezing, the cell wall thickness of Nam Dok Mai and Chok Anan was increased in the range from 1.0 to 2.0 and 1.0 to 3.0 μm, respectively. The cell walls at the partially ripe stage for both cultivars appeared less damaged than in the other stages. Severe cell wall damage was found in the fully ripe stage of both cultivars (-, -). Nam Dok Mai at all ripening stages seemed to have more cell wall damage than Chok Anan. This result may relate to the higher drip loss in the Nam Dok Mai cultivar () even though both cultivars had relatively similar moisture contents. From the microstructure and textural results, it is clear that the loss in firmness in the mango tissues after freezing and thawing can be attributed to changes in the middle lamella and cell wall.
Figure 4 TEM images of two cultivars of fresh and frozen mangoes. (a) Fresh Nam Dok Mai, (b) fresh Chok Anan, (c) frozen Nam Dok Mai, and (d) frozen Chok Anan. (1) Partially ripe, (2) ripe, and (3) fully ripe stage. Arrows indicate the swelling and folding of cell walls caused by freezing damage. CW: cell wall; ML: middle lamella; ICS: intercellular space. Bar = 1 μm (C-3 image bar = 2 μm).
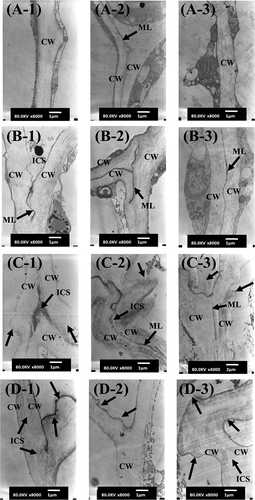
Sensory Evaluation
The sensory attributes of the frozen-thawed mangoes were evaluated by 30 untrained panelists (). These results showed that Nam Dok Mai at the partially ripe and ripe stages had improved appearance, color, and overall acceptability scores than for the fully ripe stage. The flavor and taste scores of Nam Dok Mai were not significantly different (P > 0.05) between any of the ripening stages. However, the partially ripe stage presented the highest texture score. In Chok Anan, the partially ripe and ripe stage had higher color scores than for the fully ripe stage. In addition, the flavor and taste scores for all ripening stages of Chok Anan were not significantly different (P > 0.05). The partially ripe Chok Anan samples had the highest appearance, texture, and overall acceptability scores. The different ripening stages used in the freezing process affected the sensory attributes of both mango cultivars. The sensory attributes at the partially ripe and fully ripe stage were not significantly different between cultivars (P > 0.05). However, Nam Dok Mai at the ripe stage had a higher appearance, color, and overall acceptability score than that found in Chok Anan.
Table 4 Mean sensory scores of two different cultivars of frozen-thawed mangoes at various ripening stages
CONCLUSION
The ripening stage had a significant effect on the firmness values, drip loss, WSP, microstructure, and sensory attributes of both frozen-thawed mango cultivars. The partially ripe stage exhibited a higher firmness value, higher sensory scores, and lower drip loss than the other stages. This stage also appeared to have less cell wall damage caused by the freezing and thawing process. The use of the partially ripe stage of mangoes can be recommended in the freezing process to maintain higher texture quality. In addition, the results found here were relatively similar for the two cultivars studied. However, Chok Anan demonstrated a high resistance to freezing damage and had a very low drip loss and less cell wall damage as compared to Nam Dok Mai.
ACKNOWLEDGMENTS
The authors gratefully acknowledge the funding support from the Thailand Research Fund under project RSA 5480020 and from the Graduate Study Research for International Publication, the Graduate School, Kasetsart University.
REFERENCES
- Telis , V.R.N. and Telis-Romero , J. 2007 . Freezing point and thermal conductivity of tropical fruit pulps: Mango and papaya . International Journal of Food Properties , 10 : 73 – 84 .
- Sirisomboon , P. and Pornchaloempong , P. 2011 . Instrumental textural properties of mango (cv Nam Doc Mai) at commercial harvesting time . International Journal of Food Properties , 14 : 441 – 449 .
- Titiprasert , V. , Chintanawong , S. , Chaichakan , M. and Lee-Angoonsathian , N. 2001 . “ Plant Variety Protection Office Department of Agriculture, Ministry of Agriculture and Cooperatives ” . In Plant Germplasm Database for Mango , 142 Bangkok : in Thai language .
- Lizada , C. 1993 . “ Biochemistry of Fruit Ripening ” . In Mango , Edited by: Seymour , G.B. , Taylor , J.E. and Tucker , G.A. 255 – 270 . London : Chapman and Hall .
- Medlicott , A.P. , Bhogol , M. and Reynolds , S.B. 1986 . Changes in peel pigmentation during ripening of mango fruit (Mangifera indica var . Tommy Atkins). Annals of Applied Biology , 109 : 651 – 656 .
- Yashoda , H.M. , Prabha , T.N. and Tharanathan , R.N. 2006 . Mango ripening: Changes in cell wall constituents in relation to textural softening . Journal of the Science of Food and Agriculture , 86 : 713 – 721 .
- Medlicott , A.P. and Thompson , A.K. 1985 . Analysis of sugars and organic acids in ripening mango fruits (Mangifera indica L, var . Keitt) by high performance liquid chromatography. Journal of the Science of Food and Agriculture , 36 : 561 – 566 .
- Sidhu . 2006 . “ Handbook of Fruits and Fruit Processing ” . In J.S. Tropical fruits: Guava, lychee and papaya , Edited by: Hui , Y.H. 595 – 634 . Ames, IA : Blackwell Publishing .
- El-Zoghbi , M. 1994 . Biochemical changes in some tropical fruits during ripening . Food Chemistry , 49 ( 1 ) : 33 – 37 .
- Vicente , A.R. , Ortugno , C. , Powell , A.L.T. , Greve , L.C. and Labavitch , J.M. 2007 . Temporal sequence of cell wall disassembly events in developing fruits. 1. Analysis of raspberry (Rubus idaeus) . Journal of Agriculture and Food Chemistry , 55 ( 10 ) : 4119 – 4124 .
- Carrillo-Lopez , A. , Ramirez-Bustamante , F. , Valdez-Torres , J.B. , Rojas-Villegas , R. and Yahia , E.M. 2000 . Ripening and quality changes in mango fruit as affected by coating with an edible film . Journal of Food Quality , 23 ( 5 ) : 479 – 486 .
- Chassagne-Berces , S. , Fonseca , F. , Citeau , M. and Marin , M. 2010 . Freezing protocol effect on quality properties of fruit tissue according to the fruit, the variety and the stage of maturity . LWT–Food Science and Technology , 43 ( 9 ) : 1441 – 1449 .
- Rincon , A. and Kerr , W.L. 2010 . Influence of osmotic dehydration, ripeness and frozen storage on physicochemical properties of mango . Journal of Food Processing and Preservation , 34 ( 5 ) : 887 – 903 .
- Sriwimon , W. and Boonsupthip , W. 2011 . Utilization of partially ripe mangoes for freezing preservation by impregnation of mango juice and sugars . LWT–Food Science and Technology , 44 ( 2 ) : 375 – 383 .
- Shomer , I. , Borochov-Neori , H. , Luzki , B. and Merin , U. 1998 . Morphological, structural and membrane changes in frozen tissues of Madjhoul date (Phoenix dactylifera L.) fruits . Postharvest Biology and Technology , 14 ( 2 ) : 207 – 215 .
- Fava , J. , Alzamora , S.M. and Castro , M.A. 2006 . Structure and nanostructure of the outer tangential epidermal cell wall in Vaccinium corymbosum L . (blueberry) fruits by blanching, freezing-thawing and ultrasound. Food Science and Technology International , 12 ( 3 ) : 241 – 251 .
- Ramirez , C. , Troncoso , E. , Munoz , J. and Aguilera , J.M. 2011 . Microstructure analysis on pre-treated apple slices and its effect on water release during air drying . Journal of Food Engineering , 106 ( 3 ) : 253 – 261 .
- Otero , L. , Martino , M. , Zaritzky , N. , Solas , M. and Sanz , P.D. 2000 . Preservation of microstructure in peach and mango during high-pressure-shift freezing . Journal of Food Science , 65 ( 3 ) : 466 – 470 .
- AOAC . 1995 . Official Method of Analysis of the Association of Analytical Chemists , 16th , 10 – 11 . Arlington , VA : AOAC International .
- McFeeters , R.F. and Armstrong , S.A. 1984 . Measurement of pectin methylation in plant cell walls . Analytical Biochemistry , 139 ( 1 ) : 212 – 217 .
- Blumenkrantz , N. and Asboe-Hansen , G. 1973 . New method for quantitative determination of uronic acids . Analytical Biochemistry , 54 ( 2 ) : 484 – 489 .
- Lowithun , N. and Charoenrein , S. 2009 . Influence of osmodehydrofreezing with different sugars on the quality of frozen rambutan . International Journal of Food Science and Technology , 44 ( 11 ) : 2183 – 2188 .
- Raju , P.S. , Jagannath , J.H. , Ashok , N. , Das Gupta , D.K. and Bawa , A.S. 2006 . Nondestructive monitoring of ripeness in mango cultivars by acoustic resonance spectroscopy . International Journal of Food Properties , 9 : 487 – 501 .
- Jarimopas , B. and Kitthawee , U. 2007 . Firmness properties of mangoes . International Journal of Food Properties , 10 ( 4 ) : 899 – 909 .
- Simao , R.A. , Silva , A.P.F.B. , Peroni , F.H.G. , Nascimento , J.R.O. , Louro , R.P. , Lajolo , F.M. and Cordenunsi , B.R. 2008 . Mango starch degradation . I. A microscopic view of the granule during ripening. Journal of Agricultural and Food Chemistry , 56 : 7410 – 7415 .
- Tandon , D.K. and Kalra , S.K. 1983 . Changes in sugars, starch and amylase activity during development of mango fruit cv . Dashehari. Journal of Horticultural Science , 58 : 449 – 454 .
- Mitcham , E.J. and McDonald , R.E. 1992 . Cell wall modification during ripening of ‘Keitt’ and ‘Tommy Atkins’ mango fruit . Journal of the American Society for Horticultural Science , 117 ( 6 ) : 919 – 924 .
- Vicente , A.R. , Ortugno , C. , Rosli , H. , Powell , A.L.T. , Greve , L.C. and Labavitch , J.M. 2007 . Temporal sequence of cell wall disassembly events in developing fruits. 2. Analysis of blueberry (Vaccinium Species) . Journal of Agriculture and Food Chemistry , 55 ( 10 ) : 4125 – 4130 .
- Sapers , G.M. , Burgher , A.M. , Jones , S.B. and Phillips , J.G. 1987 . Factors affecting drip loss from thawing thornless blackberries . Journal of the American Society for Horticultural Science , 112 ( 1 ) : 104 – 109 .
- Wenzel , W.G. , Calitz , F.J. and Hurter , N. 1976 . Screening strawberry clones for freezing ability . Agroplantae , 8 ( 1 ) : 7 – 9 .
- Sistrunk , W.A. 1963 . Field conditions and processing practices relating to frozen strawberry quality . Proceedings of the American Society for Horticultural Science , 83 : 440 – 446 .
- Reid , D.S. , Carr , J.M. , Sajjaanantakul , T. and Labavitch , J.M. 1986 . “ American Chemical Society: ” . In Chemistry and Function of Pectins; Edited by: Fishman , M.L. and Jen , J.J. 200 – 216 . Washington , DC Effect of freezing and frozen storage on the characteristics of pectin extracted from cell wallsInEds.
- Alonso , J. , Canet , W. and Rodriguez , T. 1997 . Thermal and calcium pretreatment affects texture, pectinesterase and pectic substances of frozen sweet cherries . Journal of Food Science , 62 ( 3 ) : 511 – 515 .
- Carrillo-Lopez , A. , Carabez-Trejo , A. , Guevara-Lara , F. and Paredes-Lopez , O. 2002 . Hydrolytic activity and ultrastructural changes in fruit skins from two prickly pear (Opuntia sp.) varieties during storage . Journal of Agriculture and Food Chemistry , 50 ( 6 ) : 1681 – 1685 .
- Alonso , J. , Tortosa , M.E. , Canet , W. and Rodriguez , M.T. 2005 . Ultrastructural and changes in pectin composition of sweet cherry from the application of prefreezing treatments . Journal of Food Science , 70 ( 9 ) : 526 – 530 .