Abstract
In this article the possibility to detect adulteration of costly olive oils with cheaper vegetable oils using fluorescence spectroscopy is studied. Total luminescence spectra were recorded by measuring the emission spectra in the range 350 nm to 720 nm for excitation wavelengths from 375 nm to 450 nm. Fluorescence spectra of 12 types of olive oil samples were studied. Ten of the olive oil types were purchased locally, while two (samples 1 and 4) were obtained directly from Greek olive oil producers. Analysis of the fatty acid and the tocopherol contents has been performed. Two of the samples exhibit the content of sunflower oils, two are admixtures of sunflower and olive oils, while the remaining eight samples are natural olive oils. The samples show differences in their fluorescence spectra. The latter fact shows that fluorescence spectroscopy can be used for the quick identification of possible adulterations of olive oil, although a more detailed gas chromatographic analysis is needed for the exact quantitative determination of the content of the adulterant.
INTRODUCTION
Olive oil is an important product in the Mediterranean countries. It is well known for being beneficial to health. It prevents several types of cancer and reduces the risk factors for coronary heart disease.[Citation1] Virgin olive oil (VOO) is derived from the fruit of the olive tree solely by mechanical or other physical means under conditions that do not lead to any chemical change.[Citation2,Citation3] Olive oil is an expensive product and is therefore often adulterated with cheaper vegetable oils such as rapeseed, soybean, and sunflower oil. Adulteration with corn oil and nut bean oils is less frequent due to their higher price. A number of analytical techniques can be used to differentiate VOO from adulterated ones which are based on the analysis of fat acid and tocopherol content. Tocopherols are important biological antioxidants. Alphatocopherol, or vitamin E, prevents oxidation of body lipids including polyunsaturated fatty acids and lipid components of cells and organelle membranes. Tocopherol has been associated with the reduction of heart disease, the delay of Alzheimer's disease, and the prevention of cancer. Tocopherols have widely been used for food, pharmaceuticals, cosmetics, and resins. In food, tocopherols are used as an antioxidant for frying oil, margarine, fried snacks. Tocopherol content is specific for each vegetable oil and can be used to track down traces of adulterants. Sunflower and olive oil basically contain -tocopherol (over 90%), while soybean and rapeseed oils contain larger quantities of
- and
-tocopherols.
Most of the analytical techniques for the detection of adulterants rely upon chromatographic methods such as gas and high performance liquid chromatography (GC and HPLC).[Citation4,Citation5] However, these methods are time consuming, expensive, require skilled operators, and produce hazardous chemical waste. Fluorescence spectroscopy can be used as an alternative to these methods since it is non-destructive, does not require chemical reagents, can be processed in real time, and is comparatively cheaper. Recently fluorescence spectroscopy has been applied to determine the authenticity of vegetable oils. Thus, Sauago et al.[Citation6] used fluorescence spectroscopy to detect walnut oil in olive oil. Dupuy et al.[Citation7] used excitation-emission matrix (EEM), obtained by taking the fluorescence spectra at several different wavelengths, to identify the olive oils according to the geographical area. Guimet et al.[Citation8] suggest a fast method to identify pomace olive oil in extra virgin olive oil by using the EEM method. Teresa Cecchi et al.[Citation9] investigated the commercial, sensory, and analytical characteristics of extra virgin olive oil from the Marche region (Italy). The objective of the present article is to study fluorescence spectra from VOO and olive oil adulterated with sunflower or other types of vegetable oil using a fiber optic spectrometer, as well as ultra violet (UV) and visible light emitting diodes (LEDs), and laser diodes (LDs) for probe excitation.
MATERIALS AND METHODS
Samples
Twelve types of olive oils labeled from 1 to 12 were investigated. The sunflower oil was obtained from manufacturers. Ten of the olive oil types were purchased locally, while two (samples 1 and 4) were obtained directly from Greek olive oil producers. In this study, these samples have been used as references since analysis shows that their parameters fully correspond to the standards recommended for extra virgin olive oil. Their basic characteristics are compared to those recommended by the standards in . presents data on the time duration of samples in the commercial premises, the production and expiry dates, as well as the storage conditions before and after purchase. In the commercial premises olive oils have been kept in dark bottles at room temperature and at a temperature of 20°C after purchase. The chemical analysis and the analysis of the physical parameters as well as the fluorescence spectra were made within 24 h after opening the bottle. The data presented in the corresponding tables or in graphs are reliable because they were obtained from two or three samples of each type of oil numbered from 1 to 12. The validity of the method presented in this article is tested for over 12 samples. For the purpose of testing the repeatability of results, several samples (in small and large bottles, from different batches and different bottling dates) of each olive oil type of the same manufacturer were used. Due to the minor differences in results, the averaged data is presented for 12 different types of oils. presents the number of analyzed samples of each type and the expiry date, as well as the production of the first (the oldest) purchased sample of a given type.
Table 1 Comparison between the physic-chemical parameters of the olive oil control samples and the recommended values for the standard of extra virgin olive oil
Table 2 Information on expiry dates and the storage duration of the investigated olive oil samples
Instruments and Software
below shows the experimental set-up used to measure the fluorescence spectra. The sources used are a 405 nm violet LD and 370 nm, 395 nm, 425 nm, and 450 nm LEDs. A fiber optic spectrometer (AvaSpec-2038, Avantes) with a sensitivity in the 200–1100 nm range and a resolution of about 5 nm was used to measure the fluorescence spectra. The oil samples were placed in a 10 mm x 10 mm cuvette and irradiated by LDs or LEDs. Fluorescence spectra were taken from a direction orthogonal to the line of transmission, as shown in the .
In the present study, the excitation-emission matrices are obtained not by the use of a Xenon lamp and a monochromator, but by the use of the above LEDs and LD. The advantage of the use of LEDs is the simplicity and cost effectiveness of the measurement set-up. The use of a fiber-optic spectrometer allows the spectrum in immediate proximity to the sample, which eliminates the need to prepare solutions with n-Hexane, to be obtained. The data from the fiber-optic spectrometer was exported to ASCII code and excitation-emission matrixes were obtained with using Matlab software.
Analyse of Lipids
Tocopherols
Tocopherols in the oils were determined directly by HPLC on a “Merck-Hitachi" (Merck, Darmstadt, Germany) instrument equipped with 250 mm × 4 mm Nucleosil Si 50-5 column (Merck, Darmstadt, Germany) and fluorescent detector “Merck-Hitachi” F 1000. The operating conditions were as follows: mobile phase of n-hexane: dioxan 96:4 (by volume), flow rate 1 cm3/min, excitation 295 nm, emission 330 nm. A 20 μl 1% solution of crude oils were injected. Tocopherols were identified by comparing the retention times with those of authentic individual tocopherols. The tocopherol content was calculated on the basis of tocopherol peak areas in the sample versus tocopherol peak area of standard α-tocopherol solution as shown.[Citation10]
Fatty acids
The total fatty acid composition, as well as the fatty acid composition of phospholipids and sterol esters, was determined by GC after transmethylation of the respective sample with 2N methanolic KOH at 50oC according to Christie.[Citation11] Fatty acid methyl esters (FAME) were purified by TLC on 20x20 cm plates covered with 0.2 mm Silica gel 60 G layer (Merck, Darmstadt, Germany) with mobile phase n-hexane:acetone 100:8 (by volume). GC was performed on a HP 5890 (Hewlett Packard GmbH, Austria) gas chromatograph equipped with a 30 mm × 0.25 mm (I.D.) capillary InnoWax column (cross-linked PEG, Hewlett Packard GmbH, Austria) and a flame ionization detector (FID). The column temperature was programmed from 165 to 240oC at 4oC/min and held at this temperature for 10 min; injector and detector temperatures were 260oC. Hydrogen was the carrier gas at a flow rate 0.8 cm3/min; split was 100:1. Identification was performed by comparison of retention times with those of a standard mixture of fatty acids subjected to GC under identical experimental conditions.[Citation12] Lovibond PFX 880 (Tintometer Limited, UK) has been used for determining the -carotene and chlorophyl content in samples of olive oil. The refractive indices (RI) of olive oils are measured at t = 20oC for λ = 589.3 nm using a standard Abbe refractometer (Carl Zeis Jena, Germany).
RESULTS AND DISCUSSIONS
The fatty acid contents of 12 samples of olive oils from those available on the market have been analyzed and the analysis is presented in . As is well known, olive oil is characterized by a higher contents of oleic acid (between 55 and 83%) and a lower contents of linoleic acid (between 3.5 and 21%).[Citation13]
The analysis of fatty acid composition shows that samples 9, 10, 11, and 12 contain a lower level of oleic and a higher level of linoleic acid. The latter can be associated with the addition of cheaper vegetable oils such as soybean, rapeseed, sunflower, and others. The percentage of linoleic and oleic acid of samples 11 and 12 corresponds to that of sunflower oil. Samples 10 and 11 are probably a mixture of olive oil and sunflower oil. It is not adulterated with rapeseed or soybean oil because of the low content of linolenic acid and the percentage of oleic acid is higher than that of linoleic acid.
The practical absence of linolenic acid in all of the samples under study shows that they do not contain additions of rapeseed or soybean oil in which it is within the range of 4 to 11%. The fatty acid contents of the other investigated samples correspond to that of olive oils. The use of GC and HPLC methods for the detection of adulterations is not always justified for mass control of olive oils on the market because it is time-consuming and uses costly materials. The study of fluorescence spectra of olive oils with excitation in the 370- to 450-nm range making use of low cost lasers and LEDs that allow real-time measurements without the additional chemicals is a more attractive approach which is attempted in this article. Since the objective is a cheaper and a faster method, it should provide indications that with a sufficiently high probability a given olive oil might be adulterated and, based on that, the whole batch can be subjected to a more detailed and profound chemical analysis to determine the exact proportions of the adulterant. Correspondingly the spectral region below 350 nm is not considered in this study because LEDs and lasers in that range are considerably more expensive.
Sunflower oil exhibits fluorescence peaks in the 430 to 520 nm which is caused by the higher content of linoleic acid. Olive oils exhibit two clearly discernable fluorescence peaks–in the 275- to 297-nm and in the 600- to 690-nm ranges. The former is due to the presence of tocopherols and the latter to the chlorophyll groups. The interval from 540 to 670 nm does not contain information about specific groups that can allow the distinction of natural oil from olive oils. Since the lowest excitation wavelength is 405 nm (LD) the first fluorescence peak cannot be observed (275 to 297 nm) due to the tocopherols of the olive oils. Fluorescence spectra of the 12 olive oil samples excited with a 425-nm LED are shown in . Of all the excitation wavelengths, the 425-nm wavelength was chosen because the differences between fluorescence peaks of natural olive oils, sunflower oil, and those containing cheaper vegetable oils are best observed.
Figure 2 Fluorescence spectra obtained with 425 nm (LD) excitation: (a) Greek olive oil.; (b) Turkish and Portuguese olive oil; (c) adulterated olive oils and sunflower oil.
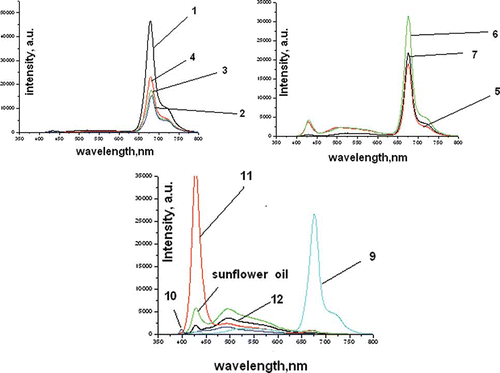
It is seen from the fluorescence peaks that:
• | Olive oils containing sunflower oil (samples 10, 11, and 12) exhibit a fluorescence peak correspondingly at 491.8, 428, and 495 nm with an intensity higher than 3000 arbitrary units. The latter is comparable to the one in sunflower oil, observed around 495.3 nm and featuring intensities around 5590 arbitrary units. | ||||
• | Olive oils exhibiting a high contents of chlorophyll (samples 1–4) show no peaks around 500 to 550 nm. These contain a fluorescence peak around 680 nm with intensities between 15,368 and 46,484 arbitrary units. | ||||
• | Samples 5 and 6 contain the fluorescence peak characteristics for olive oils around 680 nm, but also contain a second peak around 520 to 535 nm. This observation can be explained with the larger quantity of oxides obtained during storage. | ||||
• | Fluorescence peaks of sample 8 differ form those of natural oils, because it is pomace olive oil. It contains fragments of skin, pulp, and pieces of kernels. Refined olive-pomace oils are obtained from olive pomace after extraction with authorized solvents and a refining process, which includes neutralization, deodorization, and decolorization. All olive oil pomace showed a wide peak between 415 and 550 nm. This means that their content on conjugated hydroperoxides is higher as a result of a greater oxidation.[Citation14] It undergoes rapid deterioration due to the moisture content that speeds up triacylglycerol hydrolysis. | ||||
• | The 680 nm peak decreases in intensity with the increase of linoleic acid. |
It is clearly seen from the performed analysis that fluorescence spectra of samples 10, 11, and 12 strongly resemble those of sunflower oil. This fact is confirmed by the chemical analysis. Sample 9 shows a peak characteristic for olive oil but chemical analysis shows that the content of oleic acid is comparable to that of linolic acid. According to the parameters obtained from the chemical analysis, this sample is an admixture of olive oil and sunflower oil, but since chlorophyll content is high (caused by the fact that this is a mixture of natural olive oils and artificially added chlorophyll) a clearly discernable fluorescence peak resembling that of olive oil is observed. However, excitation at 450 nm causes a second fluorescence peak at 550 nm to appear.
The excitation-emission matrices for a part of the investigated types of olive oils are presented in . Using them, the natural olive oils, the adulterated, as well as pomace olive oils can clearly be separated. A detailed mathematical treatment will be reported elsewhere. It is clear that the 3D EEM of sample 6 strongly resembles those of samples 1 and 4 (the reference). This fact supports the conclusion of the natural character of this particular olive oil as confirmed by the fatty-acid content of the sample obtained from chemical analysis. The 3D matrices of the two of the adulterated samples strongly resemble that of the sunflower oil which is confirmed by their fatty-acid content. The EEM can also be used to identify pomage oil (whose chemical content is quite close to that of extra virgin) often sold for extra virgin oil.
In the present work, fluorescence spectroscopy is suggested as a method for the detection of adulterants in olive oils. For the region of Bulgaria these adulterants are lower cost vegetable oils. Studies have been performed for the validity of this method to discover admixtures of sunflower and rapeseed oils cultivated in Turkey. If the type of the pure olive oil is known the concentration of the adulterant can be determined from the fluorescence peaks. Based on these results, sunflower oil adulterant concentration in olive oil down to 10% can easily be determined from the intensity of the fluorescence spectra. Detection of lower concentrations has not been tested.
Since fluorescence spectra of vegetable oils are clearly different from those of olive oil, it can be assumed that they can be identified using fluorescence spectroscopy. Only walnut, flax, and almond oils exhibit fluorescence peaks around 650 to 670 nm that resemble the ones of olive oils. They, however have stronger peaks of varying widths in the 500- to 580-nm range. In the studied geographic regions olive oil is most frequently adulterated with corn, soy bean, and sunflower oil whose 3D excitation-emission matrices essentially differ from those of olive oil. Fluorescence spectroscopy can then be used to detect unauthorized adulteration. presents the 3D excitation-emission spectra of some of the most common adulterants.
Several tests of controlled adulteration of olive oil sample 7 with sunflower and corn oil have been performed. The results for sunflower oil adulteration are shown in . As is clearly seen, there is a clear transition from the pure olive oil spectrum to the pure sunflower oil spectrum and two effects are noticeable. The first is the increase in the fluorescence intensity peak F1 and its wavelength shift Δλ to lower wavelengths. The second is the reduction of the second fluorescence peak F2 caused by chlorophyll. Both are seen in . shows the wavelength shift Δλ versus the sunflower oil proportion. The dependence is linear and a 10% sunflower oil adulteration is easily detectable. Results for corn oil adulteration exhibit similar values.
Fluorescence can be used successfully to distinguish olive oil adulterated with sunflower oil that has lower content of chlorophyll. However, detection of rapeseed additions needs a chemical analysis of fatty acid and tocopherol contents. Apart from the fatty acid composition, the tocopherol, chlorophyll, and -carotene contents as well as the index of refraction have also been studied and the results are shown in .
Table 3 Fatty acid composition of oils
Table 4 Tocopherol, chlorophyll α,β-carotene content and index of refraction
It is evident from that samples 9, 10, 11, and 12 exhibit greater values for the index of refraction compared to the rest of the oils. Sunflower and olive oil contain basically α-tocopherols (above 90%), while soybean and rapeseed oils contain considerable quantities of γ- and δ-tocopherols. Samples 5 and 10 exhibit a higher content of γ-tocopherol (7.2 and 10.5%) which is within the acceptable range (: 89–100% ;
: 0–1%, and
: 0–11%). Sunflower oil contains mostly
-tocopherols–
: 93–98%,
: 1–2% , and
: 0–1%. Therefore, although sunflower oil has been added to sample 10 tocopherol content has not changed essentially.
This observation can be explained with the presence of larger quantity of polynonsaturated fatty acids in these samples as found from the chemical analysis. The percentage of -tocopherols in all samples is above 90% which means that no soy and rapeseed oils are present. Samples 10 and 11 are thus a mixture of olive and sunflower oil. These samples are a mixture of olive and sunflower oil and show an increase in the fluorescence peak at 520 nm. There are linear dependences between refractive index of the samples and content of the oleic and linoleic acids. It is evident from , that Greek olive oils (samples 1–4) have the highest content of chlorophyll. For them there exists a dependence between intensity of fluorescence peak at wavelength 680 nm and content of chlorophyll. The linear regressions and correlation coefficients are given in .
The first derivatives of the fluorescence obtained in samples excited with 425 nm LED have been calculated. and show the characteristic groups of pure olive oils and such in which cheaper vegetable oils have been added. By finding the first derivative of the fluorescence spectra it is easier to discern the three groups of commercially available olive oils. The first, shown in is the group of oils adulterated with sunflower oil sold as VOO, the second group is of pomace oils, also shown in the same figure, which has a poorer quality compared to VOO, but is not discernable chemically, and whose fluorescence spectra are closer to either sunflower oil a or a mixture of olive oil and sunflower oil; and the last third group is that of natural non-adulterated olive oils. If EEMs are used as those shown in , the olive oils will be subdivided into the same groups. To obtain a clearer picture of the samples under study, histograms of the positive and negative deviations from the fluorescence peaks of the 12 samples and the sunflower samples are shown in .
Table 5 Dependences between different parameters of olive oils
CONCLUSIONS
The results obtained allow for the following conclusions to be formulated: (1) Of all the excitation wavelengths the 425-nm LED appears as the most suitable for fluorescence analysis. (2) Comparison between fluorescence and chemical analysis shows that of all the 12 nominal olive oil samples, chemical analysis confirms 8 samples and rejects 4 samples as heavily adulterated with sunflower oil. Fluorescence analysis rejects the same samples as the chemical analysis, but confirms as olive oil only 5 samples, rejects the pomace oil and shows additional weak peaks characteristic for non-olive oils for 2 samples, which, however, may be due to oxidation during storage. (3) Combination of fluorescence analysis and refractive index measurements eliminates uncertainty about the above two samples and the pomace oil.
Thus a cheap fluorescence analysis with 425 nm excitation combined with a refractive index measurement can be developed provided there exists a database of reference fluorescence spectra.
ACKNOWLEDGMENTS
This work was inspired by the investigations made in frames of the project ISEKI-food, in which UFT, Plovdiv and Agricultural University, Plovdiv have participated since 2006. The partial financial support for this work from the NI 12 FF003 research grant of the Plovdiv University is acknowledged.
Notes
Color versions of one or more of the figures in the article can be found online at www.tandfonline.com/ljfp.
REFERENCES
- Stark , A.H. and Madar , Z. 2002 . Olive oil as a functional food: Epidemiology and nutritional approaches . Nutritional Reviews , 60 : 170 – 176 .
- Haward , J. and Aparicio , R. 2000 . Handbook of Olive Oil, Analysis and Properties , USA : Aspen Publishers: Maryland .
- Freihat , N. , Al-Shannag , A.K. and El Assi , N. 2008 . Qualitative responses of “Nabali” olive oil to harvesting time and altitudes at sub-humid Mediterranean . International Journal of Food Properties , 11 : 561 – 570 .
- Aparicio , R. and Aparicio-Ruiz , R. 2000 . Authentication of vegetable oils by chromatographic techniques . Journal of Chromatography A , 881 : 93 – 104 .
- Tsimidou , M. and Macrae , R. 1987 . Authentication of virgin olive oils . Food Chemistry , 25 : 251 – 258 .
- Sayage , A. , Morales , M.T and Aparicio , R. 2004 . Detection of hazelnut oil in virgin olive oil by a spectrofluorimetric method . European Food Research Technology , 218 : 480 – 485 .
- Dupuy , N. , Dreau , Y. , Olliver , D. , Artaud , J. , Pinatel , C.H. and Kister , J. 2005 . Origin of French virgin olive oil registered designation of origins predicted by chemometric analysis of synchronous excitation–emission fluorescence spectra . Journal of Agricultural and Food Chemistry , 53 : 9361 – 9368 .
- Guimet , F.R. , Ferre , J. and Boque , R. 2005 . Rapid detection of olive-pomace oil adulteration in extra virgin olive oils from the protected denomination of origin “Siurana” using excitation-emission fluorescence spectroscopy and three-way methods of analysis . Analytica Chimica Acta , 544 : 143 – 152 .
- Cecchi , T. , Passamonti , P. , Alfei , B. and Cecchi , P. 2011 . Monovarietal extra virgin olive oils from the marche region, italy: analytical and sensory characterization . International Journal of Food Properties , 14 : 483 – 495 .
- 2006 . Animal and vegetable fat and oils. Determination of tocopheros and tocotriends contents. Method using high performance liquid chromotography , ISO 9936 . p. 17.
- Christie , W.W. 2003 . Lipid Analysis , Bridgewater , England : The Oily Press .
- 2004 . Animal and vegetable fat and oils. Analysis by gas chromotography of methyl esters of fatty acids , 9 ISO 5508 .
- Codey Stan 210. Recommended standards for vegetable oils and oils products by the Codex Alimentarius Commission Revision 2001, 2003, 2009. Amendment 2005, 2011, p. 16.
- Boggia , R. , Zunin , P. , Lanteri , S. , Rossi , N. and Evangelisti , F. 2002 . Classification and class-modelling of “Riviera Ligure” extra-virgin olive oil using physico chemical parameters . Journal of Agricultural and Food Chemistry , 50 : 2444 – 2449 .