Abstract
Reflectance Visible/near-infrared spectroscopy was used to analyze the effect of peel on the non-destructive soluble solids content prediction of oranges. The spectra were interpreted and partial least squares model was developed for both intact and peeled oranges at two wavelength ranges of 450–1000 and 700–1000 nm. Results showed that the wavelengths of the main absorbance peaks on the spectra of the intact and peeled samples were the same (p > 0.05). Moreover, the developed models yielded a good prediction accuracy of soluble solids content (2.5 < standard deviation ratio < 3) for intact oranges as well as the peeled fruits at the spectral ranges of 450–1000 and 700–1000 nm (p > 0.05). It was also noted that the effect of peel chemical composition can be ignored in non-destructive spectroscopy of intact citrus for determination of soluble solids content.
INTRODUCTION
Quality of fruits and vegetables is very important to the consumer as well as the producer. In recent years, many destructive and non-destructive methods have been developed for determining the quality of agricultural products. Non-destructive methods have been widely noticed because they are fast, require less sample preparation, are easy to use in process control and grading systems, and do not disturb the product. Near-infrared (NIR) spectroscopy in reflectance or transmittance modes is one of the applicable non-destructive methods that has been widely used for determination of some inner attributes of fruits and vegetables such as apple,[Citation1−Citation4] apricot,[Citation5,Citation6] bell pepper,[Citation7] mandarin,[Citation8−Citation10] mango,[Citation11,Citation12] orange,[Citation13−Citation17] peach,[Citation18] pear,[Citation19−Citation21] strawberry,[Citation22] and tomato.[Citation23−Citation25] The peel, or skin, is part of the light path in both reflectance and transmittance spectroscopy modes which can affect the prediction results especially for fruits with thick inedible peels such as citrus. Transmittance is not a suitable mode for assessing large produce. Moreover, the amount of light penetrating the fruit is often very small, thus making it difficult to obtain accurate transmission measurements at grading line speeds.[Citation13] Reflectance mode provides some information about both appearance and internal attributes of the sample. This is the easiest mode to obtain measurements, because they require no contact with the fruit and light levels are relatively high.[Citation14] While good results have been obtained in the fruits with a thin peel using reflectance NIR spectroscopy, it is very difficult to determine the composition in a thick fruit such as citrus using this method. Moreover, the information of the appearance attributes may affect the prediction models of fruit internal composition when the wavelength ranges extend to visible region. Therefore, it is useful to assess the peel effect on the internal quality prediction of thick-skinned fruits, such as citrus, using visible/NIR (Vis/NIR) spectroscopy which has been quite popular with researchers.
In this research, reflectance Vis/NIR spectra of orange fruit (both intact and peeled) were analyzed and interpreted in terms of the main absorbance peaks. Moreover, the robustness of Vis/NIR spectroscopy was evaluated for prediction of soluble solids content (SSC), the most important quality attribute of orange fruit, for both intact and peeled oranges at two spectral ranges of 450–1000 and 700–1000 nm using partial least squares (PLS) to assess the effect of the peel and visible region on non-destructive prediction models. Regression coefficients of the models were also investigated to determine the importance of the wavelengths for modelling.
MATERIAL AND METHODS
Fruit Samples
A total of 100 Valencia oranges used for the experiments were purchased from different local markets in different days (10 samples each time). Seventy samples were used for calibration and the residual 30 samples were separated for external validation. The fruits were stored at 25°C for 1 day before Vis/NIR measurements to reach equilibrium temperature with the laboratory environment. After Vis/NIR measurements of intact fruits, tests were conducted on peeled oranges. Then, reference measurements were carried out on each sample. The average of peel thickness of the samples measured with vernier caliper was 3.45 mm.
Spectral Acquisition and Preprocessing
The spectroscopy equipment consisted of a CCD spectrometer (USB2000, Oceanoptics Inc., USA), tungsten halogen lamp (12V-50W), an optical fiber with 0.4 mm diameter and a fruit holder. The wavelength range of the spectrometer was 350–1050 nm. However, the first 100 and the last 50 wavelength values were omitted because of the existence of considerable noise in these regions. The optical fiber was placed vertically at a height of 100 mm from the holder and the light source was set up 200 mm above the holder center at an angle of 45°.
Before acquisition of spectra and after every ten samples, a white reference was used to obtain the relative reflectance. For each sample, reflectance spectra at three positions around equatorial locations (approximately 120°) and one scan at each position were collected by OOIBase32 software (Oceanoptics Inc., USA). Next, the mean spectrum was calculated from a total of three scans for each fruit. Then, all the samples were peeled and spectroscopy tests were conducted for the whole fruit flesh. Vis/NIR spectra are sensitive to the concentrations of organics materials which involved the response of molecular bonds C-H, O-H, and N-H (bonds with hydrogen atoms tend to vibrate independently from the rest part).[Citation16,Citation26] Therefore, overall reflectance spectra of each fruit (both intact and peeled) were converted to log (1/R) to obtain linear correlation between the spectrum and the sample molecular concentration. Then, the spectra were compared in terms of the wavelength of the main absorbance peaks relevant to O-H, C-H, or N-H functional groups present in soluble solids (mainly sugars and smaller amounts of organic acids, amino acids, etc.), water and pigments (chlorophyll and carotenoids). The wavelength of each main peak was detected by the computer for ten samples which were selected from the sampled lot, randomly. The paired t-test was used to investigate the difference of means between the wavelength of each main peak for intact and peeled oranges. Before developing the calibration models, the spectra of all samples were pre-processed using first derivative to increase spectral resolution. To correct both multiplicative and additive effects of the spectra due to scattering, particle size, and the change of light distance, the multiplicative scatter correction (MSC) was also used.[Citation27,Citation28] The OriginPro 8 (OriginLab Corporation, USA) and the Unscrambler v9.7 (CAMO Software AS, Norway) softwares were used for data analysis.
SSC Measurement
After spectroscopy measurements of the peeled samples, SSC was determined destructively. The juice of each sample was extracted and SSC was measured using a digital refractometer DR-A1 (Atago Co. Ltd., Japan). shows the statistics of the data sets.
Table 1 Statistics of the data sets for SSC
PLS Analysis
Calibration models were developed using PLS regression with cross validation based on 20 segments on the spectra at both 450–1000 and 700–1000 nm. The optimal number of latent variables (LVs) was determined by selection of the minimum on the root-mean-squared error of cross validation (RMSECV) versus LVs plot. Moreover, comparison of the calibration models was done based on RMSECV, correlation coefficient of cross validation (rcv), and the SDR which is defined as the ratio of the standard deviation of the response variable to the RMSECV.[Citation28] Finally, the external validation set was used to evaluate the models based on the root-mean-squared error of prediction (RMSEP), correlation coefficient of prediction (rp), and the systematic deviation (bias). The two-way ANOVA was also applied to assess the difference between the biases of the prediction models. Moreover, the obtained regression coefficients in the optimal number of LVs using the PLS models for SSC prediction of both intact and peeled oranges were investigated at two spectral ranges of 450–1000 and 700–1000 nm. The OriginPro 8 (OriginLab Corporation, USA) and the Unscrambler v9.7 (CAMO Software AS, Norway) softwares were used for the analysis.
RESULTS AND DISCUSSION
Vis/NIR Spectra Interpretation
shows the typical absorbance spectra of intact and peeled samples. In the visible region up to 700 nm, the curves had decreasing trends and there was an absorbance peak around 670 nm in both intact and peeled orange spectra because of chlorophyll absorbance.[Citation9,Citation13,Citation15] This was more perceptible for the intact fruit than the peeled orange. The difference between the spectra in this region was due to absorbance of different pigments and color of the peel. A broad peak around 740 nm was also found on the spectra which could be due to the third overtone of O-H, the forth overtones of C-H, CH2, and CH3.[Citation27] In the NIR region, a broad peak was found around 840 nm which may be attributed to the third overtones of C-H, CH2, and CH3.[Citation27] There was also a perceptible peak around 970 nm due to the second overtone of O-H and the second overtone of NH2 in both intact and peeled fruits.[Citation27] In the visible region, absorbance for the peeled sample was less compared to intact orange because of peel elimination and more reflection relevant to flesh pigments. In contrast, the absorbance for the peeled fruit in the NIR region (around 850–1000 nm) was more against that for the intact sample which could be due to higher light penetration into the flesh and more vibrated functional groups in the peeled fruit. and show the mean wavelength of the main absorbance peaks on the spectra of ten samples and paired t-test results between the wavelengths of each main peak of intact and peeled fruits, respectively. Consequently, the region of the main peaks due to stretching vibration of the overtones of O-H, C-H, or N-H functional groups for peeled orange was similar to that for the intact sample. Moreover, the wavelength means of the main absorbance peaks between intact and peeled orange were not significantly different (p > 0.05).
Table 2 Mean wavelength of the main absorbance peaks on the spectra of ten samples
Table 3 Paired t-test results comparing the wavelength of the main peaks of intact and peeled samples
Data Analysis
The results of PLS models for SSC prediction of both intact and peeled oranges are summarized in . Results indicated that calibration models had the potential to estimate SSC for intact oranges as well as peeled fruits. Due to peel removal, the calibration models for the peeled fruits (RMSECV = 0.311, rcv = 0.941, SDR = 2.965 at 450–1000 nm, and RMSECV = 0.308, rcv = 0.942, SDR = 2.994 at 700–1000 nm) were more accurate than those for the intact samples (RMSECV = 0.330, rcv = 0.933, SDR = 2.794 at 450–1000 nm and RMSECV = 0.319, rcv = 0.938, SDR = 2.890 at 700–1000 nm). Moreover, the models at 700–1000 nm could yield slightly better prediction of SSC against those at 450–1000 nm for both intact and peeled fruits. The reason is that in the visible region from 450–700 nm, there is no absorbance peak relevant to the overtones of O-H or C-H functional groups which can include the information about SSC. The SDR value of all calibration models were close to three which corresponds to good prediction accuracy.
Table 4 Statistics of PLS models for SSC prediction of both intact and peeled oranges at two wavelength ranges of 450–1000 nm and 700–1000 nm
The scatter plots of correlation between the measured and predicted values of SSC for the external data set at two wavelength ranges of 450–1000 and 700–1000 nm for both intact and peeled oranges are shown in . Results indicated that the prediction models for the peeled fruits (RMSEP = 0.291, rp = 0.951 at 450–1000 nm and RMSEP = 0.289, rp = 0.951 at 700–1000 nm) were better than those for the intact samples (RMSEP = 0.321, rp = 0.937 at 450–1000 nm and RMSEP = 0.309, rp = 0.944 at 700–1000 nm). The prediction results in this research for intact oranges at 450–1000 nm were in agreement with those achieved in reflectance mode for Satsuma mandarin in 400–2350 nm region (RMSEP = 0.33, rp = 0.94);[Citation9] and better than those reported for Satsuma mandarin in 500–1100 nm region (RMSEP = 0.63, R2 = 0.75);[Citation10] for Valencia orange in 570–1850 nm region (RMSEP = 0.51, rcv = 0.85);[Citation13] for oranges in 500–2300 nm region (RMSEP = 0.74, rcv = 0.91, SDR = 2.13);[Citation14] for navel orange in 350–1800 nm region using principle component analysis-back propagation (PCA-BPNN) model (RMSEP = 0.68, rp = 0.90);[Citation16] as well as for Gannan navel orange in 450–1750 nm region using Spline-PLS model (RMSEP = 0.47, rp = 0.87, SDR = 2.34).[Citation17] However, a comparison is not recommended due to the variety of spectrometers and spectral ranges used in the above research reports.
Figure 2 Scatter plots of the correlation between measured and predicted values of SSC for the external data set at wavelength range of 450–1000 nm for intact (a) and peeled (b) oranges, as well as at wavelength range of 700–1000 nm for intact (c) and peeled (d) oranges.
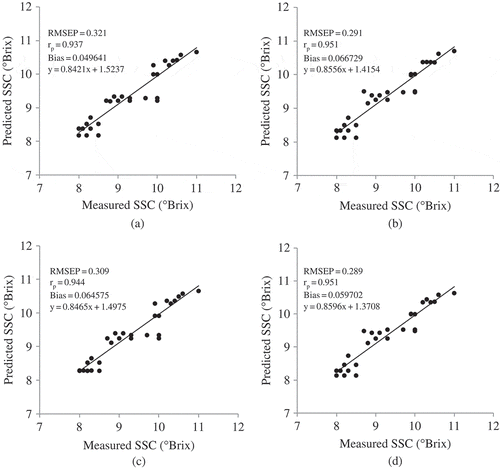
The obtained regression coefficients in the optimal number of LVs using the PLS models for SSC prediction of both intact and peeled oranges at two spectral ranges of 450–1000 and 700–1000 nm are also shown in . The importance of the wavelengths of NIR region for modeling could be seen in all regression coefficient plots which reveal good correlation between SSC and the spectral information in this region. In the 450–1000 nm range for both intact and peeled fruits the wavelength range of 450–550 nm were important especially for intact samples. However, the wavelengths around 670 nm did not play the main role to develop the model for peeled oranges because of the existence of less chlorophyll compared to that for intact fruits (). Therefore, at the spectral range of 450–1000 nm, the models rely on the spectral information due to the absorbance of both functional groups and pigments. For this reason, the accuracy of the models in this range is less than that in the 700–1000 nm for SSC prediction. However, two-way ANOVA results revealed that the biases of the prediction models were not significantly different at the 0.05 level for both the state of the sample (intact and peeled) and the spectral range (450–1000 and 700–1000 nm) factors ().
Table 5 ANOVA results between the bias squares of the prediction models for intact and peeled oranges at the wavelength of 450-1000 nm and 700-1000 nm
Figure 3 The obtained regression coefficients in the optimal number of LVs using the PLS models preprocessed with first derivative + MSC for SSC prediction at wavelength range of 450–1000 nm for intact (a) and peeled (b) oranges, as well as at wavelength range of 700–1000 nm for intact (c) and peeled (d) oranges.
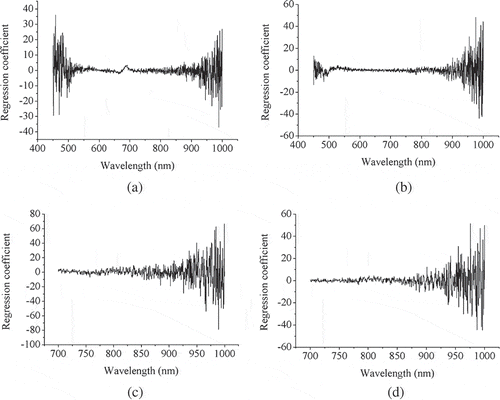
CONCLUSIONS
In this research, Vis/NIR spectroscopy technique was used to analyze the peel effect on SSC prediction of intact oranges. To this end, the reflectance spectra of orange fruit (both intact and peeled fruits) were analyzed and interpreted in terms of the main absorbance peaks. Then, PLS models using first derivative and MSC preprocessing methods were developed for SSC prediction of both intact and peeled oranges at two spectral ranges of 450–1000 and 700–1000 nm. The regression coefficients of the models were also investigated. The following conclusions can be drawn from the results of data analysis: (1) the region of the main absorbance peaks on the spectra of the intact oranges was similar to that on the spectra of peeled samples. Moreover, the wavelengths of these peaks were not significantly different at the 0.05 level. (2) Reflectance Vis/NIR spectroscopy yielded a good prediction accuracy of SSC (2.5 < SDR < 3) for intact oranges as well as peeled samples at the spectral ranges of 450–1000 and 700–1000 nm. On the other hand, the prediction models were not significantly different at the 0.05 level. (3) The peel effect on SSC prediction of intact oranges can be ignored in Vis/NIR spectroscopy. (4) The whole visible region combined with short wave NIR region can be useful for SSC prediction of oranges when some appearance attributes such as color is assessing simultaneously.
REFERENCES
- McGlone, V.A.; Jordan, R.B.; Martinsen, P.J. Vis/NIR estimation at harvest of pre- and post-storage quality indices for “Royal Gala” apple. Postharvest Biology and Technology 2002, 25 (2), 135–144.
- Peirs, A.; Tirry, J.; Verlinden, B.; Darius, P.; Nicolaï, B.M. Effect of biological variability on the robustness of NIR models for soluble solids content of apples. Postharvest Biology and Technology 2003, 28, 269–280.
- Qing, Z.; Ji, B.; Zude, M. Wavelength selection for predicting physicochemical properties of apple fruit based on near-infrared spectroscopy. Journal of Food Quality 2007, 30, 511–526.
- Jha, S.N.; Garg, R. Non-destructive prediction of quality of intact apple using near infrared spectroscopy. Journal of Food Science and Technology 2010, 47 (2), 207–213.
- Bureau, S.; Ruiz, D.; Reich, M.; Gouble, B.; Bertrand, D.; Audergon, J.M.; Renard, C.M.G.C. Rapid and non-destructive analysis of apricot fruit quality using FT-near-infrared spectroscopy. Food Chemistry 2009, 113, 1323–1328.
- Camps, C.; Christen, D. Non-destructive assessment of apricot fruit quality by portable visible-near infrared spectroscopy. LWT-Food Science and Technology 2009, 42, 1125–1131.
- Penchaiya, P.; Bobelyn, E.; Verlinden, B.E.; Nicolaï, B.M.; Saeys, W. Non-destructive measurement of firmness and soluble solids content in bell pepper using NIR spectroscopy. Journal of Food Engineering 2009, 94, 267–273.
- Antonucci, F.; Pallottino, F.; Paglia, G.; Palma, A.; D’Aquino, S.; Menesatti, P. Non-destructive estimation of mandarin maturity status through portable Vis-NIR spectrophotometer. Food Bioprocess and Technology 2011, 4 (5), 809–813.
- Gómez, A.H.; He, Y.; Pereira, A.G. Non-destructive measurement of acidity, soluble solids and firmness of satsuma mandarin using Vis/NIR-spectroscopy techniques. Journal of Food Engineering 2006, 77, 313–319.
- McGlone, V.A.; Fraser, D.G.; Jordan, R.B.; Künnemeyer, R. Internal quality assessment of mandarin fruit by Vis/NIR spectroscopy. Journal of Near Infrared Spectroscopy 2003, 11, 323–332.
- Saranwong, S.; Sornsrivichai, J.; Kawano, S. Prediction of ripe-stage eating quality of mango fruit from its harvest quality measured nondestructively by near infrared spectroscopy. Postharvest Biology and Technology 2004, 31, 137–145.
- Subedi, P.P.; Walsh, K.B.; Owens, G. Prediction of mango eating quality at harvest using short-wave near infrared spectroscopy. Postharvest Biology and Technology 2007, 43, 326–334.
- Cayuela, J.A. Vis/NIR soluble solids prediction in intact oranges (Citrus Sinensis L.) cv. Valencia late by reflectance. Postharvest Biology and Technology 2008, 47, 75–80.
- Cayuela, J.A.; Weiland, C. Intact orange quality prediction with two portable NIR spectrometers. Postharvest Biology and Technology 2010, 58, 113–120.
- Jamshidi, B.; Minaei, S.; Mohajerani, E.; Ghassemian, H. Reflectance Vis/NIR spectroscopy for nondestructive taste characterization of Valencia oranges. Computers and Electronics in Agriculture 2012, 85, 64–69.
- Liu, Y.; Sun, X.; Ouyang, A. Nondestructive measurement of soluble solids content of navel orange fruit by Visible-NIR spectrometric technique with PLSR and PCA-BPNN. LWT-Food Science and Technology 2010a, 43 (4), 602–607.
- Liu, Y.; Sun, X.; Zhou, J.; Zhang, H.; Yang, C. Linear and nonlinear multivariate regressions for determination sugar content of intact Gannan navel orange by Vis-NIR diffuse reflectance spectroscopy. Mathematical and Computer Modelling 2010b, 51 (11–12), 1438–1443.
- Golic, M.; Walsh, K.B. Robustness of calibration models based on near infrared spectroscopy for the in-line grading of stone fruit for total soluble solids content. Analytica Chimica Acta 2006, 555, 286–291.
- Liu, Y.; Chen, X.; Ouyang, A. Nondestructive determination of pear internal quality indices by visible and near-infrared spectroscopy. LWT-Food Science and Technology 2008, 41, 1720–1725.
- Liu, Y.; Ying, Y. Noninvasive method for internal quality evaluation of pear fruit using fiber-optic FT-NIR spectrometry. International Journal of Food Properties 2007, 10 (4), 877–886.
- Sirisomboon, P.; Tanaka, M.; Fujita, S.; Kojima, T. Evaluation of pectin constituents of Japanese pear by near infrared spectroscopy. Journal of Food Engineering 2007, 78, 701–707.
- Shao, Y.; He, Y. Nondestructive measurement of acidity of strawberry using Vis/NIR spectroscopy. International Journal of Food Properties 2008, 11 (1), 102–111.
- Flores, K.; Sánchez, M.; Pérez-Marín, D.; Guerrero, J.; Garrido-Vro, A. Feasibility in NIRS instruments for predicting internal quality in intact tomato. Journal of Food Engineering 2009, 91, 311–318.
- Pedro, A.M.K.; Ferreira, M.M.C. Simultaneously calibrating solids, sugars, and acidity of tomato products using PLS2 and NIR spectroscopy. Analytica Chimica Acta 2007, 595, 221–227.
- Shao, Y.; He, Y.; Gómez, A.H.; Pereir, A.G.; Qiu, Z.; Zhang, Y. Visible/near infrared spectrometric technique for nondestructive assessment of tomato “Heatwave” (Lycopersicum Esculentum) quality characteristics. Journal of Food Engineering 2007, 81, 672–678.
- Lin, H.; Ying, Y. Theory and application of near infrared spectroscopy in assessment of fruit quality: A review. Sensing and Instrumentation for Food Quality and Safety 2009, 3 (2), 130–141.
- Cen, H.; He, Y. Theory and application of near infrared reflectance spectroscopy in determination of food quality. Trends in Food Science and Technology 2007, 18, 72–83.
- Nicolaï, B.M.; Beullens, K.; Bobelyn, E.; Peirs, A.; Saeys, W.; Theron, K.I.; Lammertyn, J. Nondestructive measurement of fruit and vegetable quality by means of NIR spectroscopy: A review. Postharvest Biology and Technology 2007, 46, 99–118.