Abstract
The peanut proteins were extracted from cold pressed and hot pressed peanut meal in this work. Emulsifying properties of cross-linking between cold pressed peanut/hot pressed peanut and fish (Decapterus maruadsi) protein hydrolysates catalyzed by transglutaminase were investigated. Electrophoresis indicated that cross-linked proteins were formed in all the transglutaminase-treated samples, and the susceptibility of cold pressed peanut or hot pressed peanut subunits to transglutaminase was enhanced by addition of the (Decapterus maruadsi) protein hydrolysates. For the cross-linked cold pressed peanut-(Decapterus maruadsi) protein hydrolysates and hot pressed peanut-(Decapterus maruadsi) protein hydrolysates protein conjugates, the surface area mean diameter d32, volume mean diameter d43, creaming index, and instability decreased after TGase treatment. Besides, the addition of (Decapterus maruadsi) protein hydrolysates could remedy the disadvantage of essential amino acids (e.g., Thr, Met, and Lys) deficiency in cold pressed or hot pressed peanut. For cold pressed peanut or hot pressed peanut, transglutaminase treatment had no effect on the emulsifying activity but the emulsion stability was improved.
INTRODUCTION
Peanuts (Arachis hypogaea L.) are a very important oilseed crops on a global basis. The total peanut production in China is the highest in the world and was estimated to be approximately 15.7 × 106 t in 2010.[Citation1] Generally, most peanuts grown in China are used for extraction of edible oil, using a cold pressing or hot pressing technique. During this process, the protein-rich residues are generated. So far, these defatted peanut meals have not been fully utilized yet, since they have poor functional properties and low nutritional value due to the deficiency of some essential amino acids including lysine, tryptophan, and threonine.[Citation2] Therefore, improving the functional properties and enhancing the nutritional properties of peanut proteins from the defatted meal would be crucial for the use of this protein resource in the food industry.
Round scads (Decapterus maruadsi) are prevalent pelagic fish that make up 40–50% of the world’s total fish catch.[Citation3] This dark-fleshed fish has limited uses due to their dark color, susceptibility to oxidation, and off-flavor.[Citation4] Therefore, they are discarded or processed into low market-value products, such as fish meal and fertilizer. However, recent advances in biotechnology have demonstrated the capacity of enzymes to produce value-added products with high nutritive value and high protein digestibility.[Citation5] Thiansilakul et al.[Citation4] reported that Decapterus maruadsi hydrolysates could be a good dietary protein supplement for poorly balanced dietary proteins. Thus, the nutritional properties of Decapterus maruadsi protein hydrolysates might improve the nutritional value of peanut protein.
Transglutaminase (TGase; EC 2.3.2.13), a popular enzyme in food industries, could catalyze acyl-transfer reactions between the γ-carboxyamide of peptides or protein-bound glutamine residues (acyl donors) and various primary amines (acyl acceptors). When the epsilon-amino groups of lysine residues act as acyl acceptors, ϵ-(γ-glutamyl) lysine isopeptide bonds are formed, resulting in both intra- and intermolecular cross-linking.[Citation6] TGase can be used to modify the functional properties of many food proteins.[Citation7−Citation12] Besides, TGase can also improve the functional properties of food protein hydrolysates.[Citation13−Citation16]
Due to the high lysine content of Decapterus maruadsi fish,[Citation4] it is possible that TGase-induced cross-linking between peanut proteins and fish protein hydrolysates results in improved functional properties, which might be applied in the food system containing heterologous protein-peptides. Therefore, this work aimed to create a conjugate between cold or hot pressed peanut proteins with Decapterus maruadsi protein hydrolysates under the catalysis of TGase and to investigate the emulsifying properties of the cross-linked products.
MATERIALS AND METHODS
Materials
Defatted peanut meals following oil extraction by cold and hot pressing treatments (mainly including pulverization, with or without steam cooking, and mechanical pressing) were obtained from Tianshen Bioprotein Co., Ltd. (Linyi, China) and Luhua Group Co., Ltd (Yantai, China), respectively. The protein content of cold pressed meal (55.1%, w/w) was higher than that of hot pressed peanut (HPPP) meal (42.9%, w/w). Decapterus maruadsi was obtained from a local market in Guangzhou, China. The fish (without heads, tail, scales, internal organs, and blood) were filleted and minced in a MM12 mincer (Shaoguan Food Machine Co., Shaoguan, China). The minced Decapterus maruadsi (containing 18.9% protein, w/w) was frozen and stored at –20°C for further use within two weeks. Food-grade papain (7 × 105 U/g) was purchased from Baiao Biochemistry Co. (Jiangmen, China). Transglutaminase (TGase, 1000 U/g) was donated by Yiming Biological Products Co., Ltd. (Taixing, China). Corn oil was purchased from Yihai (Guangzhou) Oils and Fats Industrial Co., Ltd. (Guangzhou, China). All the other chemicals used in the present study were at least of analytical grade.
Preparation of Peanut Proteins
Peanut proteins, which were extracted from cold (CPPP) or HPPP, were obtained by dispersing peanut meal in deionized water (1:10 w/v), and the dispersion was adjusted to pH 8.0 with 1 M NaOH. The resultant dispersion was stirred at room temperature for 2 h, then centrifuged in a CR22G high-speed centrifuge (Hitachi Co., Tokyo, Japan) at 8000 g at 20°C for 30 min to remove the insoluble material. The supernatant was collected and adjusted to pH 4.5 with 1 M HCl and then centrifuged at 8000 g at 20°C for 10 min. The precipitate obtained was redispersed in deionized water. The dispersion was adjusted to pH 7.0 with 1 M NaOH, and dialyzed for 24 h at 4°C and then followed by freeze-drying using the Alpha 1-4 LD plus freeze dryer (Marin Christ, Osterode, Germany) to obtain CPPP and HPPP. The protein recoveries of CPPP and HPPP were 24.4 and 43.2%, respectively.
Preparation of Decapterus Maruadsi Protein Hydrolysates
The Decapterus maruadsi protein hydrolysates (DPH) were obtained by partial hydrolysis of Decapterus maruadsi proteins with papain according to the method of Ren et al.[Citation17] with some modifications. The minced fish protein was mixed with deionized water in a ratio of 1:2 (w/v). The dispersion was adjusted to pH 7.0 with 0.01 M NaOH, and then papain was added at an enzyme to substrate ratio of 1.5:1000 (w/w). The mixture was incubated at 55°C in water bath for 10 min and then heated at 95°C for 10 min to inactivate the enzyme. Degree of hydrolysis (DH), which is defined as the percentage of peptide bonds cleaved during the hydrolysis, was determined by the trinitrobenzenesulfonic acid method of Adler-Nissen.[Citation18] The hydrolysates were centrifuged at 4000 g for 20 min at 4°C and the supernatants (DH = 4.8%) were lyophilized and preserved in desiccator for further use.
TGase Treatment
The samples were cross-linked by TGase according to Tang et al.[Citation19] with slight modifications. Unless mentioned otherwise, the protein content was determined by the Kjeldahl method (N × 6.25). Twenty mg/mL of CPPP, HPPP, and DPH solutions (pH 7.0) were prepared. Then the DPH solution was blended with CPPP and HPPP at a ratio of 1:1 (v/v), respectively. All the solutions were pre-incubated at 37°C in water bath. Then TGase was added at an enzyme to protein substrate ratio of 0.5:100 (w/w) and incubated at 37°C for 90 min. At the end of the reaction, TGase was inactivated by heating at 80°C for 1 min in a water bath and the reaction products were lyophilized and preserved in desiccator for further use.
Emulsion Preparation
The protein solutions (20 mg/mL) were prepared and the pH was adjusted to 7.0 with 1 M NaOH or HCl. Corn oil was then added to the protein solution and mixed to form a final mixture containing 20% (w/w) corn oil that was homogenized using the APV-1000 homogenizer (APV Gaulin, Abvertslund, Denmark) operating at 30 MPa. Each emulsion sample was prepared in duplicate.
Sodium Dodecylsulphate–Polyacrylamide Gel Electrophoresis (SDS–PAGE)
SDS-PAGE of samples with and without TGase treatment was performed according to the method of Laemmli[Citation20] with slight modifications. A 12% acrylamide separating gel and a 5% acrylamide stacking gel containing 0.1% SDS were used in the DYCZ-30 electrophoresis apparatus (Liuyi Instrument Factory, Beijing, China). The samples were dissolved in deionized water to 2 mg/mL and mixed with 0.06 M Tris-HCl buffer (at a ratio of 1:5, v/v) containing 2% SDS, 5% 2-mercaptoethanol, 25% glycerol, and 0.1% bromophenol blue. The solutions were then heated for 5 min in boiling water and centrifuged at 10,000 g for 3 min before electrophoresis. Fifteen microliters of each sample were loaded onto the gel. A cocktail protein standards containing rabbit phosphorylase b (97.4 kDa), bovine serum albumin (66.2 kDa), rabbit actin (43.0 kDa), bovine carbonic anhydrase (31.0 kDa), and trypsin inhibitor (20.1 kDa) was also used for molecular weight markers. The electrophoresis gel sheets were stained for proteins with 0.25% Coomassie Brilliant Blue-R250 in 50% trichloroacetic acid and then destained with the solution containing 10% acetic acid and 10% methanol.
Amino Acid Analysis
The amino acid profiles of samples were determined according to the method of You et al.[Citation21] The amino acid composition was determined by high-performance liquid chromatography (Waters, Milford, MA, USA) equipped with a 300 × 3.9 mm i.d. reversed-phase column (Novapack C18, 4 μm; Waters). The total amino acids (except for tryptophan) were determined after hydrolysis at 110°C for 24 h with 6 M HCl prior to the derivatisation with phenyl isothiocyanate. The amino acid standards containing 17 amino acids (Asp, Glu, Ser, Gly, His, Arg, Thr, Ala, Pro, Tyr, Val, Met, Cys, Ile, Leu, Phe, and Lys) (Sigma–Aldrich, St. Louis, MO, USA) were analyzed using the same conditions as for the samples.
Determination of Particle Size
Particle size of emulsions was determined according to the method of Luo et al.[Citation22] Particle size distribution of the emulsions was measured using a Mastersizer 2000 (Malvern Instruments Ltd, Worcestershire, UK). Measurements were carried out at room temperature and the volume fraction of the emulsion in the diluted deionized water was approximately 1:1000. The mean particle size was characterized in terms of the surface area mean diameter d32 and volume mean diameter
where ni is the number of particles with diameter di). The d32 value was used to estimate the specific surface area of freshly made emulsion, and the d43 value was used to monitor changes in droplet size. Each sample was measured in triplicate.
Microstructure of Emulsions
The images of the emulsions were observed immediately after emulsion preparation using the Olympus CX31-12C04 microscope (Olympus Co., Tokyo, Japan). A drop of the non-diluted emulsion sample was placed on a microscope slide and covered with a cover slip, then visualized at a magnification of 400×. The images were captured by a camera connected to the microscope and the photographs of the emulsions were recorded for analysis.
Emulsion Stability Measurements
Emulsion stability was determined according to the method of Chen et al.[Citation23] with slight modifications. Emulsion stability was evaluated by determining the particle size d43 values and creaming index of emulsions during the storage time. The emulsion samples were stored at room temperature for 20 days and the particle size d43 was measured using the Mastersizer 2000. The stability of emulsions against creaming was also measured. Each emulsion sample was poured into a 10 mL glass tube (height 135 mm, diameter 15 mm), and then the height of the separated cream of the emulsion layer in each tube was recorded after 20 days of storage at room temperature. The creaming index of the emulsions was determined and defined as a ratio of the height of cream layer to the total height of emulsion. Prior to analysis, 0.02% sodium azide (w/v) was added to all the samples to avoid microbial growth.
Statistical Analysis
The data of the experiments were subjected to one-way analysis of variance (ANOVA) using the SPSS Version 13.0 for Windows (SPSS Inc., Chicago, IL, USA). The significant differences between means (p < 0.05) were identified by the least significant difference (LSD) test.
RESULTS AND DISCUSSION
SDS-PAGE Analysis
SDS-PAGE was performed to determine the effects of TGase treatment on CPPP, HPPP and their cross-linked products with DPH, respectively (). The band intensity of the four major subunits of arachin (approximately 42.0, 37.0, 35.0, and 22.0 kDa) showed no obvious difference between CPPP and HPPP (see lanes 1 and 5), but the band intensity of one major subunit of conarachin (approximately 64.0 kDa) in HPPP was much weaker than that in CPPP suggesting a loss induced by hot pressing. Chiou[Citation24] also found that conarachin was less thermal resistant than arachin since conarachin contains higher amounts of lysine and cysteine which have active functional R-groups.
Figure 1 SDS-PAGE profile of samples without (lanes 1, 2, 5, 6) and with (lanes 3, 4, 7, 8) TGase treatment. Lane M: Molecular markers; Lane 1: CPPP; Lane 2: mMixture of CPPP and DPH; Lane 3: cCross-linked CPPP; Lane 4: cCross-linked CPPP and DPH; Lane 5: HPPP; Lane 6: mMixture of HPPP and DPH; Lane 7: cCross-linked HPPP; Lane 8: cCross-linked HPPP and DPH (CPPP: cold-pressed peanut protein; HPPP: hot-pressed peanut protein; DPH: Decapterus maruadsi hydrolysates).
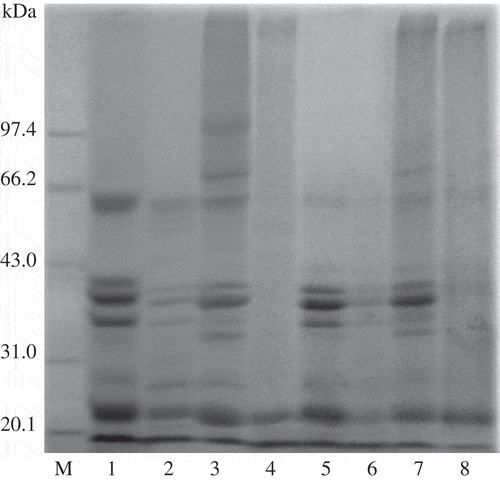
For TGase-treated CPPP and HPPP (see lanes 3 and 7), new high-molecular-weight bands (>66.2 kDa) were appeared for both CPPP and HPPP, suggesting that high-molecular-weight biopolymers were formed. A new band (approximately 97.4 kDa) was only appeared in TGase-treated CPPP, suggesting that unheated conarachin in CPPP might join in the cross-linking. Since there was no observable diminishment in the main subunit bands of CPPP and HPPP after TGase treatment (see lanes 3 and 7), the diminishment of the subunit bands (<66.2 kDa) and the broader molecular distribution in TGase-treated CPPP-DPH and HPPP-DPH (see lanes 4 and 8) might be attributed to the intermolecular cross-linking between peanut protein and DPH rather than only peanut protein subunits. It indicated that CPPP or HPPP was cross-linked with DPH by TGase, and more cross-linked CPPP or HPPP were formed after the addition of DPH.
Table 1 The amino acid composition of CPPP, HPPP, and DPH
Amino Acid Composition
The amino acid composition of CPPP, HPPP, and DPH are shown in . It was found that CPPP and HPPP had no obvious difference in the essential amino acid content, and both CPPP and HPPP were deficient in several essential amino acids, such as Thr, Met, and Lys. In comparison with CPPP and HPPP, DPH was rich in Thr, Val, Met, Ile, Leu, and Lys, which accounted for 4.3, 4.6, 2.7, 3.7, 6.7, and 8.9% of the total amino acid residues, respectively, indicating that the nutritional value of CPPP or HPPP can be improved by the addition of DPH. The amino acid Lys is considered to be very important to TGase cross-linking, and the high level of Lys in DPH might be the main reason for the enhanced susceptibility of CPPP or HPPP to TGase after the addition of DPH.
Changes in Emulsion Particle Size Distribution and Mean Particle Size D32
The particle size distributions of the emulsions are shown in and . For the emulsions of CPPP and TGase-treated CPPP (), a monomodal particle size distribution was observed and an increase in the particle size range was occurred after CPPP was treated with TGase. The emulsions of CPPP-DPH and TGase-treated CPPP-DPH showed a bimodal particle size distribution (), and the volume of small particles (d < 2 μm) in the emulsions was obviously increased from approximately 21.9 to 31.6% after TGase treatment. The increase of small particles in the emulsions implied the emulsifying activity might be improved.
Figure 2 Particle size distribution of the emulsions made with (a) CPPP samples and (b) HPPP samples.
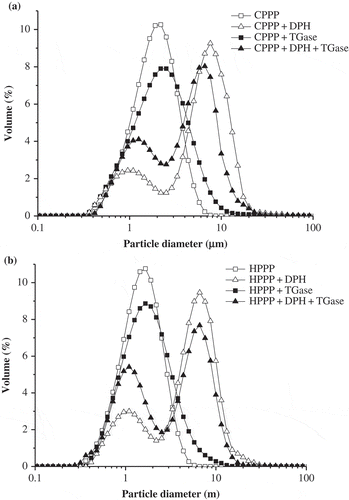
Similarly, the emulsions of HPPP and TGase-treated HPPP showed monomodal particle size distributions and the emulsions of HPPP-DPH and TGase-treated HPPP-DPH showed bimodal particle size distribution (). Compared to the HPPP emulsions, the particle size range of TGase-treated HPPP emulsions increased slightly. TGase treatment of HPPP-DPH caused a sharp increase (approximately from 23.8 to 40.8%) of volume of the small particles (d < 2 μm) in the emulsions.
The mean particle size d32 of the emulsions made with samples with and without TGase treatment is shown in . The d32 value is sensitive to the change in the number of small particles in the emulsions, which is considered to be related to emulsifying activity and low d32 value signify high emulsifying activity.[Citation25,Citation26] In this research, the d32 of HPPP was significantly lower (p < 0.05) than that of CPPP, suggesting that the emulsifying activity of HPPP was better. This was due to the hot pressing treatment induced the exposure of hydrophobic groups in the inner part of peanut proteins, resulting in increased surface hydrophobicity (data not shown). This seemed to be consistent with other research which found that heat treatment and extrusion could enhance the surface hydrophobicity of plant proteins.[Citation27,Citation28]
Figure 3 Effect of TGase treatment on the mean particle size d32 of emulsions. The columns having the same letter are insignificantly different (p > 0.05).
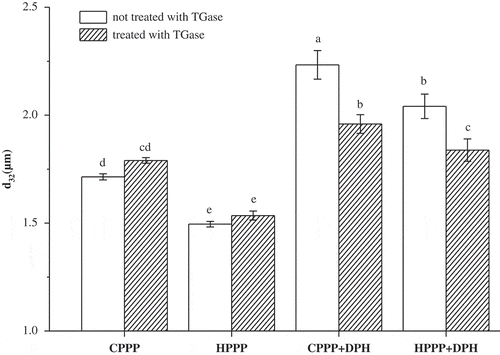
After TGase treatment, both CPPP and HPPP emulsions had no significant change in d32 (p > 0.05), indicating that TGase treatment had no beneficial effect on the emulsifying activity of peanut proteins. This finding was in agreement with previous publications concerning the emulsifying activity of TGase-treated plant proteins, such as cowpea, wheat, barley, and soy flours.[Citation29Citation30]
For the emulsions of CPPP-DPH and HPPP-DPH, the d32 of HPPP-DPH was significant smaller (p < 0.05) than that of CPPP-DPH, indicating that emulsifying activity of the HPPP-DPH mixture was better. After TGase cross-linking, the d32 of both CPPP-DPH and HPPP-DPH emulsions decreased significantly (p < 0.05), showing that the TGase treatment further improved the emulsifying activity of these mixtures. The possible explanation is that TGase treatment resulted in intermolecular cross-linking between proteins and peptides, causing an increase in molecular weight and the formation of branched-chain structures that is helpful for adsorbing and covering the oil-water interface.
Microstructure of Emulsions Analysis
The micrographs of the different emulsions are shown in . A slight flocculation was occurred in both CPPP and TGase-treated CPPP emulsions ( and ). The droplet size of HPPP () was smaller than that of CPPP emulsions, and a slight increase in droplet size was occurred with cross-linked HPPP (). The emulsions of CPPP-DPH and HPPP-DPH ( and ) showed extensive flocculation and aggregation, with CPPP-DPH showing poorer stability than the HPPP-DPH emulsions. However, a marked decrease in flocculation and coalescence was observed in the emulsions made with TGase-treated CPPP-DPH and HPPP-DPH ( and ), indicating that TGase cross-linking could improve the emulsion stability of the mixtures. The microscopic observations were consistent with the results of the droplet size distribution and the mean particle size d32 of the emulsions ( and ).
Figure 4 Microscopic structure of emulsions made with samples without (a, c, e, g) or with TGase (b, d, f, h) treatment observed by optical microscope at a magnification of 400×: (a) and (b), are cold-pressed peanut protein; (c) and (d), are hot-pressed peanut protein; (e) and (f), are cold-pressed peanut protein and Decapterus maruadsi hydrolysates; (g) and (h), are hot-pressed peanut protein and Decapterus maruadsi hydrolysates.
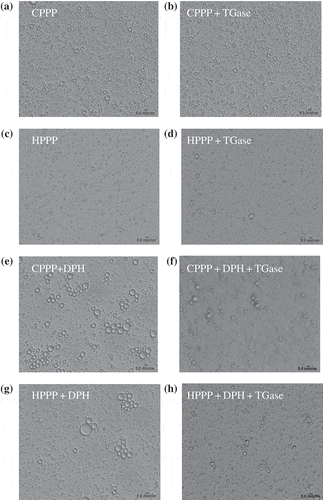
Evaluation of Emulsion Stability
The stability of different emulsions was evaluated using the changes in the particle size d43 value () and the creaming index () after 20 days of storage at room temperature. The d43 value is more sensitive to change in the larger particles in the emulsions than the d32 value, and it can provide a good indication for the droplet aggregation.[Citation31] The storage stability of emulsions can be evaluated using changes in the d43 value and the creaming index.[Citation23] TGase treatment induced a significant decrease (p < 0.05) in the d43 of CPPP emulsions and the creaming index after 20 days storage. TGase cross-linking caused no significant change (p > 0.05) in d43, but a significant decrease (p < 0.05) in the creaming index of HPPP emulsions after storage. The results showed that the emulsion stability of both CPPP and HPPP were improved by TGase cross-linking, which could be attributed to an increased resistance of the cross-linked proteins on the oil-water interface to deformation or rupture, and the surface layer rigidity could be enhanced by the presence of covalent cross-linking.[Citation29Citation32]
Figure 5 The changes in the mean particle size d43 of emulsions made with samples stored at room temperature for 20 days. The columns having the same letter are insignificantly different (p > 0.05).
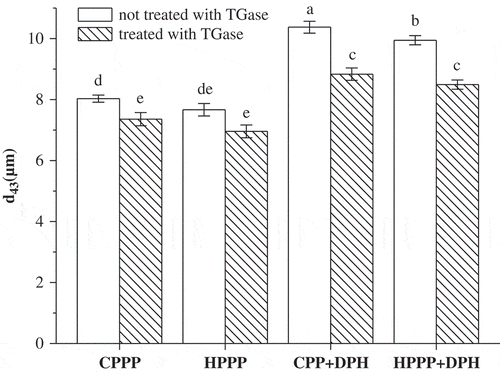
Figure 6 The changes in the creaming rate of emulsions made with samples stored at room temperature for 20 days. The columns having the same letter are insignificantly different (p > 0.05).
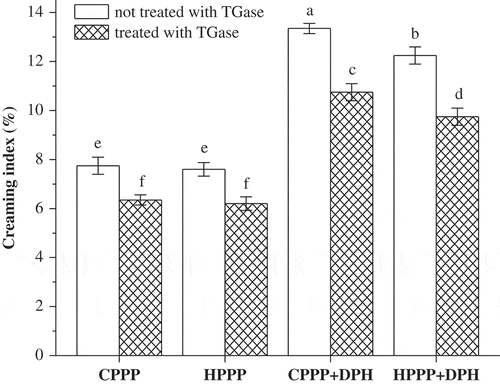
Compared to the emulsions of CPPP and HPPP, the d43 and the creaming index of the CPPP-DPH and HPPP-DPH emulsions after 20 days of storage were much higher. The inferior emulsion stability might be due to the presence of short chain peptides in DPH that could not cover the entire oil droplet surface to form a stable layer.[Citation14] When CPPP-DPH and HPPP-DPH were cross-linked by TGase, the d43 and creaming index decreased significantly (p < 0.05) after storage, indicating that TGase treatment could improve the emulsion stability of CPPP-DPH and HPPP-DPH. It might be attributed to benefits of the cross-linked protein-peptides creating branched structures that may be easily adsorbed on oil-water interface and give better oil droplet coverage.
CONCLUSION
It was demonstrated that the emulsifying properties of HPPP were better than that of CPPP. After being treated with TGase, the emulsifying activity of CPPP and HPPP was not improved, whereas the emulsion stability of both CPPP and HPPP were significantly enhanced. It was also found that CPPP or HPPP could be cross-linked with DPH by TGase, and the susceptibility of CPPP or HPPP subunits to TGase seemed to be promoted by the addition of DPH due to the presence of high Lys contents in the fish protein hydrolysate. Besides, the addition of DPH could remedy the disadvantage of essential-amino-acids deficiency in CPPP or HPPP. The emulsifying properties of CPPP-DPH and HPPP-DPH were improved by TGase cross-linking, indicating that the use of TGase to create cross-linked protein-peptides could be a feasible way to provide better proteins for the food industry.
FUNDING
The authors gratefully acknowledge the financial support from the National Natural Science Foundation of China (No. 31171783 and No. 31000759), Modern Agro-industry Technology Research System (CARS-49), and Special Scientific Research Funds for Central Non-profit Institutes (2011TS01).
REFERENCES
- FAO, Food and Agriculture Organization Statistics (FAOSTAT). http://faostat.fao.org/site/567/DesktopDefault.aspx?PageID=567#ancor ( accessed August 5, 2012.)
- Singh, B.; Singh, U. Peanut as a source of protein for human foods. Plant Foods for Human Nutrition 1991, 41, 165–177.
- Prodpran, T.; Benjakul, S.; Artharn, A. Properties and microstructure of protein-based film from round scad (Decapterus maruadsi) muscle as affected by palm oil and chitosan incorporation. International Journal of Biological Macromolecules 2007, 41, 605–614.
- Thiansilakul, Y.; Benjakul, S.; Shahidi, F. Compositions, functional properties, and antioxidative activity of protein hydrolysates prepared from round scad (Decapterus maruadsi). Food Chemistry 2007, 103, 1385–1394.
- Kristinsson, H.G.; Rasco, B.A. Fish protein hydrolysates: Production, biochemical, and functional properties. Critical Reviews in Food Science and Nutrition 2000, 40, 43–81.
- Ando, H.; Adachi, M.; Umeda, K.; Matsuura, A.; Nonaka, M.; Uchio, R.; Tanaka, H.; Motoki, M. Purification and characteristics of a novel transglutaminase derived from microorganism. Agricultural and Biological Chemistry 1989, 53, 2613–2617.
- Oner, Z.; Karahan, A.G.; Aydemir, S.; Sanlidere Aloglu, H. Effect of transglutaminase on physicochemical properties of set-style yogurt. International Journal of Food Properties 2008, 11 (1), 196–205.
- Lin, C.F.; Wang, Y.T.; Wu, J.S.B. Modification in physical properties of rice gel by microbial transglutaminase. Journal of the Science of Food and Agriculture 2009, 89, 477–481.
- Jiang, S.J.; Zhao, X.H. Transglutaminase-induced cross-linking and glucosamine conjugation in soybean protein isolates and its impacts on some functional properties of the products. European Food Research and Technology 2010, 231, 679–689.
- Liu, S.; Zhang, D.X.; Liu, L.; Wang, M.; Du, G.C.; Chen, J. Enhanced water absorption of wheat gluten by hydrothermal treatment followed by microbial transglutaminase reaction. Journal of the Science of Food and Agriculture 2010, 90, 658–663.
- Hu, X.; Zhao, M.M.; Sun, W.Z.; Zhao, G.L.; Ren, J.Y. Effects of microfluidization treatment and transglutaminase cross-Linking on physicochemical, functional, and conformational properties of peanut protein isolate. Journal of Agricultural and Food Chemistry 2011, 59, 8886–8894.
- Schäfer, C.; Neidhart, S.; Carle, R. Application and sensory evaluation of enzymatically texturized vegetable proteins in food models. European Food Research and Technology 2011, 232, 1043–1056.
- Babiker, E.E. Effect of transglutaminase treatment on the functional properties of native and chymotrypsin-digested soy protein. Food Chemistry 2000, 70, 139–145.
- Flanagan, J.; FitzGerald, R.J. Physicochemical and nitrogen solubility properties of bacillus proteinase hydrolysates of sodium caseinate incubated with transglutaminase pre- and post-hydrolysis. Journal of Agricultural and Food Chemistry 2002, 50, 5429–5436.
- Flanagan, J.; FitzGerald, R.J. Functional properties of Bacillus proteinase hydrolysates of sodium caseinate incubated with transglutaminase pre- and post-hydrolysis. International Dairy Journal 2003, 13, 135–143.
- Agyare, K.K.; Xiong, Y.L.; Addo, K. Influence of salt and pH on the solubility and structural characteristics of transglutaminase-treated wheat gluten hydrolysate. Food Chemistry 2008, 107, 1131–1137.
- Ren, J.Y.; Zhao, M.M.; Shi, J.; Wang, J.S.; Jiang, Y.M.; Cui, C.; Kakuda, Y.; Xue, S.J. Purification and identification of antioxidant peptides from grass carp muscle hydrolysates by consecutive chromatography and electrospray ionization-mass spectrometry. Food Chemistry 2008, 108, 727–736.
- Adler-Nissen, J. Determination of degree of hydrolysis of food protein hydrolysates by trinitrobenzenosulfonic acid. Journal of Agricultural and Food Chemistry 1979, 27, 1256–1262.
- Tang, C.H.; Chen, Z.; Li, L.; Yang, X.Q. Effects of transglutaminase treatment on the thermal properties of soy protein isolates. Food Research International 2006, 39, 704–711.
- Laemmli, U.K. Cleavage of structural proteins during the assembly of the head of the bacteriophage T4. Nature 1970, 227, 680–685.
- You, L.J.; Zhao, M.M.; Cui, C.; Zhao, H.F.; Yang, B. Effect of degree of hydrolysis on the antioxidant activity of loach (Misgurnus anguillicaudatus) protein hydrolysates. Innovative Food Science and Emerging Technologies 2008, 10 (2), 235–240.
- Luo, D.H.; Zhao, Q.Z.; Zhao, M.M.; Yang, B.; Long, X.T.; Ren, J.Y.; Zhao, H.F. Effects of limited proteolysis and high-pressure homogenisation on structural and functional characteristics of glycinin. Food Chemistry 2010, 122, 25–30.
- Chen, L.; Chen, J.S.; Ren, J.Y.; Zhao, M.M. Modifications of soy protein isolates using combined extrusion pre-treatment and controlled enzymatic hydrolysis for improved emulsifying properties. Food Hydrocolloids 2011, 25, 887–897.
- Chiou, R.Y.Y. Effects of heat treatments on peanut arachin and conarachin. Journal of Food Biochemistry 1990, 14, 219–232.
- Krause, J.P.; Dudek, S.; Schwenke, K.D. Changes in interfacial behaviour, emulsifying, and foaming properties of faba bean legumin after modification with dimethylsuberimidate. Nahrung 2000, 44, 403–406.
- Poon, S.; Clarke, A.E.; Schultz, C.J. Effect of denaturants on the emulsifying activity of proteins. Journal of Agricultural and Food Chemistry 2001, 49, 281–286.
- Silva, A.C.C.; Arêas, E.P.G.; Silva, M.A.; Arêas, J.A.G. Effects of extrusion on the emulsifying properties of rumen and soy protein. Food Biophysics 2010, 5, 94–102.
- Zhao, G.L.; Liu, Y.; Zhao, M.M.; Ren, J.Y.; Yang, B. Enzymatic hydrolysis and their effects on conformational and functional properties of peanut protein isolate. Food Chemistry 2011, 127, 1438–1443.
- Aluko, R.E.; Yada, R.Y. Effect of a microbial calcium-independent transglutaminase on functional properties of a partially purified cowpea (Vigna unguiculata) globulin. Journal of the Science of Food and Agriculture 1999, 79, 286–290.
- Ahn, H.J.; Kim, J.H.; Ng, P.K.W. Functional and thermal properties of wheat, barley, and soy flours and their blends treated with a microbial transglutaminase. Journal of Food Science 2005, 70, 380–386.
- Mun, S.; McClements, D.J.; Surh, J. Influence of maltodextrin addition on the freeze-dry stability of β-lactoglobulin-based emulsions with controlled electrostatic and/or steric interactions. Food Science and Biotechnology 2011, 20 (4), 1143–1150.
- Nonaka, M.; Toiguchi, S.; Sakamoto, H.; Kawajiri, H.; Soeda, T.; Motoki, M. Changes caused by microbial transglutaminase on physical properties of thermally induced soy protein gels. Food Hydrocolloids 1994, 8, 1–8.