Abstract
Eleven cereals were processed in a twin-screw extruder to produce directly expanded extrudates. Physical and functional properties (gelatinization degree, bulk density, expansion ratio, water absorption index, water solubility index, and sensory characteristics (hardness, crispness, and bowl life) of cereal extrudates were determined. Relationships among different variables were analyzed by SPSS. Gelatinization degree positively and negatively correlated to starch and protein, respectively (p ≤ 0.01). The same relationship was observed with water absorption index at the same significance level. Water solubility index positively correlated to protein (p ≤ 0.05), negatively correlated to starch (p ≤ 0.01), amylose (p ≤ 0.01), and fat (p ≤ 0.05). Density and bowl life positively correlated to fat (p ≤ 0.01) and amylose (p ≤ 0.05), respectively. Gelatinization degree had a high correlation with water solubility index (p ≤ 0.05). As gelatinization degree increased, water solubility index decreased. Crispness positively correlated to bowl life (p ≤ 0.01).
INTRODUCTION
For thousands of years, wheat, rice, and corn have served as major cereals in human diet, and the minor cereals include oats, barley, rye, sorghum, millet, and buckwheat.[Citation1] Grains are important sources of carbohydrates, proteins, fiber, and micronutrients. In recent years, there has been growing scientific evidence that grains contain a lot of health-promoting components such as antioxidants and dietary fiber. For instance, dietary fiber was found to effectively lower serum LDL cholesterol concentrations, protect against cardiovascular disease, type 2 diabetes mellitus, and colon cancer.[Citation2,Citation3] Other studies have also demonstrated that phenols in grains are able to scavenge radicals and inhibit copper (Cu2+)-mediated human low density lipoprotein (LDL) oxidation.[Citation4,Citation5] Given that most of the bioactive components of grain are situated in the bran and germ, whole grains have the potential to make a good contribution to providing dietary fiber, minerals, vitamins, and antioxidants.[Citation6] As such, it is of great importance to ensure daily intake of whole grain food, for its extraordinary benefits. However, in many western countries, the consumption of whole grain foods is far below the recommended three servings per day.[Citation1,Citation6] With the transition of people’s consumption structure and the growing demand for whole grain products, it is imperative to utilize various technologies to commercially exploit the rich source of cereals.
As one of the most important food processing technologies, extrusion cooking is a continuous unit operation that combines mixing, sterilization, and cooking with forming. Due to its low cost and high efficiency, extrusion cooking is widely used to process cereals or starches into food and industrial products, such as snack foods, baby foods, ready-to-eat breakfast cereals, and infant formulas.[Citation7] In recent years, researchers have turned their attention to understanding the role of extrusion process in relation to the properties of cereals.[Citation8,Citation9,Citation10] Functional and sensory properties of cereal extrudates like crispiness, bowl life, water absorption index (WAI), water solubility index (WSI) have been the focus of many studies, due to their importance in rehydration process. For instance, Ding et al.[Citation11] investigated the effect of extrusion conditions, including feed rate, feed moisture content, screw speed, and barrel temperature on the physical, functional, and sensory properties (density, expansion, WAI, WSI, hardness, and crispness) of an expanded rice snack. There are also some scholars discussing the relationship between chemical compositions and extruded product properties. Hood-Niefe and Tyler[Citation12] observed that expansion indices decreased, bulk density, particle densities, and hardness increased with an increase in protein or moisture content of pea flour. Positive relationship between chemical compositions and properties of cereal extrudates is essential for selecting suitable ingredients for extrudated products, in particular, for cereal breakfast and snacks. Despite the increasing use of extrusion-cooking technology, no systematic studies seem to exist on the relationship between the chemical compositions of various cereals and the properties of their extrudates. The purpose of this research was to investigate the effects of extrusion cooking on the physical, functional, and sensory properties of eleven common cereals. Furthermore, the relationships among different variables of cereal extrudates were also investigated.
MATERIALS AND METHODS
Raw Materials
Corn, wheat, rice, oat, barley, millet, black rice, sorghum, buckwheat, red bean, and green bean were purchased from a local food company in Wuxi, China. Grains were ground in a mechanical grinder (Linda Machinery, Wenling, Zhejiang, China) and were passed through a 0.63 mm mesh screen, then respectively sealed in polyethylene bags and kept at room temperature until use. The typical chemical compositions of the raw are shown in . Taka-diastase was purchased from Sigma (St. Louis, MO, USA).
Table 1 Proximate chemical compositions of raw materials (results were average of three replicates ± standard deviation).*
Preparation of Samples
According to moisture content as shown in , tap water was added to obtain the desired levels of feed moisture content (24 ± 1%). All the ingredients were weighed in 300 g batches and were blended in the mechanical grinder (Linda Machinery, Wenling, Zhejiang, China) for a period of time to ensure homogeneity of the feeding material before extrusion cooking.
Extrusion Cooking
A PTW24/25D twin screw co-rotating extruder (Thermo Electron Corporation, Houston, USA) with L/D ratio of 25:1 and screw diameter of 24 mm was used to produce cylindrical extrudates. The extruder was equipped with a Brabender twin screw volumetric feeder (Duisburg, Germany). One circular die with 5 mm diameter was used. The extruder had four temperature control zones and the temperatures were set at 70, 90, 120, and 140℃, respectively. A 100 rpm screw speed was used for all extrusions. The feed rate was kept constant at 15 rpm. The extruded samples were dried in a forced-air oven (Yiheng Instrument Corporation, Shanghai, China) at 58℃ for 2 h and stored in polyethylene bags until measurements were taken.
Chemical Analysis
Chemical analysis was carried out using the AOAC methods,[Citation13] moisture content was measured by oven drying at 105℃ until constant weight. Crude protein was determined according to the micro-Kjeldahl method, multiplied by their respective factors. For crude fat, the Soxhlet extraction method with ethyl ether as solvent was used. Total amylose content was determined on defatted starches basis by the method of Mcgrance et al.[Citation14] About 20 mg sample was accurately weighed and dissolved by heating in DMSO for 15 min in a water bath at 85°C. When dissolved, the solution was then diluted to 25 mL in a volumetric flask with deionized water. One milliliter of this solution was then diluted with 50 mL of water, 5 mL of a solution of iodine (0.0025 mol/L) in potassium iodide (0.0065 mol/L) added with mixing and the absorbance of a sample of this solution in a 1 cm path length quartz cell read at 600 nm using a visible spectrophotometer after 15 min. A standard curve was plotted for mixtures of amylose and amylopectin from potato containing 0, 10, 25, 50, 75, and 100% amylose. Total starch content was measured by the enzymatic method of Mccleary et al.[Citation15] Briefly, some deionized water was added to the sample in a beaker to aid wetting. The beaker was heated for 10 min in a boiling water bath. After cooling to the room temperature, middle-temperature amylase (3000 U, 50 ul) was added and the beaker was incubated at 50℃ for 60 min. The contents were then quantitatively transferred to a 100 ml volumetric flask, and the volume was adjusted with deionized water. The glucose content was measured according to AOAC methods.[Citation13] Total starch = △E/W*0.9, where △E is the content of glucose against the blank sample; W is the weight of sample; 0.9 is a factor to convert free glucose to starch. All results were expressed as the mean (± SD) of triplicate analyses.
Physical and Functional Analysis
Gelatinization degree, bulk density, and expansion ratio
The degree of starch gelatinization in cereal extrudates was determined enzymatically as described by Bhattacharya and Hanna.[Citation16] Bulk density, defined as the ratio of the mass of the sample to that of its volume including the voids, was measured as described by Jones et al.[Citation17] About 10 g of the sample was weighed and put into a 250 ml volumetric cylinder. Sea sands were then used to fill the remaining space, shaking the cylinder to pack down the sea sands. The weight of the 250 ml volumetric cylinder filled with sea sands was also determined. Determinations were performed five times.
WAI and WSI
The WAI and WSI were measured by the method of Anderson et al.[Citation19] The extrudates were ground to pass through a 0.63 mm mesh screen. About 2.5 g of ground samples from each treatment were weighed and suspended in 30 ml of distilled water at 30℃, intermittently stirred for 30 min, and then centrifuged at 3000 × g for 10 min. The supernatant was decanted into a pre-weighed evaporating dish and evaporated to dryness at 105℃ until a constant weight. The WSI was calculated by dividing the weight of dried solids from the supernatant by the original weight of sample. The WAI was expressed as the ratio between weight of gel and original weight of sample. The results were expressed as the average of three measurements.
Instrumental Textural Measurement
The texture characteristics of extrudates were measured using a Stable Micro System TA.XT plus texture analyser (Stable Microsystems, Surrey, UK). A force-time curve was recorded and analyzed by Texture Exponent 32 (Stable Microsystems, Surrey, UK) to calculate the peak force and area. For each test, a 3-point bending rig (HDP/3PB) was used by keeping the distance between the beams as 40 mm. The conditions used for the experiments were as follows: mode, measure force in compression; option, return to start; speeds: pretest 2.0 mm/s, test 2.0 mm/s, posttest 5.0 mm/s, and trigger force 0.1 N. The peak force in the force-deformation curve was represented as hardness and the linear distance was taken as crispness, respectively. The crispness of sample after soaking in the water for 5 min was taken as bowl life. Ten randomly collected samples of each extrudate were measured and an average taken.
Scanning Electron Microscopy (SEM)
Prior to SEM analysis, samples were cut using a razor blade and sputter coated with 20 nm thick gold, imaged in a scanning electron microscope using accelerating voltage of 5 kV (Quanta-200, FEI, Holland).
Statistical Analysis
All numerical results were the average of at least three independent replicates. Statistical analysis was conducted using SPSS 13.0 for Windows (SPSS, Inc., Chicago, IL, USA). Statistical significant differences between values and correlation between parameters were evaluated with one-way analysis of variance (ANOVA) and Bivariate Correlations, respectively.
RESULTS AND DISCUSSION
Gelatinization Degree
Gelatinization is an important processing step determining the physical properties of starch-based products. During gelatinization, the molecular order of the starch granules is irreversibly destroyed by structural changes which involve changes of shape and size of granules, absorption of water and swelling, crystallite melting, and leaching of amylose (amylopectin) from the granules.[Citation20] Under high temperature, pressure and shear force, extrusion cooking causes structural transformations in the starchy material, including the gelatinization and degradation of starch. presents the data on the gelatinization degree of extrudates. Except red bean, extrusion cooking increased degree of gelatinization to over 90%. It has been suggested that the factors such as, presence of intact tissue/cell structures enclosing starch granules hinders the swelling and solubilization of starch; formation of retrograded starch in cooked starch; presence of high viscous, soluble dietary fibers[Citation21,Citation22] may explain the lower gelatinization degree observed in red bean.
Expansion Ratio And Bulk Density
The physical properties of the extrudates, including CE, LE, and bulk density are presented in . Extrusion results in highly expanded products and significantly lower bulk densities.[Citation8] Analysis of the data showed that bulk densities of cereal extrudates ranged from 0.425 ± 0.015 to 0.982 ± 0.008, with the highest bulk density obtained with oat flour containing 24% moisture. This could be attributed to its high content of fat and protein. Protein decreases shear within the extruder, and reduces the amount of moisture flashed off, thus expansion is decreased.[Citation12] Fat also provides a lubricant function for extrusion cooking and may reduce the grinding effect within raw materials, thereby lowering expansion. More importantly, due to the ability of amylose to bind lipids, extrusion cooking results in the formation of amylose-lipid complexes, thus increasing bulk density and creating a more compact macrostructure.[Citation23,Citation24]
Table 2 Physical and textural properties of cereal extrudates
Expansions ratio of extrudates was measured by CE, and LE. Compared with the other cereals, CE of buckwheat extrudate was the highest. This might reflect some combination of its lower degree of packing between the buckwheat starch granules, more suitable feed moisture content (24%), and amylose/amylopectin ratio. The starch granules of buckwheat were very small (1–7 mm) and regular, clearly grouped in regular clusters delineated by the presence of air spaces between them.[Citation25] According to Chinnaswamy and Hanna,[Citation26] the expansion of starch increased from 8–16.4 as amylose content increased from 0–50%, and then decreased. Jyothi et al.[Citation8] reported that expansion of arrowroot starch extrudate was found to be most dependent on feed moisture and temperature. Increased feed moisture may lead to a sharp decrease in expansion value.[Citation11] In general, an inverse trend was observed between LE and CE, which is due to the release of water vapour and consistent with their definitions.
WAI and WSI
WAI reflects the ability of flour to absorb water or the swelling ability of starch. It depends on the availability of hydrophilic groups, which bind water molecules and on the gel-forming capacity of macromolecules.[Citation27] At high temperature and under shear, extrusion cooking results in the disruption of some hydrogen bonds among starch molecules. The crystalline structure in starch granular is thus destroyed and exposes more hydroxyl groups. Therefore, WAI increases with the degree of starch destruction. presents the data on WAI of the cereals and their extrudates. Compared to the native grains, the extrudates showed significantly higher WAI. This could be explained by the formation of the porous macrostructure (Fig. 3) in the die outlet facilitating the entry of water and its combination with hydrophilic groups, resulting in higher WAI of extrudates. The difference in the WAI values of cereal extrudates can be explained by their various content of damaged starch, which allows the flour to become more hydrophilic.
Figure 2 (a) WAI and (b) WSI of corn (C), rice (R), millet (M), black rice (Br), wheat (W), barley (B), oat (O), buckwheat (Bw), sorghum (S), red bean (Rb), and green bean (Gb) and their respective extrudates.
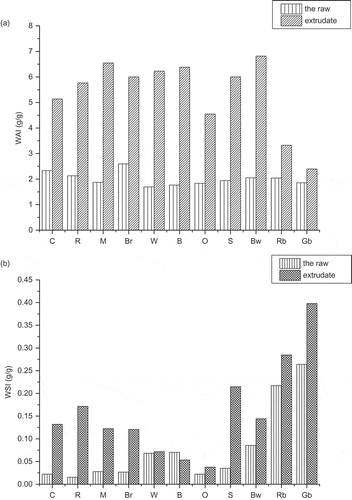
WSI measures the amount of soluble polysaccharide released from the starch after extrusion.[Citation11] It reflects the macromolecular degradation of starch at process variables likely to be heated and to be ground in extrusion cooking.[Citation28] presents the data on WSI of the cereals and extrudates. Except wheat and barley, extrusion cooking significantly increased the WSI of extrudates. A similar tendency was observed by Alonso et al.[Citation29] in pea and kidney bean proteins. The graph shows a great increase of approximately 11 times in WSI of rice extrudate, due to its high content of amylopectin. According to Zhu et al.,[Citation30] amylopectin is the primary fraction that undergoes fragmentation during extrusion process. The generation of low molecular weight starch fractions, resulting from the depolymerization of amylopectin branches during extrusion, may enhance the WSI. The lower WSI value of wheat and barley extrudates may be connected with their high content of starch, insoluble proteins and dietary fiber.
Instrumental Texture Properties-Hardness, Crispness, and Bowl Life
The results of the analysis are shown in . The hardness and crispness of expanded extrudate is a perception of the human being and are associated with the expansion and cell structure of the product. Hardness is the average force required for a probe to penetrate the extrudate.[Citation11] Feed moisture and screw speed combination with temperature were found to have a significant (p < 0.05) effect on the hardness.[Citation8,Citation9] The present study indicated that the hardness of wheat and oat extrudate were significantly higher and lower than the other extrudates, respectively (). also showed that the cell wall of wheat extrudate was more compact and thicker than oat extrudate. Comparison of the variable hardness plots to the corresponding density in suggested that the higher values of hardness were often associated with lower bulk density. For breakfast cereal, the crispness loss due to the adsorption of ambient moisture or due to the water mass transfer from neighboring components is a major cause of rejection by the consumers.[Citation31] Therefore, bowl life is a salient textural attribute of breakfast cereal strongly related to its preference. Factors affecting crispness are the properties of the material including composition, physical properties of the components, and the structure of the product.[Citation32] shows that readings of crispness and bowl life were close and shared the same trend. The crispness and bowl life of wheat, corn, barley, rice, and oat were significantly higher than those of the other extrudates.
Correlation Between Chemical Compositions and Properties of Cereal Extrudates
Attempts to establish the relationship between chemical compositions and properties of extruded products have been ongoing and some positive results have been achieved. In the present study, the correlation coefficients of the relationship between the determined properties of the extrudates from different cereals and their chemical compositions are shown in . Gelatinization degree positively correlated to starch (p ≤ 0.01), which was confirmed by the report of Van Hoan et al.[Citation33] However, gelatinization degree negatively correlated to protein (p ≤ 0.01). This might be due to the fact that starch and protein are the two major components in grains. An increase in protein content may lead to lower content of starch, thus lowering degree of gelatinization.
Table 3 Correlation coefficients between the chemical compositions of cereals and properties of extruded products
Protein and starch had a high correlation with WAI. As protein decreased and starch increased, WAI increased (p ≤ 0.01). According to Zhu et al.,[Citation30] reduced starch content, which means less starch is available to be gelatinized during extrusion cooking and subsequently absorb water, may lead to lower WAI. WSI negatively correlated with starch, amylose (p ≤ 0.01), and fat (p ≤ 0.05), but positively correlated with protein (p ≤ 0.05). These coefficient values indicated that there was a strong relation between chemical compositions and WSI. During extrusion cooking, starch (mainly amylopectin) undergoes great destruction,[Citation34] resulting in an increase in WSI. However, high starch content would increase the difficulty of extrusion processing. It was reported that the lubricant effect of fat reduced the friction between dough and screw elements as well as barrel, thereby preventing the starch granules from mechanical breakdown.[Citation23]
In this study, fat was found to positively correlate with bulk density (p ≤ 0.01), which was attributed to the formation of starch-lipid complexes that gives extrudate a compact structure. Despite the importance of bowl life, to the authors knowledge, there were few literatures focusing on the relationship between chemical compositions and bowl life. shows that bowl life positively correlated with amylose (p ≤ 0.05). The result could be explained by its relative stability and the formation of amylose-lipid complexes during the extrusion cooking.
Correlation Coefficients of Physical, Functional, and Sensory Properties
shows the correlation coefficients of the relationship between the determined properties of the extrudates. Gelatinization degree had a high correlation with WSI. As the gelatinization degree increased, WSI decreased (p ≤ 0.05). Gelatinization and degradation of starch are two structural transformations occurring during extrusion cooking. Higher degree of gelatinization can lead to less degradation of starch, thus lowering WSI. In general, the WSI trends were inverse of those for WAI, which has been observed by many authors.[Citation30,Citation35] CE negatively correlated with LE (p ≤ 0.01), and bulk density (p ≤ 0.05), which are quite logical and consistent with their definitions. Crispness positively correlated to bowl life (p ≤ 0.01), indicating selection of raw materials plays an important role in determining the final texture characteristics of breakfast cereals.
Table 4 The correlation coefficients between the physical, functional and sensory properties of cereal extrudates
CONCLUSIONS
The results showed that extrusion cooking could bring about great improvement in physical and functional properties of cereals, including gelatinization degree, WAI, and WSI. Extrudates with high degree of gelatinization, adequate porosity, and much improved dispersibility are more easily digested by consumers. Extrudate properties, such as gelatinization degree, WAI, WSI, bulk density, and bowl life showed a high correlation with chemical compositions of the raw. The results provided significant insight into the selection of suitable ingredients for snack foods and breakfast cereal. As compared to the other cereals, corn, rice, and barley with relatively high expansion ratio, high crispness, and low hardness are more preferable. Meanwhile, it is advisable to add a certain amount of amylose to ensure the bowl life of breakfast cereals. In addition, gelatinization degree had a high correlation with WSI. As gelatinization degree increased, WSI decreased (p ≤ 0.05). WSI and CE negatively correlated with WAI (p ≤ 0.05) and LE (p ≤ 0.01), respectively. Crispness positively correlated to bowl life (p ≤ 0.01). It was demonstrated that by properly selecting processing conditions and ingredient composition, cereal extrudates with desirable attributes could be produced.
ACKNOWLEDGMENT
The excellent technical assistance of Testing and Analysis Center of Jiangnan University is gratefully acknowledged.
FUNDING
This research was supported by Project of China National Key Technology Research and Development Program for the 12th five-year plan (NO.2012BAD37B08).
REFERENCES
- Liu, R.H. Whole grain phytochemicals and health. Journal of Cereal Science 2007, 46, 207–219.
- Kaczmarczyk, M.M.; Miller, M.J.; Freund, G.G. The health benefits of dietary fiber: Beyond the usual suspects of type 2 diabetes mellitus, cardiovascular disease, and colon cancer. Metabolism 2012, 61 (8), 1058–1066.
- Theuwissen, E.; Mensink, R.P. Water-soluble dietary fibers and cardiovascular disease. Physiology & Behavior 2008, 94, 285–292.
- Cirico, T.L.; Omaye, S.T. Additive or synergetic effects of phenolic compounds on human low density lipoprotein oxidation. Food and Chemical Toxicology 2006, 44, 510–516.
- Ragaee, S.; Abdel-Aal, E.S.M.; Noaman, M. Antioxidant activity and nutrient composition of selected cereals for food use. Food Chemistry 2006, 98, 32–38.
- Arvola, A.; Lahteenmaki, L.; Dean, M.; Vassallo, M.; Winkelmann, M.; Claupein, E.; Saba, A.; Shepherd, R. Consumers’ beliefs about whole and refined grain products in the UK, Italy, and Finland. Journal of Cereal Science 2007, 46, 197–206.
- Zazueta-Morales, J.; Martinez-Bustos, F.; Jacobo-Valenzuela, N.; Ordorica-Falomir, C.; Paredes-Lopez, O. Effects of calcium hydroxide and screw speed on physicochemical characteristics of extruded blue maize. Journal of Food Science 2002, 67 (9), 3350–3358.
- Jyothi, A.N.; Sheriff, J.T.; Sajeev, M.S. Physical and functional properties of arrowroot starch extrudates. Journal of Food Science 2009, 74 (2), 97–104.
- Liu, C.M.; Zhang, Y.J.; Liu, W.; Wan, J.; Wang, W.H.; Wu, L.; Zuo, N.B.; Zhou, Y.R.; Yin, Z.L. Preparation, physicochemical, and texture properties of texturized rice produce by improved extrusion cooking technology. Journal of Cereal Science 2011, 54, 473–480.
- De Pilli, T.; Severini, C.; Baiano, A.; Derossi, A.; Arhaliass, A.; Legrand, J. Effects of operating conditions on oil loss and properties of products obtained by co-rotating twin-screw extrusion of fatty meal: Preliminary study. Journal of Food Engineering 2005, 70, 109–116.
- Ding, Q.B.; Ainsworth, P.; Tucker, G.; Marson, H. The effect of extrusion conditions on the physicochemical properties and sensory characteristics of rice-based expanded snacks. Journal of Food Engineering 2005, 66, 283–289.
- Hood-Niefer, S.D.; Tyler, R.T. Effect of protein, moisture content, and barrel temperature on the physicochemical characteristics of pea flour extrudates. Food Research International 2010, 43, 659–663.
- Association of Official Analytical Chemists. Official Methods of Analysis, AOAC International, Arlington, VA, 16th edition, 1995.
- Mcgrance, S.J.; Cornell, H.J.; Rix, C.J. A simple and rapid colorimetric method for the determination of amylose in starch products. Starch/Starke 1998, 50, 158–163.
- Mccleary, B.V.; Gibson, T.S.; Solah, V.; Mugford, D.C. Total starch measurement in cereal products: Interlaboratory evaluation of a rapid enzymatic test procedure. Cereal Chemistry 1994, 71, 501–505.
- Bhattacharya, M.; Hanna, M.A. Kinetics of starch gelatinization during extrusion cooking. Journal of Food Science 1987, 52 (3), 764–766.
- Jones, D.; Chinnaswamy, R.; Tan, Y.; Hanna, M. Physiochemical properties of ready-to-cat breakfast cereals. Cereal Foods World 2000, 45 (4), 164–168.
- Chung-Wen, S. Effects of eggshell powder addition on the extrusion behavior of rice. Journal of Food Engineering 2007, 79, 607–612.
- Anderson, R.A.; Conway, H.F.; Pfeifer, V.F.; Griffin, E.L. Gelatinization of corn grits by roll and extrusion cooking. Cereal Science Today 1969, 14, 4–12.
- Spigno, G.; De Faveri, D.M. Gelatinization kinetics of rice starch studied by non-isothermal calorimetric technique: Influence of extraction method, water concentration, and heating rate. Journal of Food Engineering 2004, 62, 337–344.
- Eyaru, R.; Shrestha, A.K.; Arcot, J. Effect of various processing techniques on digestibility of starch in red kidney bean (Phaseolus vulgaris) and two varieties of peas (Pisum sativum). Food Research International 2009, 42, 956–962.
- Siddhuraju, P.; Becker, K. Effect of various domestic processing methods on antinutrients and in vitro protein and starch digestibility of two indigenous varieties of Indian Tribal Pulse, Mucuna pruriens Var utilis. Journal of Agricultural and Food Chemistry 2001, 49, 3058–3067.
- De Pilli, T.; Derossi, A.; Talja, R.A.; Jouppila, K.; Severini, C. Study of starch-lipid complexes in model system and real food produced using extrusion-cooking technology. Innovative Food Science and Emerging Technologies 2011, 12, 610–616.
- De Pilli, T.; Jouppila, K.; Ikonen, J.; Kansikas, J.; Derossi, A.; Severini, C. Study on formation of starch–lipid complexes during extrusion-cooking of almond flour. Journal of Food Engineering 2008, 87, 495–504.
- Mariotti, M.; Alamprese, C.; Pagani, M.A.; Lucisano, M. Effect of puffing on ultrastructure and physical characteristics of cereal grains and flours. Journal of Cereal Science 2006, 43, 47–56.
- Chinnaswamy, R.; Hanna, M.A. Relationship between amylose content and extrusion-expansion properties of corn starches. Cereal Chemistry 1988, 65 (2), 138–143.
- Sompong, R.; Siebenhandl-Ehn, S.; Berghofer, E.; Schoenlechner, R. Extrusion cooking properties of white and colored rice varieties with different amylose content. Starch/Starke 2011, 63 (2), 55–63.
- Su, C.W.; Kong, M.S. Effects of soybean oil, cellulose, and SiO2 addition on the lubrication and product properties of rice extrusion. Journal of Food Engineering 2007, 78, 723–729.
- Alonso, R.; Orue, E.; Zabalza, M.J.; Grant, G.; Marzo, F. Effect of extrusion cooking on structure and functional properties of pea and kidney bean proteins. Journal of the Science of Food and Agriculture 2000, 80, 397–403.
- Zhu, L.J.; Shukri, R.; de Mesa-Stonestreet, N.J.; Alavi, S. Mechanical and microstructural properties of soy protein-high amylose corn starch extrudates in relation to physiochemical changes of starch during extrusion. Journal of Food Engineering 2010, 100, 232–238.
- Saeleaw, M.; Schleining, G. A review: Crispness in dry foods and quality measurements based on acoustic-mechanical destructive techniques. Journal of Food Engineering 2011, 105, 387–399.
- Primo-Martin, C.; Castro-Prada, E.M.; Meinders, M.B.J.; Vereijken, P.F.G.; Van Vliet, T. Effect of structure in the sensory characterization of the crispness of toasted rusk roll. Food Research International 2008, 41, 480–486.
- Van Hoan, N.; Mouquet-rivier, C.; Treche, S. Effects of starch, lipid, and moisture contents on extrusion behavior and extrudate characteristics of rice-based blends prepared with a very-low-cost extruder. Journal of Food Process Engineering 2010, 33, 519–539.
- Guha, M.; Ali, S.Z. Molecular degradation of starch during extrusion cooking of rice. International Journal of Food Properties 2002, 5 (3), 509–521.
- Chevanan, N.; Rosentrater, K.A.; Muthukumarappan, K. Twin-screw extrusion processing of feed blends containing distillers dried grains with solubles (DDGS). Cereal Chemistry 2007, 84 (5), 428–436.