Abstract
The purpose of this work was to examine the viscoelastic properties of Spanish honeys with various sugar contents [fructose (32–42 g/100 g honey), glucose (24–35 g/100 g honey), sucrose (0.0–3.4 g/100 g honey)]; concentrations (79–83 °Brix), and moisture levels (16–19 g/100 g honey) at different temperatures (5, 10, 15, 20, 25, 30, and 40°C). Honey showed Newtonian behaviour, presenting a highly viscous part (loss modulus was much greater than the elastic modulus). The loss modulus (G″) and viscosity increased with moisture content and a decrease with temperature. Exponential and power law models were applied to fit loss modulus and viscosity data. Polynomial models were proposed to describe the combined effect of temperature, fructose, glucose, sucrose content, other sugars, non-sugar substance, and moisture content.
INTRODUCTION
Knowledge about the rheological behaviour of viscous food stuff is useful in quality and process control, the calculation of energy requirements, and the selection of suitable processing equipment.[Citation1] Recently, the influence of temperature on the flow behaviour of honey varieties has been studied;[Citation2−Citation17] however, there are few articles related to the effect of temperature on the viscoelastic behaviour of honeys.[Citation18] In particular, little information is available on the viscoelastic properties of Spanish honeys with different botanical origins.
The purpose of this study was, on one hand, to observe the influence of temperature and total soluble content on viscosity and the loss modulus of different honeys and, on the other hand, to obtain a polynomial model that could explain the behaviour of viscosity and the loss modulus as a function of the chemical composition (sugars, moisture, etc.) and temperature.
MATERIALS AND METHODS
Materials
Eleven different types of Spanish honey were used in this study. Before being used, they were warmed to 55°C to dissolve any crystals, and kept in flasks at 30°C to remove air bubbles that could interfere in rheological studies. This was done because rheological properties can be influenced by the presence of crystals and air bubbles.[2,19,20]
Moisture Content
The moisture content of the honey samples was obtained by measuring the refractive index at 20°C using a digital refractometer (3T Atago Abbe refractometer, Atago Co., Tokyo, Japan). The water content and °Brix concentration were determined based on a Chataway table.[Citation21] Each measurement was taken in duplicate.
Sugar Determination
Glucose, fructose, and sucrose in honey samples were measured using a HPLC 10ADVP-Shimadzu, with RI-detector, according to a method described by Bogdanov.[Citation21] The linear regression factor of the calibration curves was higher than 0.9982 for all sugars. Sugars were quantified by comparison of the peak area obtained with those of standard sugars. The results for each sugar were expressed as g/100 g. Values of parameters were expressed as the mean and standard deviation to a confidence interval of 95% for the mean.
Viscoelastic Measurement
The dynamic rheological properties of honey samples were obtained with a RheoStress 1 rheometer (Thermo Haake, Germany) at different temperatures (5, 10, 15, 20, 25, 30, and 40°C), using a parallel plate system (Ø 60 mm) at a gap of 500 μm. A batch of each composition was prepared and at least two measurements were performed on each batch, using a fresh sample for each measurement. After loading the sample, a waiting period of 5 min was used to allow the sample to recover itself and to reach the desired temperature. In order to determine the linear viscoelastic region, stress sweeps were run at 1 Hz first. Then, the frequency sweeps were performed over the range w = 0.62–62.83 Hz at 1 Pa stress. The 1 Pa stress was in the linear viscoelastic region. Rheowin Job software (v. 2.93, Haake) was used to obtain the experimental data and to calculate storage (or elastic) modulus (G′), loss (viscous) modulus (G″), and complex viscosity ().
Statistical Analysis
The variables were weighted with the inverse of the standard deviation of all objects in order to compensate for the different scales of the variables. The proposed models have been made up using SPSS trial version, Excel 2007, Statgraphics trial version, and Design Expert trial version.
To verify the suitability of the models, the mean relative deviation modulus D (Eq. 1) was calculated:
RESULTS AND DISCUSSIONS
Physicochemical parameters of honey are summarised in . The moisture content ranged between 15.60–19.10 g/100 g and met the threshold requirement of moisture content required by the Codex Alimentarius (max. 20 g/100 g).[Citation22] Sugars represent the main components of any type of honey. Reducing sugars (invert sugar), mainly fructose and glucose, are the major constituents of honey but small quantities of sucrose are also present.[Citation23] The actual proportion of fructose to glucose, in any particular honey, depends largely on the source of the nectar.[Citation7−Citation9] The total content of glucose and fructose was over 60 g/100 g of honey, in accordance with the 2001/110/CE Directive. The fructose/glucose ratio was calculated for all samples. This ratio gives information about the crystallization state of honey: when fructose is higher than glucose honey is fluid. In all of the cases, this ratio was higher than 1 (), which was confirmed by the crystallization state of the mentioned honey sample; all of the honeys were in a liquid state. For the rheological characterization of honey, the F/G ratio must be higher than 1; if not, the rheological parameters could not be determined due to crystallization of the honey.
Table 1 Physicochemical parameters of honey
presents a typical rheogram of a honey sample in a 0.10–10 Hz frequency domain at 5–40°C; it shows the changes in storage modulus (G′) and loss modulus G″ with the frequency, while the viscosity is not influenced by the shear stress. All of the samples analysed exhibited a Newtonian behaviour (). The rheological parameters (G′, G″ η) increased with the increment of the frequency applied to the sample, showing that G″ had a greater magnitude than G′. All the viscoelastic parameters are positively influenced by the °Brix concentration. In general, G′ is less important than G″ due to its low value with respect to G″ (G″ > G′) as indicated by Doublier and Cuvelier.[Citation24] Therefore, the elastic behaviour of honey seems to be less important than its viscous behaviour, as noted by Yoo.[Citation18] The rheological properties of honey can also be affected by additional factors, such as sugars and other polymeric compounds in the honey.[Citation4,Citation8] In particular, the sugar content (glucose and fructose) can greatly affect the rheological properties because these sugars have different rheological behaviours. The models used for predicting the loss modulus and viscosity (with respect to temperature and concentration), presented below, use the magnitude of the two parameters at 1 Hz.
Figure 1 Typical rheogram of honey (A: elastic modulus; B: loss modulus; C: viscosity) at different temperatures: rhombus 5°C, square 10°C, triangle 15°C, multiplication symbol 20°C, cyrillic small letter zhe 25°C, circle 30°C, and plus 40°C.
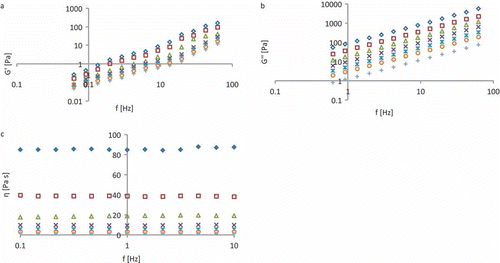
Viscosity Modeling
Influence of temperature
The influence of temperature on honey viscosity is described by the Arrhenius model (Eq. 2):
Table 2 Influence of temperature on honey viscosity
Effect of concentration
The increase of honey viscosity with soluble solid content (C, °Brix) can be described by power-law (Eq. 3):
Table 3 Effect of °Brix concentration (C) on the honey’s viscosity at different temperature (T)
Taking into account the mean relative deviation, it seems that the exponential models are suitable for predicting the influence of the concentration on the magnitude of viscosity. For a given temperature, the activation energy for flow depends on the soluble solid content, which can be described by several models.[Citation26] Two models similar to Eqs. (5) and (6) were used:
Table 4 Influence of the °Brix concentration on the viscosity’s activation energy
Combined Effect of Temperature and Concentration
For practical applications, it is useful to obtain an equation describing the combined effect of temperature and concentration on viscosity.[Citation27] The combined influence of temperature and concentration on honey viscosity is represented in . The following models for viscosity (Eqs. 7 and 8) were investigated:
Figure 2 Variation of viscosity with temperature and °Brix concentration (spatial representation with representation by contours).
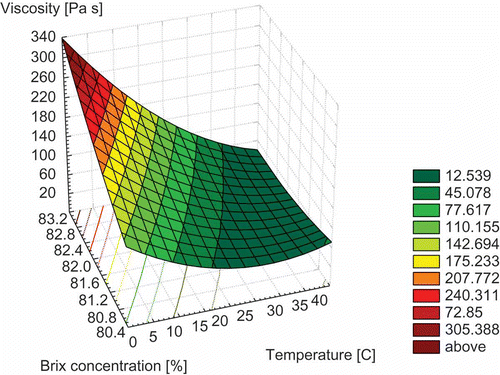
The viscosity, concentration, and temperature data were fitted to these models by nonlinear regression and the values of the model constants were determined. The values of these constants are summarised in . The viscosity predictions with temperature and °Brix concentration achieve coefficients of regression between 0.67 and 0.77. The mean relative deviation D certifies that the suitable model corresponds to Eq. (7). Therefore, for the interval of °Brix concentrations and temperatures (T) studied, the following equation (Eq. 9) is proposed to evaluate honey viscosity:
Table 5 Combined effect of °Brix concentration and temperature on the honey’s viscosity
Polynomial Modeling of Viscosity
The data model regarding the prediction of honey viscosity according to its chemical composition (fructose, glucose, sucrose, sugars (the difference between °Brix concentration—reported as dry matter—and the sum of fructose, glucose, and sucrose), non-sugars components (the difference, reported to 100 g, between 100 g and the sum of moisture content and °Brix concentration), moisture content, and temperature was made using a 3rd grade polynomial equation with seven variables. The measured and predicted values have been compared to check the suitability of the model. The equation of the model is shown below (Eq. 10):
The coefficient of regression of the proposed model represents 99.91% (R2 adjusted 99.84%). The model is a significant one (P = 0.001). In , the measured and predicted values of viscosity are plotted.
Table 6 Correspondence between actual and coded values of design variables
Loss Modulus Modeling
Influence of temperature
The influence of temperature on honey loss modulus is described by the Arrhenius model (Eq. 12):
Effect of Concentration
The increase of honey loss modulus with soluble solid content (C, °Brix) can be described by power-law (Eq. 13):
Table 7 Influence of temperature on honey loss modulus
Table 8 Effect of °Brix concentration (C) on the honey loss modulus at different temperature (T)
Taking into account the mean relative deviation, it seems that the exponential models are suitable for predicting the influence of the concentration on the magnitude of loss modulus. For a given temperature, the activation energy for flow depends on soluble solid content, which can be described by several models.[Citation26] Two models similar to Eqs. (15) and (16) were used:
Table 9 Influence of the °Brix concentration on the loss modulus’s activation energy
Combined Effect of Temperature and Concentration
For practical applications, it is useful to obtain an equation describing the combined effect of temperature and concentration on honey loss modulus.[Citation27] The combined influence of temperature and concentration on honey loss modulus is presented in . The following models for loss modulus (Eqs.17 and 18) were investigated:
Figure 4 Variation of loss modulus (G”) with temperature and °Brix concentration (spatial representation with representation by contours).
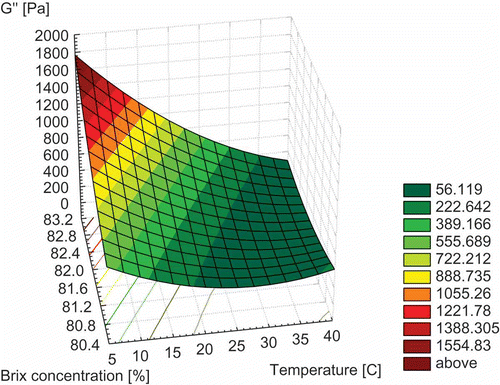
The loss modulus, concentration, and temperature data were fitted to these models by nonlinear regression and the values of the model constants were determined. The values of these constants are summarised in . The loss modulus predictions with temperature and °Brix concentration achieve coefficients of regression between 0.65 and 0.75. The mean relative deviation D certifies that the suitable model corresponds to Eq. (17). Therefore, for the interval of °Brix concentrations and temperatures (T) studied, the following equation (Eq. 19) is proposed to evaluate the loss modulus (G″) of honeys:
Table 10 Combined effect of °Brix concentration and temperature on the honey’s loss modulus
Polynomial Modeling of Loss Modulus
The data model regarding the prediction of honey loss modulus (G″) according to its chemical composition (fructose, glucose, sucrose, sugars [the difference between °Brix concentration—reported as dry matter—and the sum of fructose, glucose, and sucrose]), non-sugars components (the difference, reported to 100 g, between 100 g and the sum of moisture content and °Brix concentration), moisture content, and temperature was made using a 3rd grade polynomial equation with seven variables (according to ). The equation of the model is as follows (Eq. 20):
The coefficient of regression of the proposed model represents 99.71 (R2 adjusted 99.50). The model is a significant one (P = 0.001). In , the measured and predicted values of loss modulus are plotted.
CONCLUSIONS
Spanish honeys present a Newtonian behaviour, while from the viscoelastic point of view it behaves like a liquid with loss modulus much greater than the elastic modulus in the domain of the temperature used (5–40°C). The viscosity, loss, and modulus are influenced negatively by the increasing of the temperature, and positively by °Brix concentration. The exponential models are much more suitable for predicting the influence of °Brix concentration on loss modulus and viscosity. In the case of viscosity and loss modulus, a model of the influence of temperature and °Brix concentration was obtained. Based on the experimental design results, the cubic polynomial models with seven variables were developed for predicting the loss modulus as a combination of design variables, namely fructose, glucose, sucrose, sugars, non-sugars, moisture content, and temperature. The polynomial models were statistically validated by F-ratio test.
REFERENCES
- Kaya, A.; Ko, S.; Gunasekaran, S. Viscosity and color change during in situ solidification of grape pekmez. Food Bioprocess Technology 2008, 4 (2), 241–246.
- Bhandari, B.; D’Arcy, B.; Chow, S. Rheology of selected Australian honeys. Journal of Food Engineering 1999, 41 (1), 65–68.
- Chen, Y.W.; Lin, C.H.; Wu, F.Y.; Chen, H.H. Rheological properties of crystallized honey prepared by new type of nuclei. Journal of Food Processing Engineering 2009, 32, 512–527.
- Kang, K.M.; Yoo, B. Dynamic rheological properties of honeys at low temperatures as affected by moisture content and temperature. Food Science and Biotechnology 2008, 17 (1), 90–94.
- Yanniotis, S.; Skaltsi, S.; Karaburnioti, S. Effect of moisture content on the viscosity of honey at different temperatures. Journal of Food Engineering 2006, 72 (4), 372–377.
- Kumar, J.S.; Mandal, M. Rheology and thermal properties of marketed Indian honey. Nutrition & Food Science 2009, 39 (2), 111–117.
- Oroian, M.; Amariei, S.; Escriche, I.; Gutt, G. Rheological aspects of Spanish honeys. Food Bioprocess Technology 2013, 6 (1), 228–241.
- Oroian, M. Physicochemical and rheological properties of Romanian honeys. Food Biophysics 2012, 7 (4), 296–307.
- Oroian, M.; Amariei, S.; Escriche, I.; Gutt, G. A viscoelastical model for honey using the time-temperature superposition principle (TTSP). Food Bioprocess Technology 2012. DOI:10.1007/s11947-012-0893-7.
- Cohen, I.; Weihs, D. Rheology and microrheology of natural and reduced-calorie Israeli honeys as a model for high-viscosity Newtonian liquids. Journal of Food Engineering 2010, 100 (2), 366–371.
- Witczak, M.; Juszcak, L.; Galkowska, D. Non-Newtonian behaviour of heather honey. Journal of Food Engineering 2011, 104 (1), 532–537.
- Gómez-Díaz, D.; Navaza, J.M.; Quintáns-Riveiro, L.C. Rheological behaviour of Galician honeys. European Food Research and Technology 2006, 222, 439–442.
- Gómez-Díaz, D.; Navaza, J.M.; Quintáns-Riveiro, L.C. Physicochemical characterization of Galician honeys. International Journal of Food Properties 2012, 15, 292–300.
- Mora-Escobedo, R.; Moguel-Ordonez, Y.; Jaramillo-Flores, M.E.; Gutierrez-Lopez, G.F. The composition, rheological and thermal properties of tajonal (Viguiera dentata) Mexican honey. International Journal of Food Properties 2006, 9 (2), 299–316.
- Bhandari, B.; D’Arcy, B.; Kelly, C. Rheology and crystallization kinetics of honey: Present status. International Journal of Food Properties 1999, 2 (3), 217–226.
- Mossel, B.; Bhandari, B.; D’Arcy, B.; Caffin, N. Determination of viscosity of some Australian honeys based on composition. International Journal of Food Properties 2003, 6 (1), 87–97.
- Zaitoun, S.; Ghzawi, A.A.; Al-Malah, K.I.M.; Abu-Jdayil, B. Rheological properties of selected light colored Jordanian honey. International Journal of Food Properties 2001, 4 (1), 139–148.
- Yoo B. Effect of temperature on dynamic rheology of Korean honeys. Journal of Food Engineering 2004, 65, 459–463.
- Abu-Jdayil, B.; Al-MajeedGhzawi, A.; Al-Malah, K.I.M.; Zaitoun, S.J. Heat effect on rheology of light and dark colored honey. Journal of Food Engineering 2002, 51 (1), 33–38.
- Mossel, B.; Bhandari, B.; D’Arcy, B.; Caffin, N. Use of Arrhenius model to predict rheological behaviour in some Australian honeys. Lebensmittel-Wissenschaft und-Technologie 2000, 33, 545–552.
- Bogdanov S. Harmonised Methods of the International Honey Commission; Swiss Bee Research Centre: Bern, Switzerland, 2002.
- Codex Alimentarius. Standard for Honey, Ref. no. CL 1993/14, SH. Codex Alimentarius Commission FAO/WHO: Rome, Italy, 1993.
- Kucuk, M.; Kolayh, S.; Karaoglu, S.; Ulusoy, E.; Baltaci, C.; Candan, F. Biological activities and chemical composition of three honeys of different types from Anatolia. Food Chemistry 2007, 100, 526–534.
- Doublier, J.L.; Cuvelier, G. Gums and hydrocolloids: Functional aspect. In: Carbohydrates in Food; Eliasson, A.C.; Ed.; Marcel Dekker Inc.: New York, 1996; 283–318.
- Rao, M.A.; Cooley, H.J.; Vizali, A.A. Flow properties of concentrated juices at low temperatures. Food Technology 1984, 38, 113–119.
- Giner, J.; Ibarz, A.; Garza, S.; Xhian-Quan, S. Rheology of clarified cherry juices. Journal of Food Engineering 1996, 30, 147–154.
- Ibarz, A.; Pagán, J.; Miguelsanz, R. Rheology of clarified fruit juices. II. Blackcurrant juices. Journal of Food Engineering 1992, 15, 63–67. DOI:10.1016/0260-8774(92)90040-D.