Abstract
In order to obtain a high purity and high biological activity of mannoprotein from S. cerevisiae, the extraction, structural characterization, and antineoplastic activity of mannoprotein were investigated. The mannoprotein was dealt with trichloroacetic acid to remove the protein, and then purified by gel filtration chromatography to the purity of 92.61 ± 0.42%. The molecular weight of the mannoprotein was determined to be 166–700 Da by GPC-LLS. Structural analysis revealed that the mannoprotein was a glycoprotein. The mannan consisted of α-1, 6-glycosidic linkages as the main chains, while some α-1, 2-glycosidic linkages existed as the side chains, and the protein consisted of 17 kinds of amino acids. The atomic force microscopy results showed mannoprotein was composed of many conglobulations. S. cerevisiae mannoprotein exhibited significantly higher inhibition ratios of HepG2, HL-60, and Eca109 cells than degraded S. cerevisiae mannoprotein by proteolytic enzymes.
INTRODUCTION
Mannose polymers, or mannan, are found only as part of a glycoconjugate in S. cerevisiae, and not as an unconjugated oligosaccharide. Because of this, the terms “mannan” and “mannoprotein” have often been used interchangeably in the S. cerevisiae literature. However, to maintain clarity, in this study “mannan” refers to the glycan itself while mannoprotein refers to glycoprotein.[Citation1] S. cerevisiae mannoprotein occupied about 35–45% of the outer layer of the yeast cell wall, which could not only provide cells with rigidity that protect them from osmotic pressure but also help to maintain their shapes, allowing them to change their shape in keeping with the cell cycle stage.[Citation2,Citation3] Mannan is generally linked to protein through covalent bond and is composed of 5–20% protein and 80–90% mannose, with a molecular weight from 20,000 to 200,000 Da. The backbone, composed of α-1, 6-linked mannose residues, was found to be 83% branched at O-2 by single mannose residues as well as oligosaccharide side chains mostly in the form of tri-, di-, mono-, and tetramers. Longer side chains, penta- to heptamers, were present in lesser amounts. Nuclear Magnetic Resonance (NMR) spectra of oligosaccharides showed that they were formed mostly by a-1, 2- and a-1, 3-linked mannose residues.[Citation4]
S. cerevisiae mannoprotein has emulsifying properties that could be of commercial significance.[Citation5] Besides, mannoprotein was found to be the most significant of the immunological effects, which were shown to be potent inducers of cellular and humoral immunity.[Citation6] Moreover, it could also act as the antineoplastic, anti-oxidant modulation of intestinal flora and radiation protection. In the past decades, mannoprotein has often been isolated by acid-alkaline methods,[Citation7–Citation10] but the native structure of the mannoprotein was damaged by acids and alkalis in these methods. Besides, enzymes such as laminarinase and protease were used to isolate the mannoprotein from yeast cell wall by Johanna et al.[Citation11] and Franziskus and Werner,[Citation10] but the enzyme was expensive. Recently, a water extract method with high temperatures was used as a non-degrading isolation process for mannoprotein reported by Young et al.,[Citation12] Stefan et al.,[Citation13] Franziskus and Kulick,[Citation14] and Ližičárová et al.[Citation15]
The structure and molecular weight of mannoprotein were affected by the extract methods. The objectives of the present study were to improve the extract and purification method to obtain purified mannoprotein, characterize the structure of the mannan by NMR and Atomic Force Microscope (AFM) analysis, and then investigate the antineoplastic activities of mannoprotein and mannan. This research was basic for the study of structure and bioactivity relationships in the future.
MATERIALS AND METHODS
Materials
Spent brewer’ s yeast slurry (a strain of S. cerevisiae), a by-product from brewery with a solids content of about 18%, was kindly provided by Lvxian Biology and Technology Co., Ltd., Hebei, China.
Extraction of S. cerevisiae Mannoprotein
Extraction of mannoprotein from S. cerevisiae was carried out using the method of Liu et al.[Citation16] Spent brewer’s yeasts were first sieved (mesh diameter 125 mm) and purified, then stored at 4°C until used. The spent yeast cells slurry (adjusted to 15% w/w solids content and pH 5.0) was added to 3% sodium chloride as the autolysis promoter, then incubated at 55°C for 24 h with agitation at 120 r/min. The autolysate centrifuged at 4200 r/min for 10 min to separate the residual autolyzed cells from yeast extractions at room temperature. The autolyzed yeast cells were adjusted to 10% w/w solids with about 50 g of glass beads (diameter 3–4 mm) in a 500-ml conical flask. The suspension was heated to 121°C in an autoclave (YXQ-LS-30S11, China) for 4 h and then cooled to 45°C; it was centrifuged at 4200 r/min for 10 min, then the supernatant was collected. The residues were washed with distilled water and then re-centrifuged (4200 r/min, 10 min). The supernatant was mixed with the supernatant before and concentrated to one-fifth of the original volume. After that, triple absolute alcohol was added to the water extract and kept at 4°C for one night. The precipitated mannoprotein was centrifuged at 8000 r/min for 10 min, the supernatant liquid was discarded, and the sediment was dissolved in water.
Purification of S. cerevisiae Mannoprotein
The protein in polysaccharide was precipitated by trichloroacetic acid, and then centrifuged at high speed.[Citation13,Citation16] The supernatant was mixed with three volumes of dehydrated ethanol (ethanol final concentration, 75%) and kept overnight at 4°C. Then, the solution was centrifuged at 4200 r/min for 20 min, the precipitate was washed twice with absolute ethanol and once by ether, then dried at 70°C. After that, the mannoprotein was purified by a combined column system according to the gel filtration chromatography. For this purpose, Sepharose CL-4B as material was used to separate the mannoprotein, glucan, free protein, etc. The eluent used was deionized water containing 200 ppm NaN3 to protect the apparatus against microbial attack.[Citation17]
Chemical Analysis Methods
Total nitrogen was measured by a Kjeldahl analyzer (Foss-2300 Kjeltec, Sweden). Crude protein content was calculated by the total nitrogen multiplying by 6.25. Soluble protein content was determined according to the procedure of Lowry, which had been described by Peterson.[Citation18] Total fat and ash content were determined by AOAC Official Methods.[Citation19] Fat was determined by a Soxhlet extraction analyzer (Foss-2050 Soxtec, Sweden) using petroleum ether as the organic solvent. Ash was determined by incinerating dried samples at 600°C for about 5 h in a furnace (Lindberg/Blue M, USA). Total carbohydrate was measured by the phenol-sulfuric acid method with 6.0% (w/v) phenol and assayed at 490 nm.[Citation20]
β-Glucan and mannan were determined according to Dallies et al.[Citation21] and Liu et al.[Citation22] Polysaccharides (20 mg) were wetted with 100 μl of 72% (w/w) H2SO4 and left at room temperature for 3 h. The slurry was diluted to 1 ml to a final concentration of 2 N-H2SO4 and heated in sealed tubes for 4 h at 100°C. Sulfate ions were neutralized by drop-wise addition of NaOH until neutral pH was reached (checked with pH paper). The volume was adjusted to 100 ml with a phosphate buffer pH of 7.0. The monosaccharide was reacted with glucose oxidase and peroxidase (GOPOD), and then analyzed by spectrophotometer.[Citation17,Citation23] Glucose and mannose were used as standard in the experiments.
Molecular and Structural Characterization
Molecular weight determination
The molecular weight distribution was determined using a high-performance size-exclusion chromatograph (HPSEC) apparatus (Wyatt Technology, USA) equipped with multiple detectors: a differential pressure viscometer (DP) for viscosity determination, a refractive index detector (RI) and a UV detector for concentration determination, and a right angle laser light scattering detector (RALLS) and a low angle laser light scattering detector (LALLS) for direct molecular weight determination. The column was a TSK GEL G4000 PWXL (Showa Denko K.K., Tokyo, Japan). The columns, viscometer, and RI detector were maintained at 25°C. The eluent was 0.1 M NaNO3 containing 0.03% (w/w) NaN3 at a flow rate of 0.6 mL/min. Data were obtained and analyzed using the OmniSEC 4.6.1 software.[Citation24−Citation27]
FTIR analysis
FTIR spectra were obtained using a FTS 7000 Fourier transform infrared spectrometer equipped with a DTGS detector (DIGILAB, Randolph, MA, USA). The spectra were recorded at the absorbance mode from 4000 to 400 cm−1 (mid infrared region). To avoid the interference of water, all samples were first dried in a vacuum oven at 80°C for 3 h, and then moved to a vacuum desiccator containing a desiccant and kept for 24 h before the measurement.[Citation28,Citation29]
NMR analysis
S. cerevisiae mannoprotein was freeze-dried three times from D2O (99.96%, Sigma Chemical Co. Ltd., UK) and finally dissolved in 1 ml D2O. Quantitatively valuable 1H NMR spectra and 13C NMR spectra were obtained using an AVANCE-DPX400 NMR spectrometer (Bruker, Switzerland) at 25°C.[Citation30,Citation31]
Amino acid analysis
Polysaccharide was hydrolyzed with 2 N hydrochloric acid for 24 h at 110°C. After cooling and filtration, the filtrate was dried in vacuum, and 0.02 N hydrochloric acid was added and reacted in atmosphere for 30 min. Next, 50 μl of hydrolyzed polysaccharide was measured at 570 and 440 nm (to examine Pro and Pro-OH) with a temperature program of 53°C, pressure of 80–130 kg/cm2, and flow rate of 0.225 ml/min.[Citation32]
Atomic Force Microscope analysis
The sample was dissolved in deionized water and diluted to 1.0 × 10−2 mg/ml; then 5 μl of solution was dripped on the fresh mica and naturally aired. A Si3N4 probe and TAPPING mode were used and observed by Atomic Force Microscope analysis (Digital Instrument, USA).[Citation32]
In Vitro Antineoplastic Activity
MTT (3-(4,5-dimethylthiazol-2-yl)-2,5-diphenyl tetrazolium bromide) is commonly used to measure the number of metabolically active cells in vitro assays. It is based on the conversion of yellow water-soluble MTT to the purple water insoluble end-product formazan by mitochondrial dehydrogenase. The amount of formazan formed is proportional to the number of metabolically active cells.[Citation33,Citation34] The cancer cells, which were collected and dissolved in RPMI-1640 (Roswell Park Memorial Institute-1640), included 10% serum bovine calf to make 1 × 104 /ml cells suspension, inoculated in 96-well round-bottom plates, and 100 μl to each pore. After culturing at 37°C, 5% CO2 for 24 h, mannoprotein was added and cultivated for 3 days. Then, the supernatant was thrown away and 10 μl MTT was added to each pore and cultivated in RPMI media 1640 for 4 h. Next, 100 μl complete cell lysis solution was injected to each pore. After reacting for 24 h, the absorbance (A) at 450 nm test wavelength was measured by ELISA (enzyme-linked immunosorbent assay, Bio-Tek MQX200). The in vitro results, expressed as the inhibition ratio of tumor cell proliferation, were calculated as:
Statistical Analysis
Statistical analysis was conducted using the statistical analysis system (SAS) program. The results were presented as mean values ± standard deviation of at least four experiments. Paired t-test (levels of significance: 0.01 or 0.05) was used to evaluate the statistical significance of differences with p < 0.01 or 0.05, which were considered statistically significant.
RESULTS AND DISCUSSION
Purification of S. cerevisiae Mannoprotein
Extraction of mannoprotein from S. cerevisiae was carried out using the method of Liu et al.,[Citation16] and then, mannoprotein was purified in this study. The protein can precipitate at isoelectric point by trifluoroacetic acid (TCA), and it can be adsorbed by the active carbon or bentonite; besides, albuminous degeneration can be caused by organic solvent, sodium chloride, calcium chloride, and/or enzyme.[Citation36,Citation37] Thus, the protein in mannoprotein (20.13%) was investigated to be removed in this study by the methods mentioned above. The results in showed that the removal rate of protein by TCA and enzyme was higher than other methods; the removal rate was 70.94 and 75.42%, respectively. TCA was determined to remove the protein considering the higher cost of enzyme.
The ethanol was added slowly to the supernatant under constant stirring in a water bath at 25°C to bring the ethanol concentration to 10% (v/v). The solution was allowed to stand for 4 h at 4°C, and the precipitate was collected by centrifuging at 20,000× g at 4°C for 20 min. Then, the concentration of ethanol in supernatant was increased stepwise from 10% to a final concentration of 90%. The corresponding fractions at each step were obtained in a similar manner as described above. Little mannanoprotein was deposited at the ethanol concentration of 10%, and 79.20% mannanoprotein was deposited when the ethanol concentration reached 30%. Most of the mannanoprotein was deposited when the concentration of ethanol reached 50%. It indicated a fairly narrow Mw distribution of mannanoprotein, and the ethanol concentration was determined to 50%. The yield of carbohydrate was 5.63 ± 0.28%, the contents of mannanoprotein and impurity protein were 83.92 ± 0.42% and 11.51 ± 0.42%, respectively.
TABLE 1 Effect of different methods on deproteinization and polysaccharide loss
The mannoprotein was purified according to gel filtration chromatography using the material of Sepharose CL-4B. Results were shown in . The protein was divided into two parts, and the second part was overlapped to the polysaccharide. It was concluded that the protein related to the second part was linked to the polysaccharides and belonged to a glycoprotein. The eluents to the second peak were collected and freeze-dried, and the polysaccharide content in it was 92.60 ± 0.32%.
Yeast and Mannanoprotein Composition Analysis
The compositions of yeast and mannanoprotein were presented in . The yeast cell wall occupied 23.52% of the yeast cells, the mannanoprotein occupied 10.43% of the yeast, and the mannanoprotein occupied 35.41% of the yeast cell wall. After hot water extraction, the yield and the purity of the crude mannanoprotein were 8.65 and 65.05%, respectively. After the removal of the free protein deposited by ethanol, the yield of mannanoprotein declined to 6.42% and the purity reached 83.92%. Then, gel filtration chromatography was used to further purify the mannanoprotein; finally, the yield and the purity of the mannanoprotein reached 5.40 and 92.61%.
TABLE 2 Yeast and mannoprotein compositions
Structural Characterization of S. cerevisiae Mannanoprotein
Molecular weight measurement
The bioactivity of polysaccharides was affected significantly by molar mass; for this reason, the molecular weight distribution of S. cerevisiae mannanoprotein was measured by GPC-LLS in this study. The molecular weight (Mw) was 166,700 Da and the radius of gyration was 20.7 nm. The polydispersity index (Mw/Mn, where Mn is the number average molecular weight) of 1.350 indicated a fairly narrow Mw distribution of mannanoprotein. Franziskus and Kulick[Citation14] reported that chemically extracted mannanoprotein had molar masses from 21,000 to 62,000 Da, whereas enzymatically isolated mannanoprotein had molar masses between 64,000 and 112,000 Da. Thus, we can conclude that using the hot water extract method can protect the original structure of mannanoprotein from degradation by acid or alkali.
FTIR spectroscopic identification
FTIR spectroscopic investigations were employed to identify and inspect the purity of the isolated mannan fractions. The IR spectrum of a mannan is shown in . The OH valence vibrations appeared at 3400 cm−1. The band at 2900/2930 cm−1 was the typical of CH valence vibrations, and the band at 1645/1652 cm−1 was the typical of acylamino, which showed that mannanoprotein was a glycoprotein. The C=O asymmetric stretching vibration appear at 1635/1645 cm−1; the CH deformation vibrations occur at 1454/1460 cm−1. The C=O valence vibration is found at 1000/1100 cm−1, which showed the existence of the pyranoid ring characteristic absorption peak. The band at 820 cm−1 is particularly characteristic of mannan; this was in accordance with the result reported by Franziskus and Werner.[Citation10]
NMR spectroscopic identification
The composition of mannan can be identified by 1H NMR. The resonances of polysaccharides lie in the range of 4.0–5.5 ppm. The anomeric H atoms were assigned on the basis of published data for mannan, according to the results of periodic acid oxidation and Smith degradation. shows the stretching of the signals of the anomeric H atoms. The signal at 5.3 ppm is typical of mannose in α-1, 2-linked side chains of two or more sub-units, and 5.1–5.2 ppm is the typical of α-1, 6-mannan in the basic chain on which the mannose side groups are suspended. Furthermore, 5.02 ppm is the signal for terminal α-1, 2-linked mannose and for α-1, 2-linked mannose with a mannose substituent in the 3 position. This was in accordance with the report of Franziskus and Kulick.[Citation14]
FIGURE 3 (A) 1H NMR spectra of S. cerviseae mannoprotein. (B) 13C NMR spectra of S. cerviseae mannoprotein.
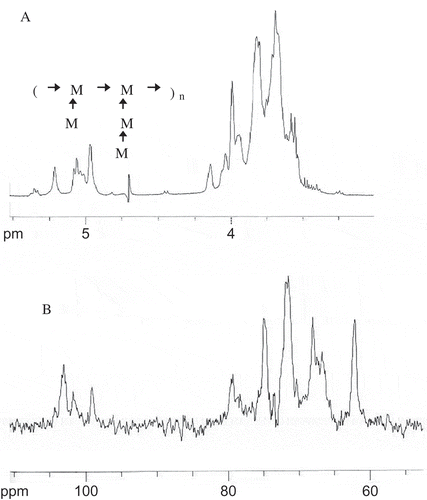
The mannan could also be identified in the 13C spectrum (). Chemical shifts between 98 and 103 ppm were a typical feature of the C-1 atoms in α-glycosidic linkages. There was no resonance signal in 82–88 ppm, so we could conclude that the sugar residues were mannopyranose. The signal at 76–80 ppm could be assigned to the substituted C-2, C-3, or C-4 atoms; the signal at 70 ppm could be assigned to the substituted C-6 atom; and the signal at 61 ppm could be assigned to the unsubstituted C-6 atom. Further assignment was not carried out.
Amino acid analysis
The composition analysis manifested that mannoprotein was a glycoprotein, so amino acid analysis was used in this study (). The results showed that mannoprotein included 17 kinds of amino acids and the ratio of serine, threonine, and aspartic acid were 11.46, 20.08, and 14.96%, respectively, which were higher than other amino acids. This was accorded with the research of Wang et al.[Citation38]
TABLE 3 Amino acid analysis of S. cerviseae mannoprotein
Atomic Force Microscope analysis
The external characteristic of mannoprotein was observed by AFM. From , we could see that the netted texture of mannanoprotein consisted of small round particles and existed as an aggregation, the reports of Nakanishishindo et al.[Citation32] had the same results. The diameter of the aggregation shown in the picture was 0.2–0.3 μm and the height was 1–2 nm. The three-dimensional pictures showed that the mannan aggregated together like some islands, but did not appear to be the same size. This was in accordance with the molecular weight distribution mentioned above.
In Vitro Antineoplastic Activity of S. cerevisiae Mannoprotein
It has been reported that water soluble polysaccharides exhibited antitumor activity containing the backbone with either β-1, 3-linked D-glucose or α-1, 4-linked D-mannan.[Citation39] The mannoprotein and the protein-bound polysaccharides played an important role in the enhancement of antitumor activity both in vivo and in vitro,[Citation40] and that depended on their monosaccharide composition, protein content, molecular mass, and chain conformation.[Citation41−Citation43] However, the mannoprotein or mannan, which played an important role, had not been reported so the antitumor activities of S. cerevisiae mannoprotein and degraded S. cerevisiae mannoprotein by proteolytic enzymes were compared in this study.
S. cerevisiae mannanoprotein was dissolved in phosphate buffer (pH 7.0) to the concentration of 15% (w/w), papain was added to hydrolyze the mannoprotein at 55°C for 5 h, and then pronase was used to hydrolyze the mannoprotein at pH 7.2, 37°C for 48 h. After inactivation of the enzyme and dialyzing for 2 days, degraded S. cerevisiae mannoprotein was obtained by freeze drying. The protein content was reduced from 5.20 ± 0.08% to 0.86 ± 0.02% at last.
After incubated with S. cerevisiae mannanoprotein and degraded S. cerevisiae mannoprotein for 24 h at the concentrations of 0.25, 1.50, 1.00, and 2.00 mg/ml, the inhibition ratio of HepG2, HL-60, and Eca109 cells was observed and compared with the control. showed that the growth of HepG2, HL-60, and Eca109 cells were significantly inhibited by S. cerevisiae mannoprotein and degraded S. cerevisiae mannoprotein mannan at the concentrations from 0.25 to 2.00 mg/ml. S. cerevisiae mannoprotein exhibited significantly higher inhibition ratios than degraded S. cerevisiae mannoprotein. Especially, at the S. cerevisiae mannoprotein concentration of 2.00 mg/ml, the inhibition activity of HepG2, HL-60, and Eca109 was the highest with an inhibition ratio beyond 33.57 ± 1.27%, 55.05 ± 0.83%, and 34.02 ± 1.26%, respectively. From the above, we can conclude that except β-1, 3-linked D-glucose and α-1, 4-linked D-mannan, α-1, 6-mannan in the basic chain with α-1, 2-linked side chains also having antitumor activity, and the protein bounded to mannan played an important role in the enhancement of antitumor activity.
FIGURE 5 (A) The inhibition ratio of S. cerevisiae mannoprotein concentration effect to HepG2. (B) The inhibition ratio of S. cerevisiae mannoprotein concentration effect to HL-60. (C) The inhibition ratio of S. cerevisiae mannoprotein concentration effect to Ecal09. SMP: S. cerevisiae mannoprotein; DSMP: Degraded S. cerevisiae mannoprotein; IR: Inhibition ratio.
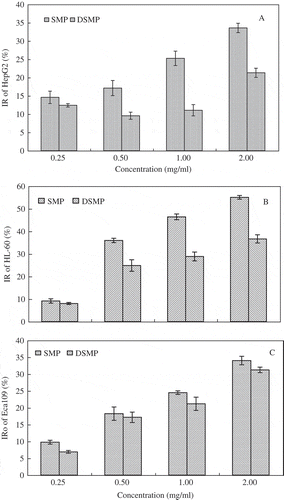
CONCLUSION
A series of isolation and depuration methods were used to obtain the high purity S. cerevisiae mannoprotein, the contents of mannan and protein were 92.61 ± 0.42% and 5.20 ± 0.08%, respectively. The molecular weight (Mw) of the purified mannoprotein got 166,700 with a narrow Mw distribution (polydispersity is Mw/Mn 1.350). Structural analysis revealed that the mannoprotein was a glycoprotein, and the mannan was composed by α-1, 6-glycosidic linkages as the main chains and there also existed some α-1, 2-glycosidic linkages as the side chains. Amino acid analysis showed that mannoprotein included 17 amino acids, and the ratio of serine, threonine, and aspartic acid were higher than others. It was observed that the netted texture of mannan consisted of little round particles and existed as an aggregation. S. cerevisiae mannoprotein exhibited significantly higher inhibition ratios of HepG2, HL-60, and Eca109 cells than degraded S. cerevisiae mannoprotein.
FUNDING
This work was supported by National Natural Science Foundation of China (No. 31171651) and by the Foundation of National and international science and technology cooperation projects (No. 2012DFA31400).
REFERENCES
- Masuoka, J. Surface glycans of Candida albicans and other pathogenic fungi: Physiological roles, clinical uses, and experimental challenges. Clinical Microbiology Reviews 2004, 17, 281–310.
- Ballou, C.E. Isolation, characterization and properties of Saccharomyces cerevisiae mannan mutants with non-conditional protein glycosylation defects. Methods in Enzymology 1990, 185, 440–470.
- Inoue, S.B.; Takewaki, N.; Takasuka, T.; Mio, T.; Adachi, M.; Fujii, Y.; Miyamoto, C.; Arisawa, M.; Furuichi, Y.; Watanabe, T. Characterization and gene cloning of 1, 3-β-D-glucan synthase from Saccharomyces cerevisiae. European Journal of Biochemistry 1995, 231, 845–854.
- Nakajima, T.; Ballou, C.E. Structure of the linkage region between the polysaccharide and protein parts of Saccharomyces cerevisiae mannan. Journal of Biological Chemistry 1974, 249, 7685–7694.
- Vasallo, M.C.; Puppo, M.C.; Palazolo, G.G.; Otero, M.A.; Beress, L.; Wagner, J.R. 2006. Cell wall proteins of Kluyveromyces fragilis: Surface and emulsifying properties. LWT–Food Science and Technology 2006, 39, 729–739.
- Pontón, J.; Omaetxebarra, M.J.; Elguezabal, N.; Alvarez, M.; Moragues, M.D. Immunoreactivity of the fungal cell wall. Medical Mycology 2001, 39, 101–110.
- Roelofsen, P.A.; Hoette, I. Chitin in the yeast cell walls. Antonie van Leeuwenhoek 1951, 17, 297–313.
- Miller, M.W.; Phaff, H.J. On the cell wall composition of the apiculate yeasts. Antonie van Leeuwenhoek 1958, 24, 225–240.
- Díaz, S.; Zínker, S.; Ruiz-Herrera, J. Alterations in the cell wall of Saccharomyces cerevisiae induced by the alpha sex factor or a mutation in the cell cycle. Antonie van Leeuwenhoek 1992, 61, 269–276.
- Franziskus, K.; Werner, M.K. Mild enzyme isolation of mannan and glucan from yeast Saccharomyces cerevisiae. Die Angewandte Makromolekulare Chemie 1999, 268, 59–68.
- Rinsum, J.V.; Klis, F.M.; Ende, H.V.D. Cell wall glucomannoproteins of Saccharomyces cerevisiae. Yeast 1991, 7, 717–726.
- Young, M.; Haavik, S.; Smestad, P.B. Further characterization of a high molecular weight glycoprotein antigen from the yeast Saccharomyces cerevisiae. Carbohydrate Polymers 1996, 30, 243–252.
- Stefan, F.; Martin, S.; Othmar, K. A new non-degrading isolation process for 1,3-β-D-glucan of high purity from baker’s yeast Saccharomyces cerevisiae. Carbohydrate Polymers 2003, 54, 159–171.
- Franziskus, K.; Kulick, W.M. Polymer analytical characterization of glucan and mannan from yeast Saccharomyces cerevisiae. Die Angewandte Makromolekulare Chemie 1999, 268, 69–80.
- Ližičárová, L.; Matulová, M.; Capek, P. A cell wall mannan with a high content of β-1,2-linked mannose residues. Carbohydrate Polymers 2007, 70, 89–100.
- Liu, X.Y.; Wang, Q.; Cui, S.W.; Liu, H.Z. A new isolation method of β-D-glucans from spent yeast Saccharomyces cerevisiae. Food Hydrocolloids 2008, 22, 239–247.
- Li, J.W.; Xiao, N.; Yu, R.Y.; Yuan, M.X. Biochemistry Experiment Elements and Methods. Beijing University: Beijing, China, 1994.
- Peterson, G.L. A simplification of the protein assay method of Lowry et al. which is more generally applicable. Analytical Biochemistry 1977, 83, 346–356.
- AOAC. Methods 920.39, 942.05. In: Official Methods of Analysis, 15th Ed.; Association of Official Analytical Chemists: Arlington, VA, 1990.
- Dubois, M.; Gilles, K.A.; Hamilton, J.K.; Rebers, P.A.; Smith, F. Colorimetric method for determination of sugars and related substances. Analytical Chemistry 1956, 28, 350–356.
- Dallies, N.; Francois, J.; Paquet, V. A new method for quantitative determination of polysaccharides in the yeast cell wall. Application to the cell wall defective mutants of Saccharomyces cerevisiae. Yeast 1998, 14, 1297–1306.
- Liu, H.Z.; Wang, Q.; Liu, X.Y.; Tan, S.S. 2008. Effects of spaceflight on polysaccharides of Saccharomyces cerevisiae cell wall. Applied Microbiology Biotechnology 2008, 81, 543–550.
- Zhang, Y.T.; Gu, W.Y. Determination of mannose in yeast by ultraviolet spectrometry. Food and Fermention Industry 1999, 5, 32–36.
- Guo, Q.B.; Cui, S.W.; Wang, Q.; Hu, X.Z.; Kang, J.; Yada, R.Y. Structural characterization of a low-molecular-weight heteropolysaccharide (glucomannan) isolated from Artemisia sphaerocephala Krasch. Carbohydrate Research 2012, 350, 31–39.
- Li, W.; Cui, S.W.; Kakuda, Y. Extraction, fractionation, structural and physical characterization of wheat β-D-glucans. Carbohydrate Polymers 2006, 63, 408–416.
- Li, W.; Cui, S.W.; Wang, Q.; Yada, R.Y. Studies of aggregation behaviours of cereal β-glucans in dilute aqueous solutions by light scattering: Part I. Structure effects. Food Hydrocolloids 2011, 25, 189–195.
- Gujral, H.S.; Sharma, P.; Kaura, H.; Singh, J. Physiochemical, pasting and thermal properties of starch isolated from different barley cultivars. International Journal of Food Properties 2013. DOI: 10.1080/10942912.2011.595863.
- Bai, L. Analysis of amino acids in protein of shiitake [Lentinus edodes (Berk.) Singer]. Journal of Fungal Research 2006, 2, 21–24.
- Wang, X.M.; Sun, R.G.; Zhang, J.; Chen, Y.Y.; Liu, N.N. Structure and antioxidant activity of polysaccharide POJ-U1a extracted by ultrasound from Ophiopogon japonicas. Fitoterapia 2012, 83, 1576–1584.
- Abeygunawardana, C.; Williams, T.C.; Sumner, J.S.; Hennessey Jr, J.P. Development and validation of an NMR-based identity assay for bacterial polysaccharides. Analytical Biochemistry 2000, 2, 226–240.
- Ovchinnikova, O.G.; Kocharova, N.A.; Kondakova, A.N.; Kokot, M.B.; Shashkov, A.S.; Knirel, Y.A.; Rozalski, A. Structure of the O-polysaccharide from the lipopolysaccharide of Providencia alcalifaciens O28. Carbohydrate Polymers 2011, 346, 2638–2641.
- Nakanishishindo, Y.; Nakayama, K.; Tanaka, A. Structure of the N-linked oligosaccharides that show the complete loss of alpha-1, 6-polymannose outer chain from och1, och1 mnn1, and och1 mnn1alg3 mutants of Saccharomyces cerevisiae. Journal of Biological Chemistry 1993, 268, 26338–26345.
- Wong, K.H.; Lai, C.K.M.; Cheung, P.C.K. 2010. Immunomodulatory activities of mushroom sclerotial polysaccharides. Food Hydrocolloids 2010, 2, 150–158.
- Zhao, T.; Mao, G.H.; Mao, R.W.; Zou, Y.; Zheng, D.H.; Feng, W.W.; Ren, Y.N.; Wang, W.; Zheng, W.; Song, J.; Chen, Y.Q.; Yang, L.Q.; Wu, X.Y. Antitumor and immunomodulatory activity of a water-soluble low molecular weight polysaccharide from Schisandra chinensis (Turcz.) Baill. Food and Chemical Toxicology 2013, 55, 609–616.
- Soto, B.L.; Hank, J.A.; Darjatmoko, S.R.; Polansc, A.S.; Yanke, E.M.; Rakhmilevich, A.L.; Seo, S.; Kim, K.M.; Reisfeld, R.A.; Gillies, S.D.; Sondel, P.M. Anti-tumor and immunomodulatory activity of resveratrol in vitro and its potential for combining with cancer immunotherapy. International Immunopharmacology 2011, 11, 1877–1886.
- Li, F.; Liu, Y.J.; Zong, X.Y.; Yu, R.H. Study on elimination protein from the roots of Aralia continentalis Kitag polysaccharide. Journal of Anhui Agricultural Sciences 2006, 1, 9–10.
- Huang, C.H.; Ma, C.H.; Song, H.Z.; Li, T.T. Study on deproteinization and decoloration in extraction of Armillariella polysaccharide. Pharmaceutical and Clinical Research 2007, 15, 45–46.
- Wang, H.B.; Xie, B.J.; Liu, D.C. Separation, purification and structural analysis of β-glucan from oat. Food Science 2005, 26, 90–93.
- Peng, Y.F.; Zhang, L.; Zeng, F.B.; Xu, Y.X. Structure and antitumor activity of extracellular polysaccharides from mycelium. Carbohydrate Polymers 2003, 54, 297–303.
- Peng, Y.F.; Zhang, L.N.; Zeng, F.B.; Kennedy, J.F. Structure and antitumor activities of the water-soluble polysaccharides from Ganoderma tsugae mycelium. Carbohydrate Polymers 2005, 59, 385–392.
- Bland, E.J.; Keshavarz, T.; Bucke, C. The influence of small oligosaccharides on the immune system. Carbohydrate Research 2004, 339, 1673–1678.
- Zhang, B.Z.; Yan, P.S.; Chen, H.; He, J. Optimization of production conditions for mushroom polysaccharides with high yield and antitumor activity. Carbohydrate Polymers 2012, 87, 2569–2575.
- Gan, D.; Ma, L.P.; Jiang, C.X.; Xu, R.J.; Zeng, X.X. Production, preliminary characterization and antitumor activity in vitro of polysaccharides from the mycelium of Pholiota dinghuensis Bi. Carbohydrate Polymers 2011, 84, 997–1003.