Abstract
Oxidative stabilities represented with variance of fatty acids, tocopherols, acid value, peroxide value, and thiobarbituric acid value of edible vegetable oils with limited air were investigated. The total tocopherol contents were positively correlated with the polyunsaturated fatty acids (PUFA, r2 = 0.856), negatively correlated with the monounsaturated fatty acids (MUFA, r2 = −0.598), and saturated fatty acids (SFA, r2 = −0.258) in initial oxidation. The residual contents of tocopherols had good negative linear correlations with the peroxide value and thiobarbituric acid value during oxidation. When α-tocopherol predominated over other homologue, it presented a pro-oxidant effect at high concentration, but would transform to be an antioxidant as its concentration decreased to the inflection point that corresponded to the inflection point of peroxide value increase for different kinds of vegetable oils. However, the γ- or δ-tocopherol did not show pro-oxidant action. Therefore, the influence of α-tocopherol on oxidation was likely more predominant than fatty acids were.
INTRODUCTION
Vegetable oil contains abundant unsaturated fatty acids (UFA), which are prone to oxidation and produce a series of compounds, such as lipid hydroperoxides, alkyl radicals, aldehydes, alcohols, ketones, lactones, and hydrocarbons.[Citation1] These products are reported to have a negative influence on a human’s physiology. Hydroperoxides play an important role in the tumor-promoting activity, and alkyl radicals can damage DNA.[Citation2] Besides, 4-hydroxy-2-nonenal (HNE) and 4-hydroxy-2-hexenal (HHE) have dramatic effects on L6 muscle cell viability.[Citation3] Some aldehydes are volatile with very low odor and taste thresholds.[Citation4,Citation5] Usually the degradation of oils is not easy to be detected. When people begin to be aware of their deterioration, the oils may be beyond the safety standard of food. A great number of losses occur in the world every year.[Citation6] Hence, oxidative profile of edible oils is significant not only for forecasting the shelf-life of oils, but also for their long-term storage.
The stability of edible oils generally depends on the composition of fatty acids in the triglycerides. Neff et al.[Citation7] reported that the rate of peroxide formation in purified triacylglycerols of sunflower and soybean oils was positively correlated with the average number of double bonds, linolenic acid, and linoleic acid, but negatively correlated with oleic acid after 60°C accelerated oxidation (Schaal oven test). Kamal-Eldin[Citation8] showed that the effect of fatty acids on oxidative stability of vegetables depended mainly on their degree of unsaturation and the position of the double bond within the triacylglycerol molecule. It was reported that vegetable oils with high contents of C18:2 and C18:3 fatty acids were susceptible to oxidation, and the presence of SFA and MUFA could improve oxidative stability.[Citation9] The reason is that PUFA are sensitive to peroxidation, whereas SFA and MUFA are resistant.[Citation10]
Tocopherols as free radical-scavengers, which are able to donate hydrogen atoms to free radicals and convert these free radicals to more stable non-radical products, are the major natural antioxidants in vegetable oils.[Citation11,Citation12] However, they are generally considered as efficient antioxidants in low content, but gradually lose efficacy as their concentrations increase.[Citation13] In other words, they participate in the side reactions, and react with species other than peroxyl radicals.[Citation14] It was still difficult to determine the optimum antioxidant concentrations of tocopherols for vegetable oils.[Citation15]
The oxidation of oils and the antioxidant behavior of tocopherols are very complex. The relationship between fatty acids and tocopherols in vegetable oils during frying or high temperature heating has been discussed by many authors. Simonne and Eitenmiller[Citation16] reported that tocopherols and tocotrienols degraded more rapidly in the oils with a high SFA content during stimulated deep-fat frying. Rossi et al.[Citation17] also found that the more oxidizable the oil was, the more stable was the tocopherol during deep-fat frying. Some other investigators have reported that the composition of fatty acids was more important for the oxidative stabilities of vegetable oils than their tocopherol levels at high temperature heating.[Citation18]
In fact, both UFA and tocopherols are present at the same time with the presence of limited air during the storage of vegetable oils before being consumed, such as the commercial oil status. Which one is the predominant factor between UFA and antioxidant remains controversial. This study was aimed at determining the stability of eight fresh and two oxidized edible vegetable oils with different composition of fatty acids and tocopherols using the Schaal oven test at 62°C for 30 days with limited air.
MATERIALS AND METHODS
Materials
Eight fresh vegetable oil samples were corn oil, sunflower oil, soybean oil, peanut oil, camellia oil, palm oil, blend oil A (blended with 55% soybean oil, 35% rapeseed oil, and 10% perilla oil), and blend oil B (blended with 90% soybean oil and 10% perilla oil). Two oxidized oil samples were perilla oil and rapeseed oil. All samples were purchased from local shops and fresh oil samples were stored at 4°C until analysis. Oxidized oil samples were kept at room temperature for approximately half a year before analysis. Four synthetic antioxidants, butylated hydroxyanisole (BHA), butylated hydroxytoluene (BHT), propylgallate (PG), and tertiary butylhydroqninone (TBHQ), were not detected in all of the samples.
Standards and Chemicals
Standard fatty acid methyl esters (FAME; GLC-463) spiked with a mixture of four positional conjugated linoleic acid (CLA) methyl ester isomers (#UC-59 M) were obtained from Nu-Chek Prep Inc. (Elysian, MN, USA). Tocopherol and 1,1,3,3-tetraethoxypropane (TEP; purity = 97%) standards were purchased from Sigma (Sigma Chemicals, Shanghai, China). Methanol, n-hexane, acetonitrile, and isopropanol of HPLC grade were purchased from Merck (Darmstadt, Germany), and other solvents were of analytical reagent grade.
GC Analysis of Fatty Acids
The fatty acid composition of oil samples was determined with an Agilent 6890N gas chromatograph and reported in relative area percentages. Oils were methylated as described by Cruz-Hernandez et al.[Citation19] The FAMEs were identified using a capillary column of fused silica (100 m × 0.25 mm × 0.2 μm) coated with 100% cyanopropyl polysiloxane (CP-Sil 88, Chrompack, Middelburg, the Netherlands) equipped with a flame ionization detector (FID). Hydrogen was used as the carrier gas with a flow rate of 30 mL min−1. The oven temperature program sustained 86 min in total as follows: the initial temperature of the oven was 45°C for 4 min, increased to 175°C at a rate of 13°C min−1, maintained for 27 min, further raised to 215°C at a rate of 4°C min−1, and finally kept at this temperature for 35 min. The injector and the detector were set at 250°C.[Citation20] Analysis of all peaks was accomplished by comparison of their retention times with FAME standards. All analyses were carried out in triplicate.
Calculation of Oxidative Stability (Cox) of Oils[Citation17]
The Cox value of oils was calculated by the percentage of C18 unsaturated fatty acids, using the formula proposed by Fatemi and Hammond:[Citation21]
HPLC Analysis of Tocopherols
The tocopherol content in each sample was determined according to the method reported by Hu et al.[Citation22] Approximately 1 g of oil sample was put into a volumetric flask and made up to 10 mL with HPLC-grade hexane. After filtering through a 0.45-μm filter, 3 μL of sample was directly injected into an Agilent 1100 series HPLC system equipped with a fluorometric detector. The excitation wavelength was set at 295 nm, and the emission wavelength was set at 325 nm. The column was a Hypersil ODS2 (5 μm, 4.6 × 150 mm). The mobile phase was methanol/water (98:2, v/v), and the flow rate was 0.8 mL min−1. The corresponding standards (α-tocopherol, γ-tocopherol, and δ-tocopherol) for identification and quantification were prepared in hexane solution. The coefficients of variation were 0.9999, 0.9998, and 0.9998, respectively.
Schaal Oven Test Assay
All of the samples (500 g) were put into loosely closed and non-colored glass bottles. In the presence of limited amounts of air, they were kept inside the oven without light and maintained at 62 ± 1°C for 30 days for oxidation. No stirring or shaking was done, but their position in the oven was randomly changed every day. After intervals of 5 days of heating, samples were removed into bottles. Then they were topped immediately with nitrogen, capped tightly, and kept frozen (−20°C) until analysis. Analyses were conducted either on the same day or within 2 d after removal from the oven. According to Arrhenius formula, it was determined that one day of storage of oils in a Schaal oven test at 62°C was the same as 1 month of storage at room temperature.[Citation23]
Determination of Oxidation Products
AOCS official methods were used to determine the contents of free fatty acids (method Cd 3d-63) and hydroperoxides (method Cd 8-53). The concentration of malonyldialdehyde (MDA) was determined by thiobarbituric acid (TBA) and trichloroacetic acid (TCA).[Citation24] Oil samples (5 g) were put into 150-mL volumetric flasks, dissolved in 25 mL of TCA reagent, and shaken in an oscillator with a water bath for half an hour. Then, it was filtered through double filter papers to remove the oil. An aliquot of 5 mL of filtrate was collected, transferred into a dry colorimetric tube, and then made up to a volume of 5 mL with freshly prepared TBA reagent (0.02 M). The mixture was thoroughly mixed, and the tube was placed in a water bath at 90°C for 40 min. Heated samples were cooled and mixed with 5 mL of chloroform. After centrifugation, the absorbance was determined at 532 nm using a visible spectrophotometer (model 722G, Shanghai, China). The calibration curve of TEP (r2 = 0.9997) as a precursor of MDA was established to determine the number of micrograms of MDA per kilogram of oil. The result was expressed as thiobarbituric acid value (TBARS).[Citation25]
Statistical Analysis
All experiments were done in triplicate. Data were expressed as mean values ± standard deviation. One-way ANOVA analysis was performed to compare the variances between mean values using SPSS 13.0 software for Windows. Significant differences between means were determined by Duncan’s multiple range tests. A p-value less than 0.05 was considered statistically significant. A partial process was used to analyze the correlation among groups under the controlling of one variable. Linear regression analysis was performed with the statistical analysis tools in Microsoft Excel.
RESULTS AND DISCUSSION
Fatty Acids
lists the fatty acid compositions of 10 vegetable oil samples. It was found that the oils contained most of the C18 unsaturated fatty acids (C18:1 + C18:2 + C18:3, 52–89%). Besides, sunflower, peanut, palm, camellia, and corn oil samples were very low-linolenate oils (VLLO) and their concentrations of n-3 PUFA were below 1%. Soybean, rapeseed, blend A, and blend B oil samples were low-linolenate oils (LLO) and their concentrations of n-3 PUFA were from 6 to 15%. Perilla oil was a high-linolenate oil (HLO) and its concentration was approximately 50%. The concentrations of SFA in these samples were from 7 to 46%, MUFA concentrations were from 21 to 78%, and PUFA levels were from 9 to 62%. As shown in , there were moderate declines in PUFA (9.57%), n-3 PUFA (14.65%), and n-6 PUFA (9.43%), and the percentage of SFA and MUFA increased 2.92 and 4.39%, respectively, as oxidation days increased. Similar results were also reported in a previous study.[Citation26]
TABLE 1 Compositions of fatty acids (area %) and tocopherols of 10 vegetable oils before Schaal oven test and oxidative stability order at the end
Tocopherols
Vegetable oils contain tocopherols, especially α-, γ-, and δ-tocopherols, as their main antioxidants. showed that soybean oil had the highest total content (the sum of the four homologues) of tocopherols (643.59 mg kg−1 oil) in 10 samples, followed by blend B oil (608.79 mg kg−1 oil) and sunflower oil (575.09 mg kg−1 oil). The total tocopherol content of rapeseed was the lowest (94.60 mg kg−1 oil). For sunflower, peanut, palm, and camellia oil samples, α-tocopherol was the major tocopherol and accounted for more than 88% of the total tocopherol. But the percentages of γ and δ homologues in these oils were quite low. Both α-tocopherol (62%) and γ-tocopherol (30%) were the major tocopherols in corn oil. All three homologues were found in soybean, blend A, and blend B oils. The percentages of δ-tocopherol in these oils were the highest (from 58 to 65%), followed by γ-tocopherol (from 21 to 27%). The oxidized oil samples (rapeseed and perilla oils) consisted of α-tocopherol (30 and 16%), γ-tocopherol (63 and 67%), and δ-tocopherol (7 and 17%), respectively.
Total content of tocopherols and their various homologues tended to decrease during 30 days of oxidation (). The total content of tocopherols in sunflower oil, peanut oil, palm oil, and camellia oil decreased significantly (100%), followed by corn oil (82%), rapeseed oil (79%), and blend B oil (60%). In addition, the content of α-tocopherol decreased more significantly than γ- and δ-tocopherols. It is generally accepted that α-tocopherol had a greater ability for hydrogen donation than the γ- and δ-homologs,[Citation27] and the oxidation rate of α-tocopherol was consistently greater compared to the losses of γ- and δ-homologs.[Citation28] It was found that the contents of α-tocopherol in sunflower, peanut, camellia, and palm oils decreased moderately in the first several days, and declined sharply with prolonged time. Similar results were reported in other studies.[Citation18,Citation29] The behavior of α-homologue presented a phenomenon of pro-oxidant effect at high concentration but it turned to be antioxidant as the concentration decreased.[Citation8] There existed different inflection points of concentration from pro-oxidant action to anti-oxidation in different oil samples (). When the concentration of α-tocopherol in sunflower oil was from 570 to 500 mg kg−1 oil, it presented a pro-oxidant effect. However, it turned to be antioxidant below 500 mg kg−1 oil. The concentrations of pro-oxidant were from 210 to 180 mg kg−1 oil and those of antioxidant were below 180 mg kg−1 oil in peanut oil. The inflection concentration of palm oil was 110 mg kg−1 oil, and that of camellia was 120 mg kg−1 oil. However, the same regulars were not found in oils in which each of γ- and δ-homologue was the main one; therefore, there was not the pro-oxidant effect of each homologue of tocopherol in corn, soybean, blend A, and blend B oils.
FIGURE 2 Changes of tocopherol compounds and peroxide value during accelerated oxidation test (n = 3). Mean values with standard deviations are plotted as lines.
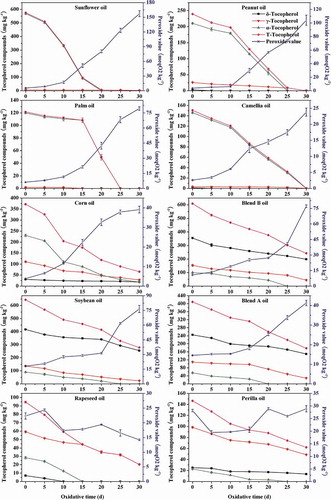
In addition, our study showed that in the initial phase of an oxidation test (about 0–5 days), the total tocopherol levels of eight fresh oils were positively correlated with the sum of PUFA (r2 = 0.86), the Cox value (r2 = 0.87), and negatively correlated with the sum of MUFA (r2 = −0.60) and SFA (r2 = −0.26). And with the degradation of tocopherols (about 10 days later), the correlations between tocopherols and fatty acids were less and less significant.
Acid Value
Free fatty acids were the products of lipid hydrolysis; the amounts of them may be directly converted by means of acid value.[Citation30,Citation31] Rapeseed oil had the highest acid value (5.2 mg KOH g−1) at the beginning of the oxidation test (). The acid values of perilla, camellia, and blend A oils were between 2.5 and 4 mg KOH g−1. The acid values of soybean, peanut, and blend B oils were not high (0.8–1.2 mg KOH g−1), and values of corn, sunflower, and palm oils were very low (0.1, 0.05, and 0.05 mg KOH g−1, respectively). The acid values of 10 oil samples increased continuously with the storage days, but the relative increases were not significant, and the correlations among the increase of acid value during accelerated oxidation, fatty acids, and tocopherols were also not significant (r2 ≪ 0.9). Hence, the acid value was not a significant oxidative evaluation parameter in the presence of limited air.
Peroxide Value
Peroxide values (POV) of eight fresh oil samples increased to different degrees during the 30-day oxidation test (). POV of sunflower oil increased significantly (27.35-fold), followed by peanut oil (26.63-fold) and palm oil (11.78-fold). The increase of POV was in the following order: sunflower oil > peanut oil > palm oil > corn oil > camellia oil > blend B oil > soybean oil > blend A oil. The increase of POV in sunflower oil, peanut oil, palm oil, and camellia oil as VLLO samples was positively correlated with the concentration of n-6 PUFA (r2 = 0.861), the sum of PUFA (r2 = 0.858), and the Cox value (r2 = 0.835), and negatively correlated with the sum of MUFA (r2 = −0.666). In contrast, corn oil as VLLO had a higher content of PUFA than peanut oil and palm oil, but the increase of POV was much less than those of the two oils. Soybean oil, B oil, and A oil were LLO; the ratios of n-6 to n-3 PUFA were between 2 and 7. The correlations among the increase of POV in the three oils and the concentration of PUFA, n-6 PUFA, n-3 PUFA, and MUFA, the ratio of n-6 to n-3 PUFA were not significant (r2 < 0.5). However, the increase of POV was positively and significantly correlated with the Cox value (r2 = 0.955). The Cox values of blend B oil, soybean oil, and blend A oil were 7.59, 7.25, and 6.88, respectively (). The polyunsaturated linoleic (C18:2) and linolenic acids (C18:3) have a higher weight in the Cox calculation than the monounsaturated oleic acid (C18:1), in which oxidation rate is far below those of the other C18 unsaturated acids.[Citation32]
Moreover, the increase of POV was closely related to the decrease of tocopherols. For sunflower oil, peanut oil, palm oil, and camellia oil (with major percentage of α-tocopherol), the decrease rate of α-tocopherol (sunflower oil > peanut oil > palm oil > camellia oil) was corresponded to the order of POV increase. The lower the residual content tocopherol had, the higher the POV of oil was. Verleyen et al.[Citation33] compared the degradation of α-tocopherol after 2 h of heating at 240°C in purified triacylglycerols from nine oils, and obtained a good linear correlation (y = 3.72 x + 137.5, r2 = 0.9463) between the residual tocopherol content (y) and the iodine value (x). Interestingly, we compared the degradation of α-tocopherol in sunflower, peanut, palm, and camellia oils, and found that there was a good negative linear correlation between POV and residual content of α-tocopherol (r2 = −0.9378 - −0.9875) or total tocopherol (r2 = −0.9182 - −0.9872) during the oxidation at 62°C. The two correlation coefficients of corn oil were −0.9070 and −0.9145, respectively. Besides, POV of corn oil also had negative linear correlation with the residual content of γ-tocopherol (r2 = −0.9240). The linear correlation coefficients among POV and residual content of α-, γ-, δ-tocopherols, and total tocopherol for soybean oil were −0.7725, −0.7344, −0.9148, and −0.8361, respectively. The correlation coefficients of blend A were −0.8558, −0.9943, −0.7699, and −0.8944 and of B oil were −0.9852, −0.8783, −0.6270, and −0.7917, respectively. In comparison, the correlation coefficients of these LLO samples between POV and residual content of total tocopherol were lower than those of VLLO.
POV of the above eight fresh oils increased slower at the beginning. As shown in , there also existed inflection points of POV like α-tocopherol in oil samples. When α-tocopherol began to decline sharply, POV increased sharply. For example, POV increased sharply from 9 to 160 meq O2 kg−1 when the concentration of α-tocopherol declined from 500 mg kg−1 oil to zero in sunflower oil. POV of peanut oil increased significantly from 6 to 105 meq O2 kg−1 when α-tocopherol decreased from 180 mg kg−1 oil to zero. The same regulars were found in palm oil (POV from 21 to 79 meq O2 kg−1 versus α-tocopherol from 110 mg kg−1 oil to zero) and camellia oil (6 to 24 meq O2 kg−1 versus 120 mg kg−1 oil to zero). Total contents of tocopherols had the same variation as α-tocopherol because of its predominant content. POV, which increased significantly, also demonstrated that the oxidation reaction was transformed to intermediate or advanced oxidation stage, and a large number of hydroperoxides was generated because of many free radicals and intermediates.[Citation34] In these stages, POV of oxidized samples (perilla oil and rapeseed oil) increased slightly. At last, POV decreased because hydroperoxides were unstable and highly susceptible to further breakdown into secondary oxidation products, such as aldehydes.[Citation35] Thus, POV only was not enough to assess the quality of edible oils.
TBARS Value
During the 30-day oxidation test, the increases in TBARS values of five VLLO samples (corn oil, sunflower oil, peanut oil, camellia oil, and palm oil) were moderate. The final TBARS values of them were no more than 1.5 mg MDA kg−1 (), but those of B oil, soybean oil, and A oil increased by 10.27-, 3.86-, and 1.72-fold. This result was related to the fatty acid composition of oils. Autoxidized linolenate produced much more MDA than autoxidized linoleate, whereas autoxidized oleate essentially produced no MDA.[Citation36] Perilla oil was the HLO sample (57.33%) with the highest TBARS value. Hence, oils with a high n-3 PUFA level had a greater influence on TBARS value than samples with a low n-3 PUFA level.
FIGURE 4 Changes of secondary oxidation products (TBARS value) in oils during accelerated oxidation test (n = 3). Mean values with standard deviations are plotted as bars. The correlation coefficients (r2) between total tocopherol content and TBARS value were shown.
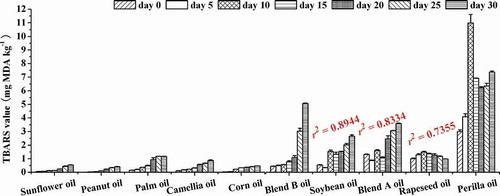
The change order of TBARS value was corresponded to that of POV in soybean, A and B oils. During oxidation, a series of secondary products were accompanied with the formation of peroxides and hydroperoxides. Besides, the increase of TBARS values in these oils was also positively correlated with their Cox value (r2 = 0.917). In addition, the TBARS value of soybean, blend A, and blend B oils also had negative linear correlation with the residual content of total tocopherol; the correlation coefficients were −0.8944, −0.8334, and −0.7355, respectively.
In addition, it also was observed that TBARS increased in fresh samples with the oxidation days increased. However, TBARS of the oxidized perilla and rapeseed oils tended to decrease, which may be due to the degradation of aldehydes. On the later oxidation stage, some aldehydes would break up to lower molecular weight compounds, which did not react with TBA, like organic acids and alcohols.[Citation37]
CONCLUSION
In conclusion, PUFA were the predominant factors in the oxidation of vegetable oils, and tocopherols were the predominant factors in the antioxidant of oils. The comprehensive behavior of these factors on stability of oils would be demonstrated well by the combination of POV and TBARS. When α-tocopherol was the main one of homologues, it presented a pro-oxidant effect at high concentration, but would become an antioxidant as its concentration decreased to the own inflection point for different kinds of vegetable oils. The inflection point concentration of α-tocopherol could correspond to the inflection point of POV increase. The other homologues of tocopherol like γ- or δ-tocopherol did not show pro-oxidant action as the main one of homologues. Therefore, the influence of α-tocopherol on oxidation was likely more predominant than fatty acids were.
FUNDING
This study was financed by the National Natural Science Fund (31071561), Jiangxi Provincial Natural Science Foundation of China (No. 20122BAB214001), the Chinese Post-Doctorate Science Foundation (No. 2012M511457), Jiangxi Provincial Post-Doctorate Support, and PhD Subject Fund from Education Department (20113601120004), and the Natural Science Fund from Science and Technology Department of Jiangxi Province (20114BAB214016).
REFERENCES
- Choe, E.; Min, D.B. Mechanisms and factors for edible oil oxidation. Comprehensive Reviews in Food Science and Food Safety 2006, 5, 169–186.
- Greenley, T.L.; Davies, M.J. Direct detection of radical generation in rat liver nuclei on treatment with tumour-promoting hydroperoxides and related compounds. Biochimica et Biophysica Acta (BBA)–Molecular Basis of Disease 1994, 1226, 56–64.
- Su, C.; White, P. Flavor stability and quality of high-oleate and regular soybean oil blends during frying. Journal of the American Oil Chemists’ Society 2004, 81, 853–859.
- Lee, J.H.; Pangloli, P. Volatile compounds and storage stability of potato chips fried in mid-oleic sunflower oil. International Journal of Food Properties 2013, 3, 563–573.
- Pillon, N.J.; Soulère, L.; Vella, R.E.; Croze, M.; Caré, B.R.; Soula, H.A.; Doutheau, A.; Lagarde, M.; Soulage, C.O. Quantitative structure–activity relationship for 4-hydroxy-2-alkenal induced cytotoxicity in L6 muscle cells. Chemico-Biological Interactions 2010, 188, 171–180.
- Bouaid, A.; Martinez, M.; Aracil, J. Long storage stability of biodiesel from vegetable and used frying oils. Fuel 2007, 86, 2596–2602.
- Neff, W.; Selke, E.; Mounts, T.; Rinsch, W.; Frankel, E.; Zeitoun, M. Effect of triacylglycerol composition and structures on oxidative stability of oils from selected soybean germplasm. Journal of the American Oil Chemists’ Society 1992, 69, 111–118.
- Kamal-Eldin, A. Effect of fatty acids and tocopherols on the oxidative stability of vegetable oils. European Journal of Lipid Science and Technology 2006, 108, 1051–1061.
- Bhatnagar, A.; Prasanth Kumar, P.; Hemavathy, J.; Gopala Krishna, A. Fatty acid composition, oxidative stability, and radical scavenging activity of vegetable oil blends with coconut oil. Journal of the American Oil Chemists’ Society 2009, 86, 991–999.
- Hulbert, A.J. Explaining longevity of different animals: Is membrane fatty acid composition the missing link? Age 2008, 30, 89–97.
- Jung, M.Y.; Min, D.B. Effects of α-, γ-, and δ-tocopherols on oxidative stability of soybean oil. Journal of Food Science 1990, 55, 1464–1465.
- Hassanien, M.F.R. Plant sterols and tocols profile of vegetable oils consumed in egypt. International Journal of Food Properties 2013, 3, 574–585.
- Kamal-Eldin, A.; Appelqvist, L.Å. The chemistry and antioxidant properties of tocopherols and tocotrienols. Lipids 1996, 31, 671–701.
- Yanishlieva, N.V.; Kamal-Eldin, A.; Marinova, E.M.; Toneva, A.G. Kinetics of antioxidant action of α- and γ-tocopherols in sunflower and soybean triacylglycerols. European Journal of Lipid Science and Technology 2002, 104, 262–270.
- Seppanen, C.M.; Song, Q.; Saari Csallany, A. The antioxidant functions of tocopherol and tocotrienol homologues in oils, fats, and food systems. Journal of the American Oil Chemists’ Society 2010, 87, 469–481.
- Simonne, A.; Eitenmiller, R. Retention of vitamin E and added retinyl palmitate in selected vegetable oils during deep-fat frying and in fried breaded products. Journal of Agricultural and Food Chemistry 1998, 46, 5273–5277.
- Rossi, M.; Alamprese, C.; Ratti, S. Tocopherols and tocotrienols as free radical-scavengers in refined vegetable oils and their stability during deep-fat frying. Food Chemistry 2007, 102, 812–817.
- Barrera-Arellano, D.; Ruiz-Méndez, V.; Márquez Ruiz, G.; Dobarganes, C. Loss of tocopherols and formation of degradation compounds in triacylglycerol model systems heated at high temperature. Journal of the Science of Food and Agriculture 1999, 79, 1923–1928.
- Cruz-Hernandez, C.; Deng, Z.Y.; Zhou, J.Q.; Hill, A.R.;Yurawecz, M.P.; Delmonte, P.; Mossoba, M.M.; Dugan, M.E.R.; Kramer, J.K.G. Methods for analysis of conjugated linoleic acids and trans-18:1 isomers in dairy fats by using a combination of gas chromatography, silver-ion thin-layer chromatography/gas chromatography, and silver-ion liquid chromatography. Journal of AOAC International 2004, 87, 545–562.
- Lei, L.; Li, J.; Li, G.Y.; Hu, J.N.; Tang, L.; Liu, R.; Fan, Y.W.; Deng, Z.Y. Stereospecific analysis of triacylglycerol and phospholipid fractions of five wild freshwater fish from Poyang Lake. Journal of Agricultural and Food Chemistry 2012, 60, 1857–1864.
- Fatemi, S.H.; Hammond, E.G. Analysis of oleate, linoleate and linolenate hydroperoxides in oxidized ester mixtures. Lipids 1980, 15, 379–385.
- Hu, J.N.; Zhang, B.; Zhu, X.M.; Li, J.; Fan, Y.W.; Liu, R.; Tang, L.; Lee, K.T.; Deng, Z.Y. Characterization of medium-chain triacylglycerol (MCT)-enriched seed oil from Cinnamomum camphora (Lauraceae) and its oxidative stability. Journal of Agricultural and Food Chemistry 2011, 59, 4771–4778.
- Hamam, F.; Shahidi, F. Enzymatic acidolysis of an arachidonic acid single-cell oil with capric acid. Journal of the American Oil Chemists’ Society 2004, 81, 887–892.
- Ahn, D.U.; Olson, D.; Jo, C.; Chen, X.; Wu, C.; Lee, J. Effect of muscle type, packaging, and irradiation on lipid oxidation, volatile production, and color in raw pork patties. Meat Science 1998, 49, 27–39.
- Guillén-Sans, R.; Guzmán-Chozas, M. Aldehydes in food and its relation with the TBA test for rancidity. Lipid/Fett 1995, 97, 285–286.
- Li, H.; Fan, Y.W.; Li, J.; Tang, L.; Hu, J.N.; Deng, Z.Y. Evaluating and predicting the oxidative stability of vegetable oils with different fatty acid compositions. Journal of Food Science 2013, 78, H633–H641.
- Pagani, M.A.; Baltanás, M.A. Production of natural antioxidants from vegetable oil deodorizer distillates: Effect of catalytic hydrogenation. Bioresource Technology 2010, 101, 1369–1376.
- Elisia, I.; Young, J.W.; Yuan, Y.V.; Kitts, D.D. Association between tocopherol isoform composition and lipid oxidation in selected multiple edible oils. Food Research International 2013, 52, 508–514.
- Isnardy, B.; Wagner, K.H.; Elmadfa, I. Effects of α-, γ-, and δ-tocopherols on the autoxidation of purified rapeseed oil triacylglycerols in a system containing low oxygen. Journal of Agricultural and Food Chemistry 2003, 51, 7775–7780.
- Frega, N.; Mozzon, M.; Lercker, G. Effects of free fatty acids on oxidative stability of vegetable oil. Journal of the American Oil Chemists’ Society 1999, 76, 325–329.
- Caglar, A.; Duman, E.; Ozcan, M.M. Effects on edibility of reused frying oils in the catering industry. International Journal of Food Properties 2012, 1–2, 69–80.
- Karabulut, I.; Durmaz, G.; Hayaloglu, A.A. C18 unsaturated fatty acid selectivity of lipases during the acidolysis reaction between tripalmitin and oleic, linoleic, and linolenic acids. Journal of the American Oil Chemists’ Society 2010, 87, 1301–1307.
- Verleyen, T.; Kamal-Eldin, A.; Mozuraityte, R.; Verhé, R.; Dewettinck, K.; Huyghebaert, A.; De Greyt, W. Oxidation at elevated temperatures: competition between α- tocopherol and unsaturated triacylglycerols. European Journal of Lipid Science and Technology 2002, 104, 228–233.
- Porter, N.A.; Caldwell, S.E.; Mills, K.A. Mechanisms of free radical oxidation of unsaturated lipids. Lipids 1995, 30, 277–290.
- Roman, O.; Heyd, B.; Broyart, B.; Castillo, R.; Maillard, M.N. Oxidative reactivity of unsaturated fatty acids from sunflower, high oleic sunflower and rapeseed oils subjected to heat treatment, under controlled conditions. LWT–Food Science and Technology 2013, 52, 49–59.
- Kenaston, C.B.; Wilbur, K.M.; Ottolenghi, A.; Bernheim, F. Comparison of methods for determining fatty acid oxidation produced by ultraviolet irradiation. Journal of the American Oil Chemists’ Society 1955, 32, 33–35.
- Guillen, M.D.; Goicoechea, E. Oxidation of corn oil at room temperature: Primary and secondary oxidation products and determination of their concentration in the oil liquid matrix from 1H nuclear magnetic resonance data. Food Chemistry 2009, 116, 183–192.