Abstract
Honey is a sweet substance that can be adulterated easily with inexpensive sweeteners by making syrup. Water being the second major constituent of honey, makes its flow behavior one of the important parameter for quality determination. In the present study, the rheological characteristics of honey samples adulterated with different concentrations of jaggery syrup were studied using rotational rheometer with parallel plate geometry. The viscosity of honey and adulterated honey samples was determined by varying temperature and percentage of jaggery concentration. All the adulterated honey samples behaved as non-Newtonian fluid. The viscosity increased linearly with increase in concentration of adulteration and varied from 2.48–4.80 Pa s as adulteration increased from 5 to 30%. Oscillatory tests were performed to find the possible effect of storage time on different honey samples. It was found that adulteration decreased the shelf life of honey, thus rheology can be considered one of the important parameters to determine adulteration of honey.
INTRODUCTION
Honey is the natural sweet substance produced by honey bees from nectar of plants or from secretions of living parts of plants or excretions of plant sucking insects on the living parts of plants.[Citation1] Honey has been used as medicinal remedy from ancient times and even now it has been reported to exhibit antibacterial and antiviral activity[Citation2−Citation4] and used for the cure of ulcers, bed sores, and other skin infections resulting from burns.[Citation5,Citation6] Honey is treated as an important dietary food product worldwide. It is a premium and expensive food product. In order to increase its quantity to make more profit, pure honey is adulterated with inexpensive adulterants such as sugar syrup, beet invert syrup, corn syrup, and jaggery syrup, etc. These adulterants increase the mass of honey but they decrease nutritional properties, thus degrading its quality.
Several studies have been done to detect adulteration in honey using different techniques. Spectroscopic methods such as Fourier transform infrared (FTIR) spectroscopy was studied for the detection of adulteration in honey using inverted cane sugar, corn syrup, and inverted beet syrup.[Citation7−Citation9] Addition of adulterants in honey such as jaggery was detected using near infrared spectroscopy and was able to predict the percentage adulteration in it.[Citation10]
Quality analysis of honey using isotopic ratio technique was done to detect adulteration of sucrose solution and high fructose corn syrup (HFCS) by determining 13C/12C ratio of carbohydrates and protein of the honey.[Citation11] Oligosaccharides profile was studied to detect adulteration of corn syrups and HFCS using high-performance anion-exchange chromatography (HPEAC).[Citation12]
Addition of these adulterants also affects the sugar and water content of honey. As honey is primarily composed of sugars such as fructose and glucose, the change in composition profile by adulteration effectuates its viscosity and thus, rheology. Many researchers have studied rheology of honey to determine the botanical origin, temperature effect, etc. Viscosity determination of sugar added honey samples with the help of a model was developed to predict the viscosity of honey based on sugar composition.[Citation13]
The rheological behaviour of pure honey mixed with propolis was studied and it was observed that viscosity of pure honey was reduced by the addition of propolis extract.[Citation14] Pseudo plastic behavior of Galician honey at 25°C was observed at low values of shear rate.[Citation15] Honey samples adulterated with starch, molasses, glucose, and distilled water has shown variation in their physico-chemical and rheological properties.[Citation16] Several studies have been done showing different honey samples following Newtonian law of Viscosity and Viscosity variation with temperature.[Citation17,Citation18]
A lot of work has been done on the viscosity-temperature relationship of honey and different models were developed to explain its characteristics.[Citation19−Citation21] Four models namely Williams-Landel-Ferry (WLF), Arrhenius, Vogel-Tammann-Fulcher (VTF), and power-law were investigated and it was observed that the WLF model was suitable for modeling the rheological behavior of different honey.[Citation22] An oscillatory test which is very much significant in rheology of honey has shown that viscous component predominates over elastic component.[Citation23] Also some of the modern oscillatory measurements showed that crystallization of honey is dependent on botanical origin, temperature, and storage time.[Citation24]
Among all the adulterants, jaggery is commonly used in North India mainly due to its physical appearance which is very much like multi-floral honey. The present study aims to study the rheological characteristics of honey samples adulterated with jaggery at different concentrations. Jaggery is commonly available in North India which is also known as Gur. It consists of 30–35 g/100 g of water, 40–60 g/100 g of sucrose, 15–25 g/100 g of invert sugars and trace elements.[Citation25] Samples under study were prepared by mixing honey with jaggery syrup. Brix value of syrup was kept same as honey sample.
EXPERIMENTAL METHOD AND ANALYSIS
Sample Preparation
Multifloral raw honey was collected from Punjab region (India) and was stored at ambient temperature for a month before it was used for the study. Jaggery used for the experiments had pH 5.11, Brix 77.73 and a refractive index 1.48203. The jaggery syrup for adulteration was prepared by mixing jaggery and distilled water in the ratio of 80:20 at 50°C. The mixture was stirred and sonicated continuously for 5 h. A total of six samples were prepared by adding the jaggery syrup to the honey in different concentration (w/w) varying from 5 to 30% with an increment of 5%. Then the samples were placed in water bath at 50°C for 2 h and sonicated for 5 h to produce a homogeneous solution. The prepared samples were brought at room temperature before analysis.
Rheological Measurement
Viscosity of honey samples was measured using Bohlin rheometer CVO 100 (Malvern Instruments Ltd.). Parallel plate geometry was used with a plate having radius of 20 mm for all the measurements. The gap between the plates was set to 1000 μm. All the rheological measurements were performed at 25°C. For each test, approximately 2–3 g of the sample was placed on the bottom plate. Two types of tests were performed namely viscometry and oscillation. The flow behavior of honey was studied by measuring the viscosity at 25°C and with temperature gradient of 5 to 30°C at a constant stress of 10 Pa. The viscosity of the honey samples along with the adulterated samples was determined as a function of temperature using Arrhenius equation.
RESULTS AND DISCUSSION
The variation in moisture content with respect to concentration of adulteration is shown in . As concentration of jaggery syrup increases there is a linear reduction in moisture content of the honey samples. The moisture content ranges from 19.7 to 21.6 g/100 g. The addition of jaggery syrup to honey increases its solid content because of the presence of sucrose and invert sugars that results in a decrease in the moisture content. illustrates the viscosity of different honey samples adulterated with jaggery syrup at a constant stress of 10 Pa at 298 K for initial 300 s. The viscosity was measured as a function of time. It was observed that there was a slight increase in viscosity as time increases showing time dependency. The rheopectic behavior of honey and jaggery adulterated honey samples were observed from the above graph indicating non-Newtonian behavior. It was found that viscosity increases with increase in percentage adulteration and the increase shows a linear behavior. As viscosity is related to the flow behaviour hence decrease in the moisture content due to adulteration results in the increase in viscosity as shown in .
FIGURE 2 (a) Variation of viscosity with respect to time for honey and adulterated samples at constant shear stress of 10 Pa; (b) Viscosity of samples having different concentration of adulteration.
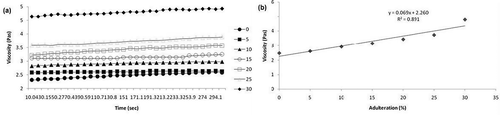
shows steady shear rheograms of honey samples adulterated with different concentrations of jaggery syrup at 298 K. The shear rate was varied from 0–20 per second and corresponding stress values were obtained. It is seen from the graph that pure honey shows Newtonian behavior as listed in literature for Chinese honey, Greek Honey, light and dark colored honey, and Indian honey.[Citation27−Citation30] It is clear from the figure that all the adulterated samples behave as Bingham plastics and its flow behavior can be explained by Bingham model:
shows that apparent viscosity decreases with temperature and the temperature dependence of viscosity follows the Arrhenius model. Viscosity of all the samples were measured by varying the temperature of the samples from 273–303 K. As the temperature of honey is raised, there is a gradual decrease in viscosity.[Citation31–Citation33] Beyond 295 K, the change in viscosity is very slow. As the temperature rises, viscosity decreases because particles in honey gain enough energy to overcome the intermolecular forces of attraction, thus decreasing the cohesive forces. Decrease in these cohesive forces reduces the viscosity in the honey. All the samples can be easily differentiated at lower temperatures from 273–295 K and showed similar behavior at higher temperature. It was also observed that viscosity increases with increase in adulterant concentration at a particular temperature, but this variation is not seen at high temperature.
FIGURE 5 (a) Viscosity versus temperature relationship in the range 273–303 K (0–30°C); (b) Arrhenius plot for various adulterated samples.
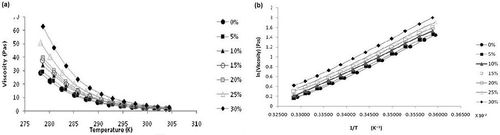
The activation energy of honey samples was calculated from Eq. (1) by plotting the graph between ln η and 1/T as shown in . The calculated values of activation energy at different concentration of adulteration are given in . There is an increase in activation energy with increase in adulteration, because more energy is required by the particles of the honey samples to overcome the viscous forces. This confirms that increase in the concentration of jaggery syrup increases the viscosity.
TABLE 1 The activation energies of the jaggery adulterated honey samples
Oscillatory tests were done to determine the possible effect of storage time on raw honey and adulterated honey with 30% jaggery syrup at 298 K (). It is observed that the viscous modulus (G”) dominates elastic modulus (G’) in both the samples until a particular frequency is reached and after that G’ increases as reported in literature.[Citation34−Citation37] The elastic modulus (G’) of both samples remains the same. This shows that adulteration only affects the viscous properties of the honey. The amplitude of viscous modulus of 30% adulterated sample is more as compared to that of raw honey. So, more adulteration means a more viscous behavior of the honey sample which is also depicted by the viscometry tests. It is shown in that the phase angle linearly decreases with the increase in the frequency of operation. The phase angle of the system is above 45°, which indicates the elastic nature of the sample under test. This phenomenon is more evident for the samples having adulteration above 20%.
FIGURE 6 Elastic modulus (G’) and viscous modulus (G’’) versus frequency of raw honey and 30% jaggery adulterated sample.
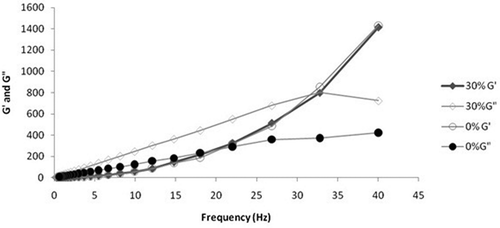
Variation of crossover frequency with different percentage of adulterated samples is shown in . It is clear from the graph that less time (higher frequency) is required by the sample to solidify as the concentration of adulteration increases and if time is delayed, the elastic behavior will dominate, leading to the complete crystallization of honey at lower frequency. This is also indicated in . So adulteration causes the degradation of honey and crossover frequency can be considered as one of the parameter to know the percentage of adulteration in honey.
CONCLUSION
Rheology plays an important role to study the effect of jaggery adulteration in honey. The study indicates that adulteration in honey affects both its viscometric and dynamic rheological behavior. Moisture content decreases with the rise in adulteration concentration. All the samples showed non-Newtonian behavior except pure honey. It was found that raw honey had minimum viscosity of 2.48 Pa s among the six samples whereas 4.83 Pa s was the maximum viscosity obtained by the 30% adulterated sample. Arrhenius model was able to justify the relationship between viscosity and temperature. There was an exponential decay in the viscosity with respect to temperature. The highest activation energy was achieved by the 30% adulterated sample which is 38.48 KJ/mol. The effect of storage time on the adulteration was depicted by oscillatory tests and it was found that lesser time will be taken by the highly adulterated sample to solidify and adulteration can be predicted at lower temperatures.
ACKNOWLEDGMENTS
The authors are thankful to Sr. TO (2) Mrs. Virmita Malhotra, C.S.I.O. Chandigarh for her help and discussions during the work. The research work was carried out at Central Scientific Instruments Organization (CSIR), Chandigarh (Punjab).
REFERENCES
- Codex Alimentarius. Draft revised for honey at step 6 of the Codex Procedure. CX5/10.2, CL1998/12-S 1998.
- Khalil, A.T.; Khan, I.; Ahmad, K.; Khan, Y.A.; Khan, J.; Shinwari, Z.K. Antibacterial activity of honey in north-west Pakistan against select human pathogens. Journal of Traditional Chinese Medicine 2014, 34, 86–89.
- Badet, C.; Quero, F. The in vitro effect of manuka honeys on growth and adherence of oral bacteria. Anaerobe 2011, 17, 19–22.
- Watanabe, K.; Rahmasari, R.; Matsunaga, A.; Haruyama, T.; Kobayashi, N. Anti-influenza viral effects of honey in vitro: Potent high activity of manuka honey. Archives of Medical Research 2014, 45, 359–365.
- Mohamed, H.; El Lenjawi, B.; Abu Salma, M.; Abdi, S. Honey based therapy for the management of a recalcitrant diabetic foot ulcer. Journal of Tissue Viability 2014, 23, 29–33.
- Vandamme, L.; Heyneman, A.; Hoeksema, H.; Verbelen, J.; Monstrey, S. Honey in modern wound care: A systematic review. Burns 2013, 39, 1514–1525.
- Sivakesava, S.; Irudayaraj, J. Prediction of inverted cane sugar adulteration of honey by Fourier transform infrared spectroscopy. Journal of Food Science 2001, 66, 972–978.
- Sivakesava, S.; Irudayaraj, J. A rapid spectroscopic technique for determining honey adulteration with corn syrup. Journal of Food Science 2001, 66, 787–791.
- Sivakesava, S.; Irudayaraj, J. Detection of inverted beet sugar adulteration of honey by FTIR spectroscopy. Journal of the Science of Food and Agriculture 2001, 81, 683–690.
- Mishra, S.; Kamboj, U.; Kaur, H.; Kapur, P. Detection of jaggery syrup in honey using near-infrared spectroscopy. International Journal of Food Science and Nutrition 2010, 61, 306–315.
- Padovan, G.J.; Jong, D.D.; Rodrigues, L.P.; Marchini, J.S. Detection of adulteration of commercial honey samples by the13C/12C isotopic ratio. Food Chemistry 2003, 82, 633–636.
- Morales, V.; Corzo, N.; Sanz, M.L. HPAEC-PAD oligosaccharide analysis to detect adulterations of honey with sugar syrups. Food Chemistry 2008, 107, 922–928.
- Mossel, B.; Bhandari, B.; D’Arcya, B.; Caffin, N. Determination of viscosity of some Australian honeys based on composition. International Journal of Food Properties 2003, 6, 87–97.
- Da Costa, C.C.; Pereira, R.G. The influence of propolis on the rheological behaviour of pure honey. Food Chemistry 2002, 76, 417–421.
- Gómez-Díaz, D.; Navaza, J.M.; Quintáns-Riveiro, L.C. Rheological behaviour of Galician honeys. European Food Research and Technology 2006, 222, 439–442.
- El-Bialee, N.M.; Sorour, M.A. Effect of adulteration on honey properties. International Journal of Applied Science and Technology 2011, 1, 122–133.
- Zaitoun, S.; Ghzawi, A.A.; Al-Malah, K.I.M.; Abu-Jdayil, B. Rheological properties of selected light colored Jordanian honey. International Journal of Food Properties 2001, 4, 139–148.
- Yanniotis, S.; Skaltsi, S.; Karaburnioti, S. Effect of moisture content on the viscosity of honey at different temperatures. Journal of Food Engineering 2006, 72, 372–377.
- Recondoa, M.P.; Elizalde, B.E.; Buera, M.P. Modeling temperature dependence of honey viscosity and of related supersaturated model carbohydrate systems. Journal of Food Engineering 2006, 77, 126–134.
- Juszczak, L.; Fortuna, T. Rheology of selected Polish honeys. Journal of Food Engineering 2006, 75, 43–49.
- Al-Malah, K.I.M.; Abu-Jdayil, B.; Zaitoun, S.; Ghzawi, A.A. Application of WLF and Arrhenius kinetics to rheology of selected dark-colored honey. Journal of Food Process Engineering 2001, 24, 341–357.
- Sopade, P.A.; Halley, P.; Bhandari, B.; D’Arcy, B.; Doebler, C.; Caffin, N. Application of the Williams-Landel-Ferry model to the viscosity-temperature relationship of Australian honeys. Journal of Food Engineering 2002, 56, 67–75.
- Ahmed, J.; Prabhu, S.T.; Raghavan, G.S.V.; Ngadi, M. Physico-chemical, rheological, calorimetric, and dielectric behavior of selected Indian honey. Journal of Food Engineering 2007, 79, 1207–1213.
- Smanalieva, J.; Senge, B. Analytical and rheological investigations into selected unifloral German honey. European Food Research and Technology 2009, 229, 107–113.
- Rao, P.V.K.J.; Das, M.; Das, S.K. Jaggery—A traditional Indian sweetener. Indian Journal of Traditional Knowledge 2007, 6, 95–102.
- F.A.O. Manuals of Food Quality Control (14/8). Press: Rome, 1997; 119 pp.
- Junzheng, P.; Changying, J. General rheological model for natural honeys in China. Journal of Food Engineering 1998, 36, 165–168.
- Lazaridou, A.; Biliaderis, C.G.; Bacandritsos, N.; Sabatini, A.N. Composition thermal and rheological behaviour of selected Greek honeys. Journal of Food Engineering 2004, 64, 9–21.
- Kumar, J.S.; Mandal, M. Rheology and thermal properties of marketed Indian honey. Nutrition and Food Science 2009, 39, 111–117.
- Abu-Jdayil, B.; Ghzawi, A.A.; Malah, K.I.M.; Zaitoun, S. Heat effect on rheology of light- and dark-colored honey. Journal of Food Engineering 2002, 51, 33–38.
- Mossel, B.; Bhandari, B.; D’Arcy, B.; Caffin, N. Use of an Arrhenius model to predict rheological behaviour in some Australian honeys. LWT-Food Science and Technology 2000, 33, 545–552.
- Kayacier, A.; Karaman, S. Rheological and some physicochemical characteristics of selected Turkish honeys. Journal of Texture Studies 2008, 39, 17–27.
- Gómez-Díaz, D.; Navaza, J.M.; Quintáns-Riveiro, L.C. Effect of temperature on the viscosity of honey. International Journal of Food Properties 2009, 12, 396–404.
- Yoo, B. Measurement of dynamic rheology during cooling of honey-invert sugar mixtures by small-deformation oscillatory rheometry. Food Science and Biotechnology 2012, 21, 1217–1220.
- Yoo, B. Effect of temperature on dynamic rheology of Korean honeys. Journal of Food Engineering 2004, 65, 459–463.
- Oroian, M.; Amariei, S.; Escriche, I.; Leahu, A.; Damian, C.; Gutt, G. Chemical Composition and temperature influence on the rheological behaviour of honeys. International Journal of Food Properties 2014, 17, 2228–2240.
- Kayacier,A.; Yüksel, F.; Karaman, S. Dynamic mechanical spectra of selected Turkish honeys: Construction of predictive models for complex viscosity using two different nonlinear modeling techniques. International Journal of Food Properties 2014, 17, 93–110.