Abstract
The objectives of this work were to study the ultrasound- and ultraviolet light-induced inactivation kinetics of polyphenol oxidase extracted from different sources in a model system. The polyphenol oxidase crude extract was obtained from bananas, apples, quince, eggplants, plums, dill, and cultured mushrooms, which exhibited high enzyme activity. The polyphenol oxidase crude extract was treated with ultrasound and ultraviolet light at 40°C temperature for 40 min. The study showed that the polyphenol oxidase enzyme was inactivated between 12 and 100% during ultrasound only treatment; between 4 and 29% during ultraviolet light only treatment; and between 80 and 100% during simultaneous ultrasound and ultraviolet light treatment. Based on the measurements, an exponential decay model for determining polyphenol oxidase inactivation kinetics was developed. The model provides high determination coefficients (R2): 0.968–0.999 with ultrasound only treatment, 0.881–0.990 with ultraviolet only treatment, and 0.975–1.000 with simultaneous ultrasound and ultraviolet treatment. The polyphenol oxidase kinetics evaluation showed that different treatments provided different inactivation times, or D-values. The D-values were 7.0–656.1 min for ultrasound only treatment (DUS), 251–1887 min for ultraviolet only treatment (DUV), and 3.3–59.4 min for combined ultrasound and ultraviolet treatment (DUS+UV).
INTRODUCTION
Polyphenol oxidase (PPO; EC. 1.14.18.1) is an enzyme responsible for the browning that occurs in many vegetables and fruits, as well as melanization in animals. The enzyme catalyzes the hydroxylation of monophenols to o-diphenols and the oxidation of o-diphenols to o-quinones in the presence of oxygen. These o-quinones are readily polymerized and cause undesirable brown pigmentation, which leads to organoleptic and nutritional loss and causes serious problems.[Citation1–Citation3] Since enzymatic browning has generally negative influences on food quality, it is considered undesirable in food technology. Therefore, the inactivation kinetics of PPO has been investigated by numerous researchers.[Citation4–Citation8]
Enzymatic browning can be reduced by controlling the enzyme, substrate, or oxygen (O2) content of a food product. Thermal and non-thermal methods, such as chemical treatments, have been widely used for controlling PPO in foods. However, heat treatment can result in undesirable organoleptic and nutritional changes such as changes in taste, flavor, color, and nutritional value. Therefore, recent studies have focused on the non-thermal treatment techniques, as consumers demand fresh-like food products.[Citation9,Citation10]
Ultrasound (US), high hydrostatic pressure (HPP), pulsed electric field (PEF), ultraviolet (UV), and carbon dioxide (CO2) treatments are widely used and researched non-thermal techniques used for enzyme inactivation, either alone or in combination. US technology has been used to inactivate enzymes such as lipoxygenase,[Citation11] lipase,[Citation12] protease,[Citation12] pectin esterase,[Citation13–Citation15] lactoperoxidase,[Citation16] alkaline phosphatase,[Citation16] and PPO.[Citation7] Kuldiloke reported that US treatment performed at temperatures above 60°C could inactivate PPO effectively.[Citation17] UV light is not used widely for enzyme inactivation due to its limited penetration depth. The penetration depth of ultraviolet-C light (200–280 nm) has been reported to be 4 m in air, 30 cm in clear water, only 1 mm in fruit juices, and no more than 0.1 mm in milk.[Citation18,Citation19] However, Guerrero-Beltran and Barbosa-Canovas reported that UV light could inactivate PPO in mango nectar up to 81%,[Citation19] and Manzocco et al. reported that UV-C light treatments could result in complete PPO inactivation in their model system.[Citation20]
A first-order model is generally used for describing the kinetics of enzyme inactivation by thermal or non-thermal processing. Although the kinetic models of PPO inactivation by several non-thermal processing techniques have been established,[Citation4–Citation6] a model for PPO inactivation using combined UV and US treatments is not available. Therefore, this research focused first on developing a first-order model of a combined treatment and on obtaining D-values for the model.
The combination of US and UV was described by Toy and Carter as photosonication.[Citation21] This treatment was first studied in food research by Şengül et al., who reported that the combination could be used successfully for microbial inactivation in milk.[Citation22] Başlar and Ertugay reported that the combination method is more effective than stand-alone US treatment for PPO inactivation in cloudy apple juice.[Citation7] The aim of the present study was to determine the effects of US- and UV light on the inactivation kinetics of PPO extracted from selected vegetables, fruits, and mushrooms in aqueous model systems.
MATERIALS AND METHODS
Material
In this study, crude extracts of banana (Musa cavendishii), apple (Malus domestica, cv. Amasya), quince (Cydonia vulgaris), eggplant (Solanum melongena), plum (Prunus domestica), dill (Anethum graveolens), and culture mushroom (whitecap) procured from the city of Erzurum, Turkey were used. Final concentrations of polyvinylpyrrolidone (PVP) and Triton X-100 in buffer solutions were adjusted to 1 and 0.25%, as suggested by Galeazzi et al.[Citation23] To prepare the extracts, 0.2 M phosphate buffer (pH 7) for banana, plum, and eggplant; 0.2 M phosphate buffer (pH 6.5) for dill and culture mushroom; and 0.5 M phosphate buffer (pH 7.3) for apple and quince were used. The material and buffer (the ratio of material to solution was 1/2 kg/L) were homogenized with a mixer for 30–60 s and then filtered. The extract was then centrifuged at 18,000 × g at 4°C for 30 min.
PPO Activity
PPO enzyme activity was recorded as described by Şakiroğlu.[Citation24] One unit of PPO enzyme activity (EU) was defined as the amount of enzyme that caused an increase in 0.001 ΔA420 per minute for 1 mL under the assay conditions. The increase in absorbance was recorded at 420 nm with a spectrophotometer (Pharmacia LKB Biochrom Ultrospec III, UK) for 3 min after 2.8 mL phosphate buffer was mixed with 0.1 mL catechol (Sigma C9510) solution (0.1 M) and 0.1 mL crude extract. The catechol solution was used as the enzymatic substrate for PPO.
US and UV Treatments
The US treatment was carried out with a US processor (Hielscher UP400S, Germany) working at a constant frequency (24 kHz) with a 22 mm-diameter titanium probe (H22 L2D). The maximum acoustic power density of the processor was 85 W/cm2, providing a maximum amplitude of 100 µm and efficiency of 90% or above.[Citation25] The US probe was immersed into the 200 mL PPO crude extract at a depth of approximately 2.5 cm in a 250 mL glass reactor with cooling jacket. US was applied using maximum amplitude level (100%).
The UV treatment was carried out with four 4400 µW/cm UV lamps (Pen-Ray UV lamps, 254 nm; Cole-Parmer, USA). The UV lamps were immersed in the PPO extract in parallel at a depth of approximately 3.5 cm. The combined US and UV treatments were carried out by using the US processor and UV lamps simultaneously, similar to the methods described by Şengül et al.[Citation22] and Karaman et al.[Citation26] A peristaltic pump working at 7 L/min and a water bath (Memmert, Germany) were used to maintain the temperature of the samples in the glass reactor with cooling jacket at 40°C to avoid overheating during the treatments. The temperatures of the PPO extracts were checked with a thermometer. The treatments were applied to the extracts for up to 40 min, with maximum 5-min periods.
Mathematical Modeling
The PPO inactivation kinetics was modeled by fitting an exponential decay model, a first-order kinetic model (Eq. 1), to the experimental data:
where, A is the PPO activity (EU) of the extracts, A0 is the PPO activity (EU) before treatment, t is time (min), and –k is the first-order kinetic constant (min–1). Decimal reduction time (D) in minutes were expressed using Eq. (2):
The derived models were evaluated using root mean square error (RMSE), Chi-square (χ2), and determination coefficient (R2) to detect differences between the experimental data and the model estimates.[Citation27]
RESULTS AND DISCUSSION
Effect of US and UV Treatment on PPO Stability
The PPO activities of banana, apple, quince, eggplant, plum, dill, and culture mushroom extract in phosphate buffer were determined to be 725, 352, 394, 542, 608, 301, and 725 EU/mL, respectively. The effects of the stand-alone and combined US and UV treatments on relative inactivation of the PPO of eggplant, dill, plum, and culture mushroom are shown logarithmically in , , , and , respectively. The relative enzyme activity decreased dramatically with the 40 min treatment time in the stand-alone US and combined treatments, and decreased slowly with treatment time in the stand-alone UV treatment. The effects of the stand-alone US treatment and the combined US and UV treatments on the relative PPO inactivation of quince, apple, and banana are shown logarithmically in , , and , respectively. Stand-alone UV treatment was not performed on PPO extracted from quince, apple, or banana in this study.
FIGURE 1 Logarithmic inactivation of PPO extracted from (a) eggplant, (b) dill, (c) plum, and (d) mushroom.
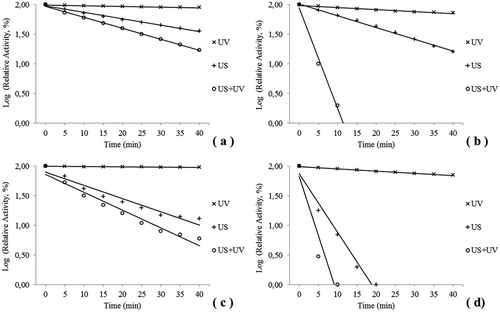
In general, the residual PPO activity decreased logarithmically during all of the treatments. After 40 min of the stand-alone UV treatment, plum and eggplant PPO were only inactivated 4 and 10%, respectively, the dill and culture mushroom enzymes were inactivated 27 and 29%, respectively. It was observed that the large differences in inactivation rates were due to the different extracted sources. The UV radiation penetration problem might be mitigated to some extent with US agitation.
PPO inactivation by UV light was not generally enough for food technology, as UV light treatment has some disadvantages due to its penetration problem. In this study, an aqueous model system was used to prevent the negative effects of factors such as organic material. Manzocco et al.[Citation20] reported that PPO in their model system was inactivated by 13.8 W/m UV light after about 75 min, and that residual PPO activity was over 40% after 3.9 W/m UV light was applied for 150 min.[Citation20] They also reported that when more UV lamps are used, greater enzymatic inactivation is achieved. Guerrero-Beltran and Barbosa-Canovas reported that PPO enzyme in mango nectar was inactivated up to 81% by UV light.[Citation19] In the current study, the maximum level of PPO inactivation by UV light was 29%, in cultured mushrooms after 40 min.
It has also been reported that enzyme modification associated with protein photo-oxidation might be direct photo-oxidation arising from the absorption of radiation by the protein structure or bound chromophore and indirect protein oxidation mediated by singlet oxygen generated by protein-bound or other chromophores.[Citation20,Citation28] Modifications to the native structure of enzymes lead to activity loss. UV radiation can cause oxidation of sulfhydryl groups in enzyme PPO and changes in the native structure of the latter, as well as the formation of covalent crosslinks between polypeptides. However, the mechanism of enzyme inactivation by UV light is not clearly understood.[Citation29]
In the present study, stand-alone US treatment and combined US and UV treatment inactivated PPO enzymes at different rates. Activity loss of the dill and mushroom PPOs were observed during the US only and combined treatments; they exhibited rapid inactivation during the first minutes ( and ). Inactivation occurred at 25 min with the stand-alone US treatment and after 15 min of the combined treatment. Banana PPO activity decreased only 12% under stand-alone US after 40 min; however, its reduction rate with the combined treatment was 80%. A reduction of 88% in apple enzyme activity was achieved by stand-alone US after 40 min, and it was completely inactivated by the combined treatment after 35 min.
Cheng et al.[Citation30] reported that 46% of PPO activity in mushroom extract was lost after 30 min at 55°C, and that the reduction was more than 90% at 60°C.[Citation30] Başlar and Ertugay reported that apple juice PPO was inactivated about 83% by US after 10 min at 60°C, while the reduction was only about 29% at 50°C.[Citation7] These studies showed that PPO activity decreased very rapidly at temperatures above 50°C.
Acoustic shock waves produced by US treatment can result in strong shear and cause protein denaturation to occur via the breakdown of hydrogen bonding and van der Waals interactions in the polypeptide chains. The effective mechanism of PPO inactivation by US treatment is mainly attributed to acoustic cavitation caused by the extreme increase in localized pressure (1000 MPa) and temperature (5000 K). When US energy is applied to a liquid medium, the formation of highly reactive chemical radicals may also take place alongside the previously mentioned extreme pressure and temperature.[Citation31] These radicals are also important for enzyme inactivation. The influence of the combination of US and UV treatment on different PPO sources is greater than that of stand-alone US and UV treatment. It was predicted that the inactivation mechanism of PPO via the combination method is mainly due to acoustic cavitation and secondarily due to photo-oxidation caused by UV light. Agitation is also a factor in the achievement of better enzyme inactivation results with the combined use of US and UV.
The methods used in this study affected PPO inactivation at different levels in the various enzyme sources. This effect might be due to the different isoenzymes of the PPOs extracted from the various sources. It is possible that some isoenzymes were sensitive to US and/or UV, while others were stable. In addition, the presence of compounds absorbed in the UV might act as a shelter for the enzyme. Liu et al. reported a similar inactivation effect obtained for PPO of watermelon juice by high-pressure CO2.[Citation32]
The Inactivation Kinetics of PPO during Treatment
US, UV, and combined treatment were modeled for the inactivation of PPO in phosphate buffer. Estimations of the kinetic parameters (k, D-value, and R2) of the first-order reaction to inactivate the kinetics of PPO extracted from the different sources, which were computed using Eqs. (1) and (2), are presented in . The experimental data were well fitted to first-order kinetic models, with high determination coefficients (R2): 0.968–0.999 for US-only treatment; 0.881–0.990 for UV-only treatment; and 0.975–1.000 for the US and UV combination treatment. The RMSE and χ2 values were mostly low, except for some treatments used for dill and apple. A high R2 value or low RMSE and χ2 values means that inactivation can be predicted more accurately. As a result, the statistical analysis test parameters indicated that the combination treatment model displayed better effects than the stand-alone US and UV treatments. The results also showed that the highest R2 values (1.000) and lowest absolute RMSE and χ2 values (0.304 and 0.104, respectively) were obtained with the statistically fitted model of inactivation by the US and UV combination method of PPO extracted from mushrooms.
TABLE 1 Inactivation kinetics parameters of the first-order model* for PPO extracted from different sources
shows that the k values were 0.351, 2.774, 4.351, 4,777, 5.043, 7.094, and 32.775 min–1 in banana, eggplant, dill, quince, apple, plum, and mushroom, respectively, with the US treatment. Higher k values indicate more sensitive PPO stability. The results also indicate that banana PPO is more stable than that of the other sources. With the UV and US combination treatment, the k values were 3.877, 4.680, 6.780, 7.323, 10.425, 45.670, and 49.767 in banana, eggplant, quince, apple, plum, dill, and mushroom, respectively. The combination treatment increased inactivation of PPO as the US-only treatment did. However, the most striking point here is that the combined treatment increased the inactivation of PPO extracted from dill significantly. With the UV treatment, the k values were 0.122, 0.310, 0.911, and 0.917 in plum, eggplant, dill, and mushroom. It was observed that the effect of UV light on PPO was very weak compared with US. In addition, it was observed that with UV light, the PPO extracted from dill was affected more than the PPO extracted from plum, while the opposite was true with the US treatment ( and ). This finding indicates that the effects of UV and US on the PPO enzymes differed depending on the various PPO sources.
Enzyme activity tends to decrease logarithmically with time under inactivation conditions. The decimal reduction time, or D-value, which is the time required at a certain temperature to inactive 1 log (90%) of the enzyme being studied, is calculated as a function of the first-order kinetic constant. In the present study, the DUV values were determined to be between 251 and 1887 min. Guerrero-Beltran and Barbosa-Canovas[Citation19] reported that their DUV values computed from the first-order kinetics ranged from 152 to 199 min (2.280 to 2.991 kJ/m2). The DUS values in the current study were between 7.0 and 656.1 min, and the DUS+UV values were 3.3 and 59.4 min for the combined US and UV treatment. The kinetic parameters showed that the most vulnerable PPOs were found in eggplant and most stable PPOs were found in bananas. A long time is needed to inactive 1 log of PPO enzyme by UV; the combination treatment reduced the amount of the time. Başlar and Ertugay[Citation7] reported D40, D50, and D60 values of PPO were 146, 66.4, and 12.9 min, respectively, with US at 100 μm amplitude, while the D-values with photosonication were 116, 35.2, and 10.3 min, respectively.
CONCLUSIONS
The combined US and UV treatments were found to be more effective for inactivating the PPO in extracts than the stand-alone treatments. Synergistic inactivation of PPO was observed during the combined treatment compared to the stand-alone treatments. The inactivation of PPOs extracted from different sources exhibited first-order inactivation kinetics during all treatments. This study provides a scientific and technological basis for using UV, US, and combination of the two in food processing.
Acknowledgments
The authors would like to thank Dr. Mustafa Fatih Ertugay from the Department of Food Engineering, Atatürk University for his valuable support during this study.
References
- Mazzafera, P.; Robinson S.P. Characterization of polyphenol oxidase in coffee. Phytochemistry 2000, 55, 285–296.
- Yang, C.P.; Fujita, S.; Ashrafuzzaman, M.D.; Nakamura, N.; Hayashi, N. Purification and characterization of polyphenol oxidase from banana (Musa sapientum L.) pulp. Journal of Agriculture and Food Chemistry 2000, 48, 2732–2735.
- Kumar, V.B.A.; Mohan, T.C.K.; Murugan K. Purification and kinetic characterization of polyphenol oxidase from Barbados cherry (Malpighia glabra L.). Food Chemistry 2008, 110, 328–333.
- Zhong, K.; Wu, J.; Wang, Z.; Chen, F.; Liao, X.; Hu, X.; Zhang Z. Inactivation kinetics and secondary structural change of PEF-treated POD and PPO. Food Chemistry 2007, 100, 115–123.
- Altunkaya, A. Partial purification and characterization of polyphenoloxidase from Turkish tea leaf (Camellia Sinensis L.). International Journal of Food Properties 2014, 17, 1490–1497.
- Aydemir, T. Selected kinetic properties of polyphenol oxidase extracted from Rosmarinus Officinalis L. International Journal of Food Properties 2010, 13, 475–485.
- Başlar, M.; Ertugay, M.F. The effect of ultrasound and photosonication treatment on polyphenoloxidase (PPO) activity, total phenolic component, and colour of apple juice. International Journal of Food Science and Technology 2013, 48, 886–892.
- Sakiroglu, H.; Yilmaz, E.; Erat, M.; Ozturk, A.E. Selected properties of polyphenol oxidase obtained from Ispir sugar bean. International Journal of Food Properties 2013, 16, 1314–1321.
- Barbosa-Canovas, G.V.; Gongora-Nieto, M.M.; Pothakamury, U.R.; Swanson, B.G. Fundamentals of High-Intensity Pulsed Electric Fields (PEF). In Preservation of Foods with Pulsed Electric Fields; Academic Press: USA, 1999; 1–19.
- Falguera, V.; Pagán, J.; Ibarz, A. A kinetic model describing melanin formation by means of mushroom tyrosinase. Food Research International 2010, 43, 66–69.
- Lopez, P.; Burgos, J. Lipoxygenase inactivation by manothermosonication: Effects of sonication physical parameters, pH, KCl, sugars, glycerol, and enzyme concentration. Journal of Agricultural and Food Chemistry 1995, 43, 620–625.
- Vercet, A.; Lopez, P.; Burgos, J. Inactivation of heat-resistant lipase and protease from Pseudomonas fluorescens by manothermosonication. Journal of Dairy Science 1997, 80, 29–36.
- Lopez, P.; Vercet, A.; Sanchez, A.C.; Burgos, J. Inactivation of tomato pectic enzymes by manothermosonication. Zeitschrift für Lebensmittel-Untersuchung und. Forschung 1998, 207, 249–252.
- Vercet, A.; Lopez, P.; Burgos, J. Inactivation of heat-resistant pectinmethylesterase from orange by manothermosonication. Journal of Agricultural and Food Chemistry 1999, 47, 432–437.
- Vercet, A.; Sanchez, C.; Burgos, J.; Montanes, L.; Lopez-Buesa, P. The effects of manothermosonication on tomato pectic enzymes and tomato paste rheological properties. Journal of Food Engineering 2002, 53, 273–278.
- Ertugay, M.F.; Yüksel, Y.; Sengül, M. The effect of ultrasound on lactoperoxidase and alkaline phosphatase enzymes from milk. Milchwissenschaft 2003, 58, 593–595.
- Kuldiloke, J. Effect of ultrasound, temperature, and pressure treatments on enzyme activity and quality indicators of fruit and vegetable juices. Doctorate thesis, Technischen Universität Berlin, Berlin, Germany, 2002.
- Mannapperuma, J.D. Residual Management in Fruit Processing Plants. Processing Fruits: Science and Technology, Vol. 1. In Biology, Principles, and Applications; Somogyi, L.P.; Ramaswamy, H.S.; Hui, Y.H.; Eds.; Lancaster, PA, 1996.
- Guerrero-Beltran, J.A.; Barbosa-Canovas, G.V. Inactivation of Saccharomyces cerevisiae and polyphenolokxidase in mango nectar treated with UV light. Journal of Food Protection 2006, 69, 362–368.
- Manzocco, L.; Quarta, B.; Dri, A. Polyphenoloxidase inactivation by light exposure in model systems and apple derivatives. Innovative Food Science and Emerging Technologies 2009, 10, 506–511.
- Toy, M.S.; Carter, M.K. Method for detecting organic compounds by photoacoustic conversion. US patent. Patent No: 4945063, 1990.
- Şengül, M.; Erkaya, T.; Başlar, M.; Ertugay, M.F. Effect of photosonication on inactivation of total and coliform bacteria in milk. Food Control 2011, 22, 1803–1806.
- Galeazzi, M.A.M.; Sgarbieri, V.C.; Constantinides, S.M. Isolation, purification, and physicochemical characterization of polyphenoloxidases (ppo) from a dwarf variety of banana (Musa cavendishii, L). Journal of Food Science 1981, 46, 150–155.
- Şakiroğlu, H. Kuşburnu meyvasından izole edilen polifenol oksidaz enziminin kinetik ve elektroforetik özelliklerinin incelenmesi (Investigation of kinetics and electrophoretic properties of the polyphenol oxidase enzyme isolated from rosehip fruit). PhD thesis, Atatürk University, Turkiye, 1994.
- Hielscher, Ultraschall-Technologie. UP200S/UP400S Betriebsanleitung, ultraschall prozessoren für Laboranwendungen (Operating Instructions, ultrasonic processors for laboratory applications). Germany, 2007.
- Karaman, S.; Yilmaz, M.T.; Ertugay, M.F.; Başlar, M.; Kayacier, A. Effect of ultrasound treatment on steady and dynamic shear properties of glucomannan based salep dispersions: Optimization of amplitude power, sonication time, and temperature using response surface methodology. Ultrasonic Sonochemistry 2012, 19, 928–938.
- Toğrul, H.; Arslan, N. Mathematical model for prediction of apparent viscosity of molasses. Journal of Food Engineering 2004, 62, 281–289.
- Davies, M.J.; Truscott, R.J.W. Photo-oxydation of proteins and its role in cataractogenesis. Journal of Photochemistry and Photobiology B: Biology 2001, 63, 114–125.
- Krivandin, A.V.; Muranov, K.O.; Yakovlev, F.Y.; Poliansky, N.B.; Wasserman, L.A.; Ostrovsky, M.A. Resistance of a-crystallin quaternary structure to UV irradiation. Biochemistry (Moscow) 2009, 74, 633–642.
- Cheng, X.-F.; Zhang, M.; Adhikari, B. The inactivation kinetics of polyphenol oxidase in mushroom (Agaricus bisporus) during thermal and thermosonic treatments. Ultrasonics Sonochemistry 2013, 20, 674–679.
- Mawson, R.; Gamage, M.; Terefe, N.S.; Knoerzer, K. Ultrasound in Enzyme Activation and Inactivation, Ultrasound Technologies for Food and Bioprocessing; Feng, H.; Barbosa-Canovas, G.V.; Weiss, J.; Eds.; Springer: New York, 2011.
- Liu, Y.; Hu, X.; Zhao, X.; Song, H. Combined effect of high pressure carbon dioxide and mild heat treatment on overall quality parameters of watermelon juice. Innovative Food Science & Emerging Technologies 2012, 13, 112–119.