Abstract
Aspergillus niger, an isolate of soil contaminated with effluents from cotton ginning mill was grown in Czapek-Dox medium containing sawdust, Triton-X 100 and urea for production of an extracellular β-glucosidase. β-Glucosidase enzyme was purified (86-fold) from culture filtrate of A. niger by employing ammonium sulphate precipitation and gel filtration on sephadex G-75. The molecular mass of the purified enzyme was estimated to be 95 kDa by sodium dodecyl sulphate polyacrylamide gel electrophoresis. The enzyme had an optimal activity on p-nitrophenyl β-D-glucopyranoside at 50°C and pH 5.0. The Km and Vmax of the enzyme on p-nitrophenyl β-D-glucopyranoside at 50°C and pH 5 were 8.0 mM and 166 µmol/min/mg of protein, respectively. The enzyme could hydrolyze cellobiose and lactose but not sucrose. Heavy metals like Hg2+, Al3+, and Ag+ inhibited the activity, whereas Zn2+ and detergents such as Triton-X 100 and Tween-80 increased the activity at 0.01%. The enzyme activity increased in the presence of methanol and ethanol.
INTRODUCTION
β-D-Glucosidases (β-D-glucoside glucohydrolase, EC 3.2.1.21) constitute a heterogeneous group of enzymes and catalyze hydrolysis of alkyl and aryl β-glucosides as well as disaccharides and oligosaccharides.[Citation1] Their distributions are widespread in biological living world—plants, animals, fungi, and bacteria.[Citation2] The physiological roles postulated for β-glucosidase are extremely diverse—glucoside glycolipid catabolism in human tissue, cell wall pigment, cyanoglucoside metabolism, and defense against pathogens in plants. In recent years, β-glucosidases have gained importance for utilization of cellulose and oligosaccharide substrates by fungi and bacteria for production of fuel ethanol.[Citation3,Citation4] These enzymes are widely used in various biotechnological processes.[Citation5,Citation6] In the flavor industry, they facilitate releasing of flavor generating aroma compounds from glucosidic precursors present in fruits and fermentation products.[Citation7–Citation10] Supplementation of exogenous β-glucosidase enhanced not only glucose yields but also ethanol to certain extent.[Citation5] In view of the involvement of β-glucosidase in various applications, its production and characterization are gaining much importance. Growth of Aspergillus niger, isolated from soil contaminated with effluents from a cotton ginning mill[Citation11] on a medium supplemented with saw dust yielded higher production of β-glucosidase. In the present study, an extracellular β-glucosidase was purified from the culture filtrate of A. niger and characterized for its kinetic properties.
MATERIALS AND METHODS
Organism and Culture Conditions
Aspergillus niger used in this study was an isolate from soil contaminated with effluents of cotton ginning industry.[Citation11] The fungus was maintained on potato dextrose agar (PDA) plates. For enzyme production, A. niger was grown in Czapek-Dox liquid medium (g/L. sucrose, 30; NaNO3, 1.0; K2HPO4, 1.0; MgSO4.7H20, 0.05; KCl, 0.5; FeSO4.7H20, 0.01; pH 5.0) containing 1% saw dust, 0.3% urea, and 0.01% Triton-X 100 as surfactant. Fifty milliliter aliquots of liquid medium contained in Erlenmeyer flasks (250 mL) were inoculated with 2 × 106 viable conidial spores obtained from 7-day old A. niger grown on PDA and suspended in 0.015% Triton-X 100 (v/v). The culture was incubated at 28°C for 7 days on an orbital shaker (180 rpm) and was harvested by filtration through Whatman filter paper No. 1. The filtrate was pooled and used as the crude β-glucosidase preparation.
Enzyme Assay
β-Glucosidase activity in the crude culture filtrate and different fractions was determined in assay mixtures containing p-nitrophenyl β-D-glucopyranoside (PNPBG; Merck) at 5 mM in 0.05 M citrate buffer (pH 5.0) and aliquots of diluted enzyme source with appropriate controls.[Citation12] After incubation for 30 min at 50°C, the reaction was terminated by adding 4 mL of 0.05 M NaOH-glycine buffer (pH 10.6) and p-nitrophenol produced was determined in a Spectronic-20D spectrophotometer (Milton Roy) at 420 nm. All assays were performed in duplicates. One unit of β-glucosidase activity was defined as the amount of enzyme required to release one micromole of p-nitrophenol per minute under assay conditions. Protein was measured by method of Lowry et al.[Citation13,Citation14] with bovine serum albumin as standard.
Enzyme Purification
All steps for the enzyme purification were carried out at 4°C. β-Glucosidase was precipitated from crude filtrate by adding 90% (w/v) powdered ammonium sulphate to the culture filtrate and kept overnight at 4°C. The resulting precipitate was collected by centrifugation (Remi C-24) at 10,000 g for 30 min at 4°C. The precipitate was suspended in 0.05 M citrate buffer (pH 5.0) and dialyzed in a bag (Sigma 10–60 kDa) overnight against the same buffer. During the course of dialysis the buffer was frequently changed until no traces of ammonium were found in the buffer upon testing with Nessler’s reagent. The dialyzed fraction was then subjected to gel filtration on sephadex G-75 (Sigma) pre-equilibrated with 0.05 M citrate buffer (pH 5.0). One milliliter fractions of elute in the same buffer were collected in test tubes in an automatic fraction collector (Biorad Model-2110) at 4°C and the absorbance of 1 mL fractions was measured at 280 nm in UV-visible spectrophotometer (Chemito) for protein content. Aliquots from these fractions were tested for β-glucosidase activity under standard conditions and only fractions (25 to 35) with the enzyme activity were pooled and concentrated. The above two steps of purification yielded the enzyme almost to its homogeneity.
Sodium Dodecyl Sulphate Polyacrylamide Gel Electrophoresis (SDS-PAGE)
Electrophoresis was performed to find out apparent molecular mass of β-glucosidase using 12% polyacrylamide gel in a mini system (Biotech India) as described by Laemmli et al.[Citation15] The denatured protein along with molecular markers (Pharmacia)—phosphorylase (97 kDa), bovine albumin (66 kDa), ovalbumin (45 kDa), carbonic anhydrase (30 kDa), soybean trypsin inhibitor (20 kDa), and α-lactalbumin (14 kDa) was electrophoresed at 50 V for 2–3 h. Developed gels were stained with either Coomassie Blue R-250 or silver staining[Citation16] according to manufacturer’s instructions.
Characterization of β-Glucosidase
Kinetic parameters of β-glucosidase were carried out by adding different concentrations of PNPBG within a range of 0.5–30 mM to the reaction mixture and the rate of p-nitrophenol released was determined at pH 5.0 and 50°C as described previously. The values of the Michaelis constant (Km) and the maximal velocity (Vmax) were calculated from Lineweaver-Burk plots.[Citation17]
The effect of pH on β-glucosidase activity was determined by assaying with the substrate—PNPBG at 5 mM concentration at 50°C and desired pH (3, 5, 7, 9) maintained with use of the four buffers system of the same molarity (50 mM)—acetate, citrate, phosphate, and tris, respectively. p-Nitrophenol liberated in the reaction mixture was spectrophotometrically quantified as described earlier. The effect of temperature on β-glucosidase activity was determined by assaying with PNPBG at different temperatures within a range of 30 to 90°C and pH 5.0. p-Nitrophenol released was determined spectrophotometrically as mentioned earlier.
The influence of various metal ions and EDTA at 1 mM on β-glucosidase activity with PNPBG was determined by pre-incubating the enzyme with the inhibitors/effectors in 50 mM citrate buffer (pH 5.0) at 30°C for 30 min. The amount of p-nitrophenol liberated during the assay was measured as described earlier and assay system without any metal ions/EDTA served as control.
Selected disaccharides at only 30 mM concentration were included in the reaction mixture in place of PNPBG to test for their hydrolysis by the purified enzyme. The reducing sugar liberated was measured by dinitrosalicylic acid method.[Citation18] The activity of the enzyme is expressed in units and one unit is equivalent to one micromole of reducing sugar released per minute under assay conditions.[Citation19] The effect of organic solvents such as methanol and ethanol at the final concentration of 30% (v/v) on the enzyme activity was also tested. The reaction mixture, containing the enzyme and PNPBG, was incubated at 50°C and pH 5.0 for 30 min. The enzyme activity, assayed in citrate buffer alone without an organic solvent, was taken as 100%. Triton X-100, Tween-80, or sodium dodecyl sulphate (SDS) at 0.015% was added to the reaction mixture containing PNPBG at 5 mM level in 0.05M citrate buffer (pH 5.0). The reaction mixture without surfactant served as control. p-Nitrophenol released from the substrate was quantified as described earlier.
RESULTS AND DISCUSSION
Following a two-step protocol-(NH4)2SO4 precipitation and gel filtration, an extracellular β-glucosidase was purified to homogeneity from the culture filtrate of A. niger grown on Czapek-Dox medium with saw dust for 7 days (). The fraction derived from precipitation by adding 90% (NH4)2SO4 to the crude fraction contained the bulk (63%) of total enzyme activity. The fraction was further resolved on sephadex G-75 column by gel permeation chromatography into three peaks as shown in . The first sharp peak exhibited β-glucosidase activity. Fractions from 25 to 35 corresponding to the first peak were pooled together and this pooled fraction was purified β-glucosidase. The purification of β-glucosidase was 86-fold with 36% recovery of initial and total activity in the crude fraction. The specific activity of the purified enzyme was 60.6 U/mg of protein ().
TABLE 1 Summary of purification of β-glucosidase
Similarly, extracellular β-glucosidase from the culture filtrates of various fungi was purified following a variety of protocols. For instance, β-glucosidase was partially purified by 17 or 38 folds from culture filtrate of Bipolaris sonokiniana and Xylaris regalis by liquid chromatography on Sepharose-CLAB.[Citation20,Citation21] Seven forms of β-glucosidase from different Aspergillus species were purified using anion exchange chromatography and gel filtration.[Citation22] β-Glucosidase was purified to homogeneity by 129 and 1800 folds from the culture filtrate of Aureobasidium pullulans and Candida peltata.[Citation23,Citation24] The purification of extraceullar β-glucosidase from Trichoderma pseudokoningi and A. niger involved a three-step procedure.[Citation25,Citation26]
The specific activity of purified β-glucosiciase of A. niger in the present study was 60.60 U/mg of protein (). Similarly, purified β-glucosidase from various organisms such as A. niger CCRC 31494, A. oryzae, C. peltata, and A. pullulans had specific activity within a range of 100–1000 U/mg of protein under standard assay conditions.[Citation23,Citation24,Citation27,Citation28,]
The molecular mass of denatured β-glucosidase was estimated to be around 95 kDa by SDS-PAGE analysis in the present study (). SDS-PAGE of the purified β-glucosidase of Acremonium persicinum gave a single band with an apparent molecular mass of 128 kDa.[Citation29] The major form of β-glucosidase of A. oryzae had a molecular mass of 130 kDa by SDS-PAGE analysis.[Citation28] β-Glucosidases from different strains of A. niger had molecular weight within a range of 68–220 kDa by SDS-PAGE. The difference in the molecular weight of β-glucosidase in different organisms could be due to the presence of multiple forms of the enzyme and glycosylation of the enzyme.
FIGURE 2 Determination of molecular mass of β-glucosidase from Aspergillus niger by SDS-PAGE. Lane 1: Protein markers phosphorylase (97 kDa), bovine albumin (66 kDa), ovalbumin (45 kDa), carbonic anhydrase (30 kDa), soybean trypsin inhibitor (20 kDa), and α-lactabumin (14 kDa). Lane 2: Purified enzyme.
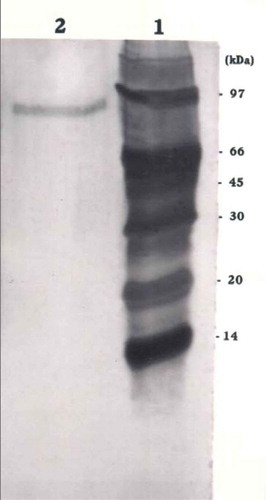
Double reciprocal plot of substrate concentration and initial rate of hydrolysis yielded a straight line () and Km and Vmax of β-glucosidase on the substrate PNPBG were found to be 8 mM and 166 U/min/mg of protein, respectively. The maximal velocity around 300 U/mg of protein was recorded in respect of β-glucosidase from organisms such as Chalara paradoxa, Cladosporiurn resinae, and Xylaria regalis.[Citation20,Citation30,Citation31] The Vmax values of β-glucosidase from A. niger and A. oryzae were 1240 and 3040 µmol/min/mg of protein, respectively.[Citation28,Citation32] The maximum activity of β-glucosidase (1.38 U) was recorded at pH 5.0 in the present study (). Similarly, β-glucosidase from X. regalis, Lycoperdon pyriforme, A. niger, and Metschnikowia pulcherrima exhibited the maximum activity at pH 5.0 and the least activity at pH 9.0 and 4.0.[Citation20,Citation33–Citation35] Purified form of β-glucosidases of Volvareilla volvacea displayed a broad pH optimum between 6.2 to 7.4 for β-glucosidase I and 5.4–6.6 for β-glucosidase II.[Citation36] β-Glucosidases of other organisms such as B. sonokiniana,[Citation21] A. pullulans,[Citation23] A. persicinum,[Citation29] Sporotrichum thermophile,[Citation37] C. peltata,[Citation24] different strains of A. niger,[Citation27,Citation32,Citation38] Cladosporium resinae,[Citation31] Armillaria mellea,[Citation39] and Tolipocladium cyclindrosporum[Citation40] had an optimal pH falling within a range of 2.4 to 5.
The activity of β-glucosidase from A. niger was maximal (0.132 units) at 50°C and declined at temperatures above 50°C (). Similarly, β-glucosidase of L. pyriforme,[Citation33] A. niger,[Citation34] Stachybotrys strain,[Citation41] V. volvaciae,[Citation42] and A. mellea[Citation39] had optimum temperature of 50°C. β-glucosidase of A. perscinum,[Citation29] C. resinae,[Citation31] A. niger,[Citation27,Citation32] C. peltata,[Citation24] and Melanocarpus sp.[Citation43] had optimal temperature following within a range of 50–60°C. But optimal temperature of β-glucosidases from S. thermophile, A. pullulans, and A. niger was 65, 75, and 65°C, respectively.[Citation23,Citation37,Citation38]
β-Glucosidase activity was affected by the presence of specific certain metal ions (). Mn2+ stimulated the enzyme activity by 20% over control whereas Hg2+, Ag+, and Al3+ inhibited the enzyme activity by 10–30%. Similar observation was made with β-glucosidase of the buffalo rumen fungus Neocallimastix patriciarum.[Citation44] Activity of β-glucosidase was unaffected by the presence of EDTA and Mg2+. A similar inhibitory effect was reported by Hg2+ and Ag+ on β-glucosidase of A. niger,[Citation38] C. paradoxa,[Citation30] C. resinae,[Citation31] X. regalis.[Citation20] All metal ions except Mn2+ had a negative effect on activity of β-glucosidase of A. mellea.[Citation39] The innocuous effect of EDTA in the present study is well in agreement with the results from other studies.[Citation23,Citation24] Differential effect of metal ions on β-glucosidase could be due to conformational changes caused by binding of metal ions at different sites of enzyme.[Citation45]
TABLE 2 Influence of effectors and inhibitors on β-glucosidase assay
The enzyme could hydrolyse cellobiose with a maximum activity of 1.52 U. Lactose was also an effective substrate for β-glucosidase and was hydrolyzed with an activity of 1.05 U. No significant activity was observed with sucrose. β-Glucosidase appears to require fulfillment of β-configuration of glucose unit in substrate for display of its action. No activity by β-glucosidase against sucrose in the present study and other studies could be attributed to α-configuration of glucose unit in sucrose. β-Glucosidase of X. regalis exhibited activity against disaccharides such as laminariobiose, sophorose, and gentiobiose but not sucrose.[Citation20] Among different substrates with p-nitrophenyl moiety conjugated to different sugars tested, the maximum activity was observed with PNPBG in respect of β-glucosidase of A. mellea.[Citation29] C. peltata had a greater activity against cellobiose, laminariobiose, and gentiobiose.[Citation24] β-Glucosidase of C. resinae cleaved glycosidic bonds in cellobiose, lamanariobiose, and sophorose but failed to act on gentiobiose.[Citation31]
Methanol and ethanol increased the activity of β-Glucosidase by 80 and 30%, respectively, in comparison to control (). Similarly, ethanol and methanol at 30% (v/v) concentration stimulated the activity of β-glucosidase of C. paradoxa by 3- and 2-fold, respectively.[Citation30] The stimulatory response of organic solvents toward the activity of β-glucosidase from A. niger,[Citation27] Botrytis cinera,[Citation46] C. peltata,[Citation24] and Thermatoga sp.[Citation47] has been attributed to glucosyl transferase activity of the enzyme in using ethanol as a suitable acceptor instead of water.
The enzyme activity was stimulated by Triton X-100 and Tween-80 by two fold, whereas the activity remained unaffected by SDS (). In contrast, the activity of β-glucosidase of C. paradoxa was inhibited by SDS, Tween-80, and Triton X100.[Citation30] Similar inhibitory effect of SDS was reported on activity of β-glucosidase from C. resinae,[Citation30] X. regalis,[Citation20] Pericornia sp.,[Citation48] and V. volvacea[Citation42] within a range of 0.1 to 1%. A final concentration of 0.75% SDS showed 100% inhibitory effect on β-glucosidase of L. pyriforme.[Citation33]
CONCLUSIONS
The fungal isolate Aspergillus niger was grown in liquid culture on a simple medium containing cheaply available raw material, saw dust, for production of β-glucosidase. β-Glucosidase was purified from the culture filtrate by simple two-step procedure for biotechnological applications. β-glucosidase of A. niger has a molecular weight of 95 kDa and displayed maximum activity at pH of 5.0 and 50°C and has kinetic properties with Km of 8.0 mM and Vmax of 166 µmol/min/mg protein.
ORCID
Golla Ramanjaneyulu
http://orcid.org/0000-0002-8595-9899Bontha Rajasekhar Reddy
http://orcid.org/0000-0001-6665-3627REFERENCES
- Lecleric, M.; Arnaud, A.; Ratomahenina, R.; Galaxy, P. Yeast β-Glucosidases. Biotechnology & Genetic Engineering Reviews 1987, 5, 269.
- Woodward, J.; Wiseman, A. Fungal and Other β-D-Glucosidase Their Properties and Application. Enzyme and Microbial Technology 1982, 4, 73.
- Esen, A. β-Glucosidases: Overview. In β-glucosidases - Biochemistry and Molecular Biology; Esen, A.; Ed.; ACS Symposium Series 533; American Chemical Society, Washington, DC, 1993, 1 pp.
- Krisch, J.; Tako, M.; Papp, T.; Vagvölgyi, C. Characteristics and Potential Use of β-Glucosidases from Zygomycetes. In Current Research, Technology and Education Topics in Applied Microbiology and Microbial Biotechnology; Mendez-Vilas, A.; Ed.; FORMATEX Research Centre: Badajoz, Spain, 2010.
- Xin, Z.; Yinbo, Q.; Peiji, G. Acclimation of Ethanol Production from Paper Mill Waste Fiber by Supplementation with β-glucosidase. Enzyme Microbial Technology 1993, 15, 62.
- Bothast, R.J.; Saha, B.C. Ethanol Production from Agricultural Biomass Substrates. Advances in Applied Microbiology 1997, 44, 261.
- Roitner, M.; Schalkhammer, T.; Pittner, F. Characterization of Naringinase from Aspergillus niger. Monatshe fur Chemie 1984, 115, 1255.
- Shoseyov, O.; Bravdo, B.A.; Ikan, R.; Chet, I. Immobilized Endo—β-Glucosidase Enriches Flavour of Wine and Passion Fruit Juice. Journal of Agriculture and Food Chemistry 1990, 27, 1973.
- Gueguen, Y.; Chemardin, P.; Janbon, G.; Arnaud, A.; Galaxy, P.A. Very Efficient β-Glucosidase Catalyst for Hydrolysis of Flavor Precursors of Wines and Fruit Juices. Journal of Agriculture and Food Chemistry 1996, 44, 2336.
- Martino, A.; Schiraldi, C.; di lazzaro, A.; Fume, I.; Spagna, G.; Piffers, P.G.; De Rosa, M. Improvement of the Flavor of Falanghina White Wine Using a Purified Glucosidase Preparation from Aspergillus niger. Process Biochemistry 2000, 36, 93.
- Narasimha, G.; Babu, G.V.A.K.; Rajasekhar, R.B. Effect of Effluents of Cotton Ginning Industry on Physico Chemical and Biological Properties of Soil. Asian Journal of Microbial Biotechnology and Environmental Science 1999, 1, 89.
- Herr, D. Secretion of Cellulases and β-Glucosides by Trichoderma viridae TTCC 1433 in Submerged Cultures on Different Substrates. Biotechnology and Bioengineering 1979, 21, 1361.
- Lowry, O.M.; Rosenbrough, N.J.; Farr, A.L.; Randall, R.J. Protein Measurement with Folin Phenol Reagent. Journal of Biological Chemistry 1951, 193, 265.
- Nagihan, S.E.; Ayse, T.; Melike, Y.A.; Sabana, K. Purification and Characterization of Armillaria Mella β-Glucosidase. International Journal of Food Properties 2014, 17, 678–689.
- Laemmli, U.K. Cleavage of Structural Proteins during the Assembly of the Head of the Bacteriophage T4. Nature 1970, 224, 80.
- Heukeshoven, J.; Dernic, R. Improved Silver Staining Procedure Fast Staining in Plant System Development Unit. Staining of Sodium Dodecyl Sulfate Gels. Elctrophoresis 1988, 9, 28.
- Lineweaver, H.; Burk, D. The Determination of Enzyme Dissociation Constants. Journal of the American Chemical Society 1934, 56(3), 658–666.
- Miller, G.L. Use of Dinitrosalicylic Acid Reagent for Determination of Reducing Sugars. Analytical Chemistry 1959, 31, 426.
- Turan, Y.; Zheng, M. Purification and Characterization of An Intracellular β-Glucosidase from the Methylotrophic Yeast Pichia Pastoris. Biochemistry (Moskow) 2005, 70, 1363–1368.
- Wei, D.L.; Kirimura, K.; Usami, S.; Lin, T.H. Purification and Characterization of An Extracellular β-Glucosidase from the Wood Grown Fungus Xylaria Regalis. Current Microbiology 1996, 33, 297.
- Geimba, M.P.; Rife, A.; Agostini, V.; Brandilli, A. Characterization of Cellulase Hydrolyzing Enzymes from Biopolaris Sonokiniana. Journal of the Science of Food and Agriculture 1999, 79, 1848.
- Decker, C.H.; Visser, J.; Schreier, P.B. β-Glucosidases from Five Black Aspergillus Species: Study of Their Physico-Chemical and Biocatalytic Properties. Journal of Agriculture and Food Chemistry 2000, 48, 4929.
- Saha, B.C.; Freer, S.N.; Bothast, R.J. Production, Purification, and Properties of a Thermostable Beta Glucosidase from a Colour Variant Strain of Aureobasidium Pullulans. Applied and Environmental Microbiology 1994, 60, 3774.
- Saha, B.C.; Bothast, R.J. Production and Purification and Characterization of a Highly Glucose Tolerant Novel Beta-Glucosidase from Candida peltata. Applied and Environmental Microbiology 1996, 62, 3165.
- Dong, W.; Yinbo, Q.; Peiji, G. Primary Studies on Several Cellulase Components with Special Characteristics Purified from Trichoderma Pseudokoningii S.38. Biotechnology Applied Biochemistry 1997, 25, 181.
- Ashadi, R.W.; Shimakowa, K.; Ogawa, K. The Mechanism of Enzymatic Cellulose Degradation (1). Purification and Some Properties of Cellulolytic Enzymes from Aspergillus Niger UC. Journal of General and Applied Microbiology 1996, 42, 93.
- Yan, T.; Lin, C.L. Purification and Characterization of a Glucose Tolerant β-Glucosidase from Aspergillus Niger, CCRC 3494. Bioscience, Biotechnology, and Biochemistry 1997, 61, 965.
- Riou, C.; Salmon, J.; Vanier, M.; Gunata, Z. Purification, Characterisation, and Substrate Specificity of a Novel Highly Glucose-Tolerant β-Glucosidase from Aspergillus Oryzae. Applied Environmental Microbiology 1998, 64, 3607.
- Pitson, S.M.; Seviour, R.J.; McDugall, B.M. Purification and Characterization of An Extracellular β-Glucosidase from the Filamentus Fungus Acremonium Persicinum and Its Probable Role in β-Glucan Degradation. Enzyme Microbial Technology 1977, 21, 182.
- Lucas, R.; Robles, A.; Cienfuegos, G.A.D.; Galvez, A. β-Glucosidase from Chalara Paradoxa CH 32. Purification and Properties. Journal of Agriculture and Food Chemistry 2000, 48, 3698.
- Oh, K.; Hamada, K.; Saito, M.; Lee, H.; Matsuoka, H. Isolation and Properties of Extracellular β-Glucosidase from Filamentous Fungus, Cladosporium Resinae Isolated from Kerosene. Bioscience, Biotechnology, and Biochemistry 1999, 63, 281.
- Yan, T.; Lin, Y.; Lin, C.L. Purification and Characterization of An Extracellular β-Glucosidase II with High Hydrolysis and Transglucosylation Activities from Aspergillus Niger. Journal of Agriculture and Food Chemistry 1998, 46, 43.
- Akatin, Y.M. Characterization of a β-Glucosidase from An Edible Mushroom, Lycoperdon Pyriforme. International Journal of Food Properties 2013, 16(7), 1565–1577.
- Peshin, A.; Mathur, J.M.S. Purification and Characterization of β-Glucosidase from Aspergillus Niger Strain 32. Letters in Applied Microbiology 1999, 28, 401–404.
- Gonzalez-Pombo, P.; Perez, G.; Carrau, F.; Guisan, J.M.; Batista-Viera, F.; Brena, B.M. Onestep Purification and Characterization of An Intracellular β-Glucosidase from Metschnikowia Pulcherrima. Biotechnology Letters 2008, 30, 1469–1475.
- Cai, Y.J.; Buswell, J.A.; Change, S.T. β-Glucosidase Components Of Cellulolytic System Of The Edible Straw Mushroom, Volvariella Volvaceae. Enzyme Microbial Technology 1998, 22, 122.
- Bhat, M.K.; Gaikwad, J.S.; Maheswari, R. Purification and Characterization of An Extracellular β-Glucosidase from the Thermophilic Sporotricum Thermophile and Its Influence on Cellulase Activity. Journal of General Microbiology 1993, 139, 2825.
- Galas, E.; Romanowska, I. Purificaion and Some Properties of Beta Glucosidase from Aspergillus Niger 1BT-90. Acta Microbiologica, Polonica 1997, 46, 241.
- Nagihan, S.E.; Ayse, T.; Melike, Y.A.; Sabana, K. Purification and Characterization of Armillaria Mella β-Glucosidase. International Journal of Food Properties 2014, 17, 678–689.
- Yi-bo, Z.; Yuan, L.-J.; Chen, Z.-J., Fu, L., Lu, J.-H.; Meng, Q.-F.; He, H.; Yu, X.-X.; Lin, F.; Teng, L.-R. Purification and Characterization of β-Glucosidase from a Newly Isolated Strain Tolypocladium Cylindrosporum Syzx4. Chemistry Research Chinese Universities 2011, 27(4), 557–561.
- Amouri, B.; Gargouri, A. Characterization of a Novel β-Glucosidase from a Stachybotrys Strain. Biochemical Engineering Journal 2006, 32, 191–197.
- Li, X.; Pei, J.; Wu, G.; Shao, W. Expression, Purification, and Characterization of a Recombinant β-Glucosidase from Volvariella Volvacea. Biotechnology Letters 2005, 27, 1369–1373.
- Kaur, J.; Chanda, B.S.; Kumar, B.A.; Kaur, G.S.; Saini, H.S. Purification and Characterization of β-Glucosidase from Melanocarpus sp. MTCC 3922, Electronic Journal of Biotechnology 2007, 10(2) 260–270.
- Chen, H.L.; Chen, Y.C.; Lu, M.Y.J.; Lui, J.C.; Wang, H.T.C.; Ke, H.M.; Wang, T.Y.; Ruan, S.K.; Wang, T.Y.; Hung, K.Y.; Cho, H.Y.; Lin, W.T.; Shih, M.C.; Li, W.H. A Highly Efficient β-Glucosidase from the Buffalo Rumen Fungus Neocallimastix Patriciarum W5, Biotechnology for Biofuels 2012, 5, 24.
- Ditusa, C.A.; Christensen, T.; Mccall, K.A.; Fierke, C.A.; Toone, E.J. Thermodynamics of Metal Ion Binding.1. Metal Ion Binding by Wild-Type Carbonic Anhydrase. Biochemistry 2001, 40, 5338–5344.
- Gueguen, Y.; Chemardin, P.; Arnaud; Galzy, P. Purification and Characterization of An Intracellular β-Glucosidase from Botrytiscinera. Enzyme Microbial Technology 1995, 17, 900.
- Ruttersmith, L.D.; Daniel, R.M. Thermostable β-Glucosidase and β-Xylono-Sidase from Thermatoga sp. Strain Fiss 3–181. Biochemica et Biophysica Acta 1998, 1156, 167.
- Harnpicharnchai, P.; Champreda, V.; Sornlake, W.; Eurwilaichitr, L. A Thermotolerant ß-Glucosidase Isolated from An Endophytic Fungi, Periconia sp., with a Possible Use for Biomass Conversion to Sugars. Protein Expression and Purification 2009, 67, 61–69.