Abstract
The aim of this study was the experimental determination of the thermal conductivity of mango pulp of cultivar “ubari” and its subsequent comparison with values estimated using different structural models, such as the serial, parallel, and Maxwell-Eucken models. A linear heating probe calibrated with a solution of 10% sucrose was utilized. Heat was applied to the probe, generating an increase in temperature, which was recorded through a signal conditioning system. The thermal conductivity of the pulp was calculated from the slope obtained by linear regression of the natural logarithm of the temperature versus time. The data was within the range of 0.465 to 1.595 W/m.K for temperatures of 0 and –30°C, respectively, showing an increase of conductivity with freezing. The initial freezing temperature and ice fraction were determined at every temperature of analysis for further use in the models. The best fit to the experimental data were obtained using the Maxwell-Eucken model, yielding minimum and maximum errors of 10.8 and 17.8%, respectively. An exponential model was fitted, which allowed for the direct calculation of the thermal conductivity of mango pulp using only the knowledge of the recorded temperature during thermal processing.
INTRODUCTION
The preservation of fruits in the form of pulp, juices, and other products has been developed to increase out-of-season supply and allow for the use of surplus production. The frozen fruit pulp is the product obtained from the edible part of the fruit after crushing and/or pulping and preservation by freezing. Frozen fruit pulp is almost always used as raw material for processing other products, such as nectars, juices, jams, ice creams, and sweets.[Citation1]
Mango is considered an important tropical fruit due to its excellent flavor, aroma, and characteristic staining. However, due to its seasonality, industrialization is required so as to allow for out-of-season availability in addition to providing better utilization and reduction of production losses. It is a widely accepted fruit on the market, besides being rich in vitamin C, with values ranging from 66.5 mg.100 g–1 in fruit “green,” 43.0 mg.100 g–1 in ripe fruit,[Citation2] and up to 110 mg.100 g–1, depending on the variety.[Citation3]
The cultivar Uba is currently the most used variety in the mango juice agribusiness in Brazil, presenting a pulp yield of approximately 69%, which is approximately 10% lower than the pulp yield of cultivar “Ubarí.” The need to accurately know the thermal properties of mango pulp has stimulated the study of the influence of the composition and temperature of the food on these properties.[Citation4] One of the most important properties for a process involving heat transfer is the thermal conductivity, as during a heating or cooling treatment, changes in the structure as well as the physical and chemical properties of the food may occur.[Citation5] The thermal conductivity is a property highly dependent on the composition of the food and the fraction of ice present.[Citation6,Citation7]
Predictive models and new methods of determination were developed, and the hot wire probe method is the most widely used for food.[Citation8–Citation10] However, there are few data on the thermophysical properties of the pulps of tropical fruits in the literature, especially at low temperatures. According to Carson[Citation8] and Delgado et al.[Citation11] the fraction of frozen water present during the freezing of a food greatly affects its thermal conductivity, due to the conductivity of the ice being four times that of liquid water. The processing of mango derivatives as pulp or slices deserves attention, given its use as feedstock for secondary industries or for direct consumption.[Citation1]
The present work was proposed based on two considerations: the importance of the use of surplus production, the potential for industrialization and consumption of pulp and mango juice, and the scarcity of data regarding its thermophysical properties. The objectives were the experimental determination of the apparent thermal conductivity of mango pulp (Mangifera indica L.) cultivar “Ubari,” its comparison with estimated values from structural models, such as serial, parallel and Maxwell-Eucken or Dispersed, and the fitting of equations for direct evaluation of this property as a function of temperature during thermal processing.
MATERIALS AND METHODS
Sample Preparation for Analysis of Thermal Conductivity
The fruits were sanitized and the pulp was extracted with an electric depulper (Macanuda, Joinville, Santa Catarina), with a sieve opening of 0.5 mm. The chemical composition presented in [Citation12] was used.
Probe Construction, Calibration, and Experimental Setup
The probe was constructed with a hypodermic needle with an external diameter of 0.6 mm and length of 70 mm, into which was placed a resistance heating wire (nickel-chromium; Omega Engineering Inc., Stamford, CT, USA) with a length of 0.36 m and diameter of 0.08 mm, together with a small type T thermocouple AWG 30 (Omega Engineering Inc.) located at the exact center of the probe. These components were insulated with epoxy resin. The probe was calibrated for temperature and thermal conductivity.[Citation13] First, the probe calibration was performed with respect to the temperatures of analysis (–30, –25, –20, –15, –10, –5, and 0°C). A thermometer was also inserted in the sample with the probe, and the measured values for the two instruments were correlated. After the temperature calibration, the probe was further calibrated using a solution of 10% sucrose (weight/volume). Its conductivity was determined under the same conditions as used in the pulp and then compared with theoretical values from the literature. The values were 1.81, 1.78, 1.74, 1.71, and 1.65 W/mK for the thermal conductivity of 10% sucrose at temperatures of –30, –25, –20, –15, and –10°C, respectively.[Citation14] With the calibration, a correction factor f was obtained as shown in Eq. (1).[Citation13]
For conductivity measurements, the probe was then inserted into the geometric center of the pulp, initially maintained at a fixed temperature, in a 250 mL beaker set in an ultra-thermostatic bath (model 521/3DE; Nova Ética, Vargem Grande Paulista, Brazil). The visualization of the entire apparatus is shown in .[Citation13]
FIGURE 1 Experimental setup of the hot wire probe for determining the thermal conductivity of the pulps: (1) Sample; (2) Probe heating; (3) Ultra thermostatic bath; (4) Current source; (5) Signal conditioning system; (6) Microcomputer; (7) Ammeter; (A) Constantan wire; (B) Copper wire; (C and D) Feeder of the probe resistance.
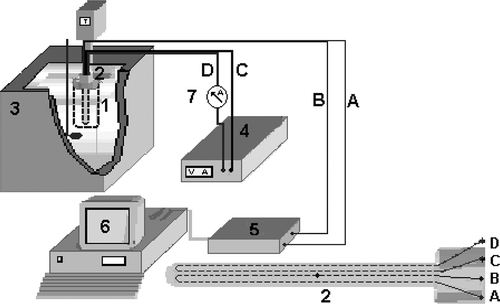
Mathematical Analysis and Theoretical Models for the Evaluation of Thermal Conductivity
The experiment with the hot wire probe is based on the application of a constant heat flux from the heat source to the material, initially at equilibrium, thereby generating a variation in temperature of To to T.[Citation13,Citation15–Citation18]
To review the theoretical thermal conductivity, the existence of a relationship between the effective thermal conductivity of a food with the intrinsic conductivities of its components and their individual volume fraction is assumed, as described by Eq. (2).
where, is the effective thermal conductivity of the food,
are the values of the intrinsic thermal conductivities of the components and
,
,
,… are the volume fractions of each component.[Citation19] The volume fractions can be determined according to Eq. (3).
where, is the mass fraction of each component and
The parameters used to determine the thermal conductivities and densities of the pure components are obtained from ASHRAE.[Citation20] To determine the molar fractions of ice at each temperature, Eqs. (4) and (5) proposed by Heldman and Lund[Citation21] were used.
where, is the molar fraction of unfrozen water;
is the freezing initiation temperature of the food (K);
is the initial freezing of water (273.15 K);
is the temperature of the food (K); Rg is the universal gas constant (8.314 kJ/kg.mol.K);
is the latent heat of fusion of water (333.6 kJ/kg).
where, mwo (T) is the mass of water that does not freeze at the temperature (T), ms is the effective mass of the solids, and Mw and MS are the molecular weights of water and solid components, respectively. Thus, if the initial freezing temperature of the food is known (TfS), by substituting T for TfS in Eq. (4), the effective molecular weight of the solid components (Ms) can be determined by substituting Eq. (4) into Eq. (5). The fraction of unfrozen water at a temperature T of analysis can then be determined by Eq. (4); substituting the result into Eq. (5) finally determines the mass of unfrozen water. The mass of ice is then determined by the difference between the total mass of water and the mass of unfrozen water at temperature T.
Parallel Model
In this model, the thermal conductivity is calculated by summing the product of the thermal conductivity with the volume fraction of each component according to Eq. (6).[Citation19] For a multiphase system in parallel, as shown in , the effective thermal conductivity is calculated as:
Serial Model
In this model, the phases are in series or in the direction perpendicular to heat flow, which leads to high resistance to heat flow. For a multiphase system in series, as shown in , the thermal conductivity is described by Eq. (7).[Citation19]Maxwell-Eucken Model
FIGURE 3 (A) Results of the average thermal conductivity of mango pulp; (B) Evaluation of freezing initiation temperature of mango pulp through the cooling curve; (C) Increase in mass fraction of ice of mango pulp during freezing; (D) Increased mass fraction of ice and the thermal conductivity of mango pulp during freezing.
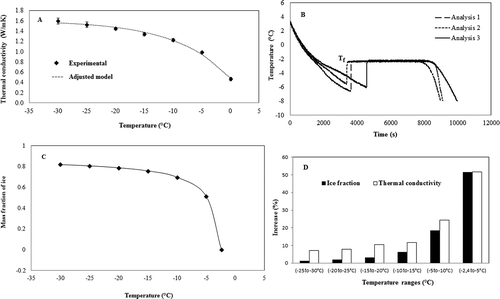
According to Resende and Silveira Júnior[Citation22] in a frozen state, a system is composed of two phases: the ice phase (dispersed phase) and the phase containing liquid water and solutes (continuous phase). In this case, it is assumed that ice is also dispersed in the second phase, consisting of unfrozen water and solutes.
Subsequently, the experimental thermal conductivity of the pulp was compared to values predicted by the serial, parallel, and Maxwell-Eucken mathematical models. A percentage error was calculated using Eq. (9). This error indicated which model best fitted the experimentally observed thermal conductivities.
Initial Freezing Temperature
The initial freezing temperature was evaluated directly from the cooling curve obtained from the temperature drop as a function of time during the freezing of the pulp. The experimental analyses were performed in triplicate. For data acquisition, a type T thermocouple AWG 30 (Omega Engineering Inc., Stamford, CT, USA) was used. The thermocouple was inserted in the center of the beaker with the sample, along with a thermometer for subsequent adjustment of temperature. For the cooling, an ultra-thermostatic bath (model 521/3DE; Nova Ética, Vargem Grande Paulista, Brazil) with digital temperature control was used. The temperature was recorded through a signal conditioning system (National Instruments Mod. SCXI; Budapest, Hungary). The temperature was recorded at 1 s intervals, using the LabView 8.5 software (National Instruments, Newbury, Ireland).[Citation23]
RESULTS AND DISCUSSION
Probe Calibration and Determination of the Correction Factor f
shows the experimental results of the thermal conductivity of the 10% sucrose solution as a function of temperature. The slopes of the straight lines obtained by the linear fit of the experimental data are also presented. The heating power applied per linear meter of resistance was 3.412 W/m. The factor was calculated using Eq. (1).
TABLE 1 Composition of frozen mango pulp from the Tabela Brasileira de Composição de Alimentos, TACO[Citation12]
TABLE 2 Experimental and theorical thermal conductivities of 10% sucrose solution and the correction factor
Experimental Thermal Conductivity Corrected
Three measurements were taken at each temperature to determine the corrected thermal conductivity of the pulps. The average of the correction factor , multiplied by the experimental thermal conductivity at each temperature was used. Mean values were plotted as a function of temperature, with the respective standard deviations, as shown in .
The parallel, perpendicular, and Maxwell-Eucken mathematical models were used to predict the thermal conductivity of the pulps and errors were evaluated. The Maxwell-Eucken model produced the most accurate adjustment for the mango pulp at −30 to 0ºC, yielding minimum and maximum errors of 10.8 and 17.8%, respectively. The parallel and perpendicular models yielded minimum and maximum errors of 45.8, 15.6, and 37.4, 5.2%, respectively.
The distribution of the points presented in is consistent with that shown by Heldman.[Citation24] The thermal conductivities of mango pulp increased with decreasing temperature, thus showing the interdependency between these two factors. There was a sharp increase in the thermal conductivity in the temperature range of 0 to –10°C because, in this range of temperatures, the variations in the ice fraction are too high. According to Renaud et al.,[Citation14] the fraction of frozen water greatly affects the thermal conductivity of the frozen material, as the conductivity of ice is four times higher than that of liquid water.
With the results obtained in , an exponential model was fitted to determine the specific thermal conductivity of mango pulp in the temperature range of 0 to –30°C as shown in Eq. (10). The correlation coefficient (R2) was 0.992.
Freezing Initiation Temperature
shows the dynamic freezing process of the mango pulp obtained by the method of the cooling curve. In all three analyses, an undercooling was observed, followed by a jump to a plateau at the initial freezing temperature. shows typical curve of frozen fruit pulp, which shows the ice nucleation and initial or equilibrium freezing point.[Citation23] The initial freezing point of mango pulp was found Tf = –2.4°C. The initial freezing temperature was further used to determine the ice fraction.
Fraction of Frozen Water
The frozen water fraction was determined according to Eqs. (4) and (5) at temperatures of –30, –25, –20, –15, –10, and –5°C, and the results are shown in . A continuous increase in mass was observed with decreasing temperature, with the largest increase observed for the range of 0 to –10°C. According to Pereira et al.,[Citation13] these two equations are presented as those featuring the lowest error in the prediction of the mass fraction of ice.
Comparison of Gains of Fraction of Frozen Water and Thermal Conductivity
shows the gain in percentage of ice fraction and conductivity for each variation of temperature drop to –5°C, starting is from 0 to –30°C, to better understand and evaluate the changes in thermal conductivity of mango pulp at low temperatures. The analysis of allows for the following conclusion: In the temperature range of 0 to –10°C, the percentage increase in thermal conductivity was accompanied by an increase in the mass fraction of ice, thus showing the significant contribution of water in frozen form to the conductivity of a food. This non-negligible contribution can be proven using the intrinsic conductivities. According to Ashrae,[Citation20] the contributions of the components: protein, ash, fiber, carbohydrates, fats, and water are 0.173, 0.323, 0.177, 0.194, 0.195, and 0.562 W/mK, respectively, at a temperature of –5°C. For ice at the same temperature, the conductivity is 2.253 W/mK.
CONCLUSION
The results relative to the fraction of frozen water showed large increases from the onset temperature of freezing to about –10°C. In this temperature range, a sharp increase in thermal conductivity of the pulp was also observed, pointing at the direct dependence between the two properties. The models used to determine the theoretical thermal conductivities were not completely adjusted to the values obtained experimentally. Therefore, a specific exponential model was fitted to determine the thermal conductivity of mango pulp in the temperature range of 0 to –30°C. The equation allows for the direct calculation of this property, using only the knowledge of the history of the temperatures recorded during processing, and thereby enabling the acquisition of data necessary for planning projects involving low temperatures.
FUNDING
The authors wish to thank the Fundação de Amparo à Pesquisa do Estado de Minas Gerais (FAPEMIG—Brazil), Conselho Nacional de Desenvolvimento Científico e Tecnológico (CNPq—Brazil), and Coordenação de Aperfeiçoamento de Pessoal de Nível Superior (CAPES—Brazil) for financial support for this research.
NOMENCLATURE
Table
Subscripts
= | Continuous | |
= | Disperse | |
exp | = | Experimental |
= | Initial freezing | |
= | Initial freezing of the sample | |
= | Initial freezing of water | |
= | Pure component | |
= | Maxwell-Eucken | |
= | Number of components | |
= | Initial | |
= | Parallel | |
= | Solids | |
= | Series | |
the | = | Theorical |
= | Water | |
= | Unfrozen water |
Additional information
Funding
REFERENCES
- Brunini, M.A.; Durigan, J.F.; Oliveira, A.L. Quality of ‘Tommy-Atkins’ mango pulp frozen and stored at –18 °C. Revista Brasileira de Fruticultura 2002, 24(3), 651–653.
- Franco, G. Tabela de Composição Química Dos Alimentos, 9ª Ed; Rio de Janeiro, RJ: Editora Livraria Atheneu, 1999, 307 p.
- Bleinroth, E.W. Caracterização de Variedades de Manga Para Industrialização. In Instruções Técnicas Campinas: Instituto de Tecnologia de Alimentos, Campinas, SP, 1976; 78 p.
- Moura, S.C.S.R.; França, V.C.L.; Leal, A.M.C.B. Propriedades Termofísicas de Soluções Modelo Similares A Sucos: Parte II. Ciência e Tecnologia de Alimentos 2005, 25(3), 454–459.
- Sweat, V.E. Thermal Properties of Foods. In Engineering Properties of Foods; Rao, M.A.; Rizvi, S.S.H., Eds.; Marcel Dekker: New York, NY, 1986; 49–87 pp.
- Mohsenin, N.N. Thermal Properties of Foods and Agricultural Materials; Gordon and Breach: London, 1980; 407 p.
- Park, K.J.; Alonso, L.F.; Nunes, A.S. Determinação Experimental da Condutividade e Difusividade de Grãos em Regime Permanente. Ciência e Tecnologia de Alimentos 1999, 19(2), 264–269.
- Carson, J.K. Review of Effective Thermal Conductivity Models for Food. International Journal of Refrigeration 2006, 29(6), 958–967.
- Ampawan Tansakul, A.; Saengrayup, H.K.R.; Sura, P. Thermophysical Properties of Papaya Puree. International Journal of Food Properties 2012, 15(5), 1086–1100.
- Nguyen, L.T.; Balasubramaniam, V.M.; Sastry, S.K. Determination of In-Situ Thermal Conductivity, Thermal Diffusivity, Volumetric Specific Heat, and Isobaric Specific Heat of Selected Foods under Pressure. International Journal of Food Properties 2012, 15(1), 169–187.
- Delgado, A.E.; Gallo, A.; Piante, D.; Rubiolo, A. Thermal Conductivity of Unfrozen and Frozen Strawberry and Spinach. Journal of Food Engineering 1997, 31(2), 137–146.
- Ministério da Saúde. Tabela Brasileira de Composição de Alimentos. 4ª Ed., Ministério da Saúde, Editora FODEPAL NEPA/UNICAMP. Campinas: SP, 2011.
- Pereira, C.G.; Resende, J.V.; Pereira, G.G.; Giarola, T.M.O.; Prado, M.E.T. Thermal Conductivity Measurements and Predictive Models for Frozen Guava and Passion Fruit Pulps. International Journal of Food Properties 2013, 16(4), 778–789.
- Renaud, T.; Briery, P.; Andrieu, J.; Laurent, M. Thermal Properties of Food Materials in the Frozen State. Journal of Food Engineering 1992, 15(2), 83–97.
- Azoubel, P.M.; Cipriani, D.C.; El-Aouar, A.A.; Antonio, G.C.; Murr, F.E.X. Effect of Concentration on the Physical Properties of Cashew Juice. Journal of Food Engineering 2005, 66(4), 413–417.
- Jury, V.; Monteau, J.-Y.; Comiti, J.; Le-Bail, A. Determination and Prediction of Thermal Conductivity of Frozen Part Baked Bread During Thawing and Baking. Food Research International 2007, 40(7), 874–882.
- Hamdami, N.; Monteau, J.-Y.; Le Bail, A. Thermophysical Properties Evolution of French Partly Baked Bread During Freezing. Food Research International 2004, 37(7), 703–713.
- Brock, J.; Nogueira, M.R.; Zakrzevski, C.; Corazza, F.C.; Corazza, M.L.; Oliveira, J.V. Experimental measurements of viscosity and thermal conductivity of vegetable oils. Ciência e Tecnologia de Alimentos 2008, 28(3), 564–570.
- Miyawaki, O.; Pongsawatmanit, R. Mathematical Analysis of the Effective Thermal Conductivity of Food Materials in the Frozen State. Bioscience, Biotechnology Biochemistry 1994, 58(7), 1222–1225.
- Ashrae. Handbook of Refrigeration; ASHRAE: Atlanta, GA, 2002.
- Heldman, D.R.; Lund, D.B. Handbook of Food Engineering; Taylor & Francis Group: New York, NY, 1992; 1023 p.
- Resende, J.V.; Silveira Júnior, V. Medidas da Condutividade Térmica Efetiva de Modelos de Polpas de Frutas No Estado Congelado. Ciência e Tecnologia de Alimentos 2002, 22(2), 177–183.
- Rahman, M.S.; Guizani, N.; Al-Khaseibi, M.; Al-Hinai, S.; Al-Maskri, S.S.; Al-Hamhami, K. Analysis of Cooling Curve to Determine the End Point of Freezing. Food Hydrocolloids 2002, 16(6), 653–659.
- Heldman, D.R. Food Properties During Freezing. Food Technology 1982, 36(2), 92–96.