Abstract
Emulsifying properties of two commercial food proteins (plasma protein or egg albumen) conjugated with two sugars (glucose or lactose) by spray-drying or lyophylization were studied. Changes in protein structure were determined by sodium dodecyl sulfate-polyacrylamide gel electrophoresis, sulfhydryl group, Fourier transform infrared spectroscopy and differential scanning calorimetry, emulsion properties (emulsion capacity and stability, emulsion work, and emulsifying activity index). Protein conjugation increased proteins molecular weight and decreased sulfhydryl groups, coinciding with lower denaturation enthalpies, corroborating structural changes by conjugation with glucose or lactose by either spray-drying or freeze-drying. Glucose conjugation of the proteins employed at the experimental conditions resulted in structural changes that emulsify more oil with no detrimental effect on emulsion superficial area formation. Although freeze-drying conjugation improved emulsifying properties, spray-drying also enhanced these properties, as compared to non-conjugated proteins. Spray-drying was a faster way to conjugate food proteins via Maillard reaction with improved emulsifying properties.
INTRODUCTION
Physicochemical properties of proteins can be modified enzymatically or chemically in order to improve their functional properties. Enzymatic modification requires choosing the correct proteolityc enzyme, environmental conditions, and degree of hydrolysis in order to enhance functional properties of proteins. In contrast, chemical modification involves protein side chain reaction to reagents designed to preserve or alter electrostatic charge and to increase hydrophobicity (e.g., acylation, succinylation, derivatization or glycosylation, between others).[Citation1,Citation2] Chemical modification like derivatizaton of whey proteins enhanced emulsifying properties, expedite the development of applications with the novel dairy ingredients.[Citation3] Enzymatic modification also enhances emulsifying properties. For example, bovine globin hydrolysis improved emulsion stability in a wide range of pH.[Citation4] In the same manner, transglutaminase modified casein emulsifying activity index and emulsifying stability index were about 12 and 20% higher than that of original casein.[Citation5]
Glycosylation, otherwise known as Maillard reaction, endows food proteins with improved functional properties occurring under mild and safe conditions and requires no extraneous chemicals, making this method promising for protein modification in the food industry.[Citation6] Glycation had been employed to improve the stability and emulsifying properties of proteins.[Citation7] Glycosylation takes place in foods when a proteins side chains suffer modifications in the presence of reducing sugars during thermal processes, resulting in the formation of N-glycosides as well as the numerous consecutive reactions, classed under the term of Maillard reaction.[Citation8] These proteins–sugars conjugates combine the emulsifying capacity of proteins with solvating characteristics of sugars,[Citation9,Citation10] enhancing emulsifying properties of proteins.[Citation11–Citation16]
The common way to induce protein–sugars conjugated products via Maillard reaction is by lyophilization and conjugation of protein–sugars mixtures at relatively high relative humidity (RH) and temperature. There is not an established range of temperatures, times, and RH conditions after lyophilization (despite components concentrations and pH conditions) to conjugate protein–sugars mixtures. For example, Aoki et al.[Citation17,Citation18] conjugated ovalbumin and glucose and other sugars at 50°C at 65% RH during 12 to 72 h. In same manner, Du et al.[Citation19] employed 60°C and 79% RH during 24 h to conjugate rice dreg glutelin and κ-carrageenan. In another study, lysozyme and caseins were conjugated with dextran during 48 or 78 h at RH = 79%.[Citation20] Sato et al.[Citation21] incubated at 50°C and 35% RH for 120 h preparations of carp myofibrillar protein and alginate. It seems that the longer the time the higher degree of sugars conjugated to proteins. As an alternative, spray-drying is a technique to reduce or eliminate water from solutions, involving higher temperatures and short exposure times. Spray-drying of protein–sugars systems result in Maillard compounds formation, where some chemical reactions may be exploited for desirable results, like colored powders for food coloration.[Citation22] Formation of Maillard compounds (volatile compounds identified as a series of furans, pyrroles, and pyrazines) had been reported in glucose-glycine mixtures,[Citation23] as well as in whey protein-rich powders (formation of hydroxymethylfurfural and covalently bound protein with free lactose).[Citation24] In the same manner, Maillard reaction products produced during spray-drying by whey proteins-maltodextrin mixtures had been employed for conjugated linoleic acid microencapsulation.[Citation25]
In this view, since it also has been reported that spray-drying process also forms protein–sugar conjugates, this can be considered an option to obtain proteins–sugars conjugates as new food ingredients with improved functional properties, in a faster way as compared to the conjugation after lyophilize at high RH. The aim of this work was to analyze the emulsifying properties of food proteins conjugated with glucose or lactose by to different ways: spray-drying or freeze-dry (lyophilization).
MATERIALS AND METHODS
Samples Preparation
Commercial food grade proteins were employed. Plasma protein (Porcine Plasma Powder U70®, Interaliment S.A., México City) and egg albumen protein (Egg Albumen Campeon®, Alimentos Deshidratados S.A., México City). Glucose (J.T. Baker, Xalostoc, México) or D-lactose (HYCEL de México, Zapopan) was employed to conjugate with proteins by two different methods.
The protein and sugars solution for conjugation was prepared adapting the Saeki and Inoue[Citation26] methodology. Proteins were solubilized in distilled water (250 mL), adjusting protein content to 6 mg/mL, determining soluble protein by using biuret reaction.[Citation27] Glucose or lactose was added to protein solutions adjusting concentration to 0.3 M. For spray-dry conjugation, protein–sugar solutions were spray-dried in a Büichi 190 Mini Spray Dryer (Büichi (Büichi) Labortechnik AG, Flawil), at 130°C inlet temperature, 97°C outlet temperature, and 1.50 L/h airflow rate. For the freeze-dry conjugation, protein–sugar mixtures were frozen at 78°C 24 h before being freeze-dried in a Labconco FreeZone Freeze Dry System (Labconco Corporation, Kansas City). Lyophilized protein–sugar powder was incubated at 43°C and 65% RH for 24 h in an EH201 oven (Aparatos de Laboratorio, México). Protein-conjugated powders of both conjugation methods were kept in closed dark flasks until analysis.
Structural Characteristics of the Conjugates
Molecular weight by gel electrophoresis
To determine the effect of glucose or lactose conjugation by spray-dry or freeze-dry, food proteins molecular weight was determined in sodium dodecyl sulfate-polyacrylamide gel electrophoresis (SDS-PAGE), under denaturing conditions,[Citation28] in a Mini Protean II Slab cell equipment (Bio Rad, Richmond, California, USA). Acrylamide concentrations were 4 and 7.5% (w/v) for resolving and stacking gels, respectively. Molecular weight markers (Bio-Rad No. 161-0303) were run simultaneously to determinate molecular weight. Gels were stained with Coomassie brilliant blue R-250 (40% methanol-10% acetic acid solution, v/v) and analyzed in a Gel Doc 2000 (Bio-Rad, Richmond, USA) with the Quality One Bio-Rad software version 4.2.1.
Sulfhydryl groups
Quantification of sulfhydryl groups were determined employing Ellman’s reagent based on the molar absorptivity of 5, 5’-dithiobis (2-nitrobenzoic) acid (TNB; Ellman’s Reagent #22582, PIERCE Biotechnology, Rockford. USA). A 250 μL aliquot of conjugated protein samples was mixed with 2.5 mL of Reaction Buffer (0.1M sodium phosphate, pH 8.0, containing 1 mM EDTA) and 50 μL of Ellman’s Reagent Solution. Absorbance at 412 nm was reading after 15 min and the amount and concentration of sulfhydryls was calculated according to:
where, Abs412 nm is the absorbance at 412 nm, D is the dilution factor (final volume/aliquot ratio), and E is the TNB molar absorptivity (14,150 M–1cm–1).
Differential scanning calorimetry (DSC)
Protein-conjugated samples were analyzed in a differential scanning calorimeter TA Instruments DSC model 2010 (TA Instruments, New Castle, USA). Samples (ca. 10 mg) were placed in hermetic aluminum containers, sealed and placed in the heating cell of the calorimeter. Samples were heated (air purge) from 20 to 200°C at 10°C/min, calculating thermal transition temperature (onset and maximum temperature peak, Td) and thermal denaturation enthalpy (integral under the maximum peak area, ΔH).
Fourier transform infrared (FTIR) analysis
Infrared spectra were performed in a Thermo Nicolet Spectrophotometer model Avatar 300 FTIR (Thermo Fisher Scientific Inc., Waltham. USA) with Fourier transform equipped with a KBr deuterated triglycine sulfate detector. Dry powder of each sample was weight (ca. 5 mg) and mixed with 0.5 g of KBr (Sigma-Aldrich, St. Louis, USA) in an agate mortar. Pure KBr was employed as base line and samples were run with 64 sweeps in Transmittance mode with length wave intervals of 4000 to 600 cm–1.
Emulsifying Properties of Conjugates
The emulsifying capacity reported by Kato et al.[Citation29] was adapted. In an acrylic device, 15 mL of protein solution (6–10 mg/mL) plus 10 mL of corn oil (Alimentos Capullo, México City) were emulsifying for one minute before adding more corn oil at a constant rate (10 mL/min) with a burette, employing an Oster homogenizer to emulsifying. Electric resistance or conductivity of the protein solution was monitored during the test with a Multimeter MUL600 (Electrónica Steren, México City) until phase inversion, indicated by the abrupt interruption in conductivity. Emulsion capacity was reported as the total emulsified mL of oil per gram of protein before phase inversion. The conductivity-time graphs were obtained employing the MUL600 Interface Program version 2.0, and exported to Origin Software ver. 7.0 (OriginLab Corporation, Northampton), to calculate the area under the curve as emulsion work (Ω/s)[Citation30] and emulsion stability,[Citation29] according to:
where, Cs is the conductivity of the protein emulsion after one minute (before add more oil), Ce is the conductivity of emulsion before slope change, and Δt/Δc is the reciprocal of initial slope of the conductivity curve during the first minutes when oil was added.
Emulsion activity index (EAI; area of interface stabilized per unit weight of protein) was determined according to the Pierce and Kinsella[Citation31] methodology. To 6 mL of protein solution (6–10 mg/mL) 2 mL of corn oil (Alimentos Capullo, México City) were added and emulsified during 1 min employing an Oster homogenizer to form the emulsion. After 1 min, an aliquot of 0.5 mL was dispersed in 4.5 mL of sodium dodecyl sulphate solution (1% w/v) to read the absorbance at 500 nm in order to calculate EAI according to:
where, ϕ is the dispersed phase fraction (0.25), and C is the weight of protein per unit volume of aqueous phase before the emulsion is formed.
Experimental Design and Data Analysis
A complete factorial design was employed to determine the effect of the protein type, type of sugar, and conjugation method on functional properties of conjugated proteins. The proposed model was:
where, yijk represents the functional properties for the ith protein type (egg albumen protein or plasma protein), the jth type of sugar (glucose or lactose), the kth conjugation method (spray-drying or freeze-dry); μ is the overall mean; αi, βj, and γk are the main effects of protein, sugar and conjugation method, respectively; ∈ijk is the residual or error terms assumed to be normally distributed with zero mean and variance σ2.[Citation32] Results were analyzed with the PROC ANOVA procedure in SAS Software version 8.0 (SAS System, Cary, USA). Significant differences between means were determined by the Duncan means test.
RESULTS AND DISCUSSION
Structural Characteristics
Molecular weight by SDS-PAGE
Conjugation increased the molecular weight of the two main egg white albumen protein fractions (approx. at 45 kDa and 97 kDa, , line 2). It seems that conjugation with glucose resulted in higher molecular weight, irrespectively of the conjugation method (, lines 3–6). Lower molecular weight was observed in conjugated protein fractions with lactose obtained by freeze-drying (, line 6). For plasma proteins, the effect of sugars and conjugation method seems to be more marked for the three main protein fractions that can be appreciated at around 66, 98, and 116 kDa (, line 2). When glucose was employed (, lines 3 and 4), the molecular weight was higher than for lactose samples (, lines 5 and 6). The conjugation by spray-drying resulted in lower molecular weight fractions as compared to freeze-drying conjugation (, lines 3 and 5).
FIGURE 1 SDS-PAGE electrophoretogram for A:(a) egg albumen protein and B:(b) plasma protein. Line labels: (1) molecular weight markers; (2) control non-conjugated; (3) +glucose conjugated by spray-drying; (4) +glucose conjugated by freeze-drying; (5) +lactose conjugated by spray-drying; (6) +lactose conjugated by freeze-drying.
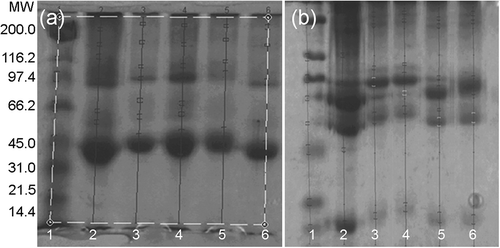
In Maillard reaction, regarding to reducing sugars, hexoses are more reactive than disaccharides.[Citation33,Citation34] Modification degree was in direct relation with the sugar size, where the shorter the carbonic chain of the sugar is, the more open chain forms exist and the more reactive is the sugar with the amino groups of proteins.[Citation33] The formation of protein-sugar complex via Maillard reaction has been demonstrated with SDS-PAGE.[Citation5,Citation9,Citation15,Citation16,Citation35] These results suggest that higher molecular weight conjugates were obtained in glucose and freeze-drying samples.
Sulfhydryl groups
Proteins conjugation with sugars at the employed experimental conditions affected the sulfhydryl group content. Plasma proteins presented significantly (p < 0.05) higher sulfhydryl groups content than egg white proteins. Lactose conjugation resulted in significantly (p < 0.05) lower sulfhydryl groups content for both proteins. Conjugation by spray-drying or freeze-drying significantly (p < 0.05) decreased the sulfhydryl groups content as compared to non-conjugated samples (). These changes can be attributed to sulfhydryl group oxidation and disulfide bond formation as a result of protein polymerization due to protein unfolding through the Maillard reaction.[Citation14,Citation36]
TABLE 1 Sulfhydryl groups (mM) content of conjugated food proteins with dextrose or lactose by freeze-drying or spray-drying
DSC
Thermal properties of conjugated proteins were affected by sugar and conjugation method. For the denaturation temperature, egg albumen protein presented significantly (p < 0.05) higher values than plasma protein. Significantly higher (p < 0.05) denaturation temperature (Td) values were obtained when proteins were conjugated with lactose. Protein–sugar conjugation by spray-drying resulted in significantly (p < 0.05) higher denaturation temperature for both proteins, followed by freeze-drying conjugated samples (). Denaturation enthalpy (ΔH) of plasma protein was significantly (p < 0.05) higher than egg albumen protein. Lactose containing samples presented significantly (p < 0.05) lower enthalpy values than glucose containing samples. Spray-drying conjugation increased as well significantly (p < 0.05) the denaturation enthalpy of protein conjugates (). Glycosylation of proteins results in higher thermal stability,[Citation13,Citation32,Citation37] reflected in an increase transition temperature[Citation12,Citation15] and a decrease in ΔH protein conjugates by the crosslinking between proteins and sugars.[Citation15] Lower amount of sulfhydryl groups coincide with lower denaturation enthalpies, corroborating structural changes (besides SDS-PAGE results) in proteins by conjugation with glucose or lactose by either spray-drying or freeze-dry.
FTIR spectra
For egg white albumen conjugated samples, changes in wavelengths 3500–3000 and 2050–2030 cm–1 (sulfur groups) were associated to their hydrogen bond sensibility associated to sugars conjugation. In plasma protein samples, a similar behavior was observed, with main changes at protein band (3500–3000 cm–1) and 1700–1100 cm–1 band (COO- region, related to sugar presence; ). Changes in protein conformation can be determined by FTIR.[Citation38] Changes in protein structure by the employed sugar to conjugate proteins were related to some specific protein and sugar infrared (IR) spectra regions. In conjugated protein samples, saccharides band regions (around 1180–953 cm–1) were stronger presenting a series of overlapping peaks as result of the vibration modes of C–C and C–O stretching and C–H bonds bending.[Citation35] In the same manner, conjugated proteins had a more intense absorption band at 3700–3200 cm–1 caused by –OH stretching vibration, and decreasing the intensity of the bands at 1660–1400 and 1100–1000 cm–1, and loss of functional groups like NH2 provokes remarkable absorption around 1654 and 1528 cm–1.[Citation19]
Conjugates Emulsifying Properties
In general, plasma protein presented significantly (p < 0.05) higher emulsifying properties values than egg albumen protein. The protein-glucose conjugates resulted in significantly (p < 0.05) higher values, with no difference between lactose-conjugated and the control samples. Emulsifying capacity of freeze-drying conjugated samples was significantly (p < 0.05) higher. Control samples obtained the lower emulsifying capacity values (). Similar tendency was observed for emulsion stability, where glucose conjugation increased significantly (p < 0.05) the emulsion stability in comparison with lactose and control samples. Freeze-drying treatment resulted in significantly (p < 0.05) higher emulsion stability values than spray-drying treatment. Control treatment obtained the lower values (). The emulsion work increased significantly (p < 0.05) when glucose was employed to conjugate proteins, followed by lactose samples. In the same way, emulsion work was significantly (p < 0.05) higher for freeze-drying conjugated samples, followed by spray-drying treatment. Lower emulsion work values were observed in Control samples ().
TABLE 2 Denaturation temperature (Td) and enthalpy (∆H) of conjugated food proteins with dextrose or lactose by freeze-drying or spray-drying
TABLE 3 Emulsifying properties of conjugated food proteins with dextrose or lactose by freeze-drying or spray-drying
Emulsifying activity index of conjugated proteins significantly (p < 0.05) decreased in lactose samples, with no difference between glucose and non-conjugated samples. Conjugation decreased significantly (p < 0.05) the emulsifying activity index, with lower values for spray-drying treatments ().
The improved emulsifying properties of conjugated proteins can be attributed to a more stable structure and to the formation of an emulsion droplet network due to the presence of adsorbed polysaccharide molecules in the inter-droplet region,[Citation12,Citation14–Citation16] and the increase of the amphiphilic character caused by the sugar attachment.[Citation13] Hence, protein conjugation, by spray-drying or freeze-dry, improved the emulsifying capacity of proteins as compared to non-conjugated proteins. Glucose enhanced the ability of proteins to emulsifying oil, probably in relation to higher molecular weight, higher content of sulfhydryl groups and a more thermally stable structure of these conjugates. A more stable emulsion formation in glucose freeze-drying conjugated samples was observed since the rate of coalescence of oil droplets, reflected in the initial slope of the conductivity curves during emulsion, decreased during emulsion formation.[Citation29] In the same way, the resulting conjugated presented strong electrostatic interactions during oil emulsion, increasing the energy required to form the emulsion.[Citation30] Lactose conjugation decreased proteins capacity to emulsifying more oil and reduced their emulsifying area formation as well. The main differences between both conjugation methods employed are related to water remotion, changing chemical structure that affected their behavior as food ingredients. For example, spray-dried lactose/protein mixtures are obtained from rapid removal of water, which allows some shrinkage and possibly intermolecular interaction and hydrogen-bonding. On the contrary, in freeze-dried lactose/protein mixtures, water molecules are removed from freeze-concentrated glassy solids, presenting higher amount of hydrogen bonding sites available for water molecule in sorption than spray-dried materials.[Citation39]
CONCLUSIONS
The improvement in the emulsifying capacity seems to be related to the decrease in –SH groups, as a result of the molecular weight increase of certain proteins fractions, with the concomitant formation of thermally stable proteins conjugated structures. Glucose conjugation of the proteins employed at the experimental conditions resulted in structural changes that emulsify more oil with no detrimental effect on emulsion superficial area formation. Although freeze-drying conjugation enhanced emulsifying properties, spray-drying improved these properties as compared to non-conjugated proteins, resulting in a faster way to conjugate food proteins via Maillard reaction. The obtained results will allow developing new food ingredients since the spray-drying conjugation showed to be a faster and viable way to obtain sugar-conjugated food proteins.
FUNDING
Sonia Hernández-García thank CONACyT (México) for the grant for her M.Sc. studies.
ACKNOWLEDGMENT
A special thanks to Miguel Marques-Robles (CINVESTAV-IPN) for his assistance on the DSC tests.
Additional information
Funding
REFERENCES
- Means, G.E.; Feeney, R.E. Chemical Modifications of Proteins: History and Applications. Bioconjugate Chemistry 1990, 1, 1–12.
- Panyam, D.; Kilara, A. Enhancing the Functionality of Food Proteins by Enzymatic Modification. Trends in Food Science and Technology 1996, 7, 120–125.
- Firebaugh, J.D.; Daubert, C.R. Emulsifying and Foaming Properties of a Derivatized Whey Protein Ingredient. International Journal of Food Properties 2005, 8, 243–253.
- Capobiango, M.; Schaper Bizzotto, C.; Rolim Biasutti, E.A.; Pinto Coelho Silvestre, M. Action of Pepsin on Emulsifying Properties of Globin. International Journal of Food Properties 2006, 9, 357–364.
- Jiang, S.-J.; Zhao, X.-H. Cross-Linking and Glucosamine Conjugation of Casein by Transglutaminase and the Emulsifying Property and Digestibility in Vitro of the Modified Product. Journal of Food Properties 2012, 15, 1286–1299.
- Liu, J.; Ru, Q.; Ding, Y. Glycation a Promising Method for Food Protein Modification: Physicochemical Properties and Structure, A Review. Food Research International 2012, 49, 170–183.
- Lv, L.; Chi, Y.; Chen, C.; Xu, W. Structural and Functional Properties of Ovalbumin Glycated by Dry-Heating in the Presence of Maltodextrin. International Journal of Food Properties 2015, 18, 1326–1333.
- Belitz, H.-D.; Grosch, W.; Schieberle, P. Food Chemistry, 4th Ed.; Springer-Verlag: Berlin, 2009.
- Diftis, N.; Kiosseoglou, V. Improvement of Emulsifying Properties of Soybean Protein Isolate by Conjugation with Carboxymethylcellulose. Food Chemistry 2003, 81, 1–6.
- Choi, S.J.; Kim, H.J.; Park, K.H.; Moon, T.W. Molecular Characteristics of Ovalbumin–Dextran Conjugates Formed Through the Maillard Reaction. Food Chemistry 2005, 92, 93–99.
- Kato, A. Preparation and Functional Properties of Protein-Polysaccharide Conjugates. In Surface Activity of Proteins; Magdassi, S.; Ed.; Marcel Dekker: New York, NY, 1996; 115–129 pp.
- Nacka, F.; Chobert, J.-M.; Burova, T.; Leonil, J.; Haertle, T. Induction of New Physicochemical and Functional Properties by the Glycosylation of Whey Proteins. Journal of Protein Chemistry 1998, 17, 495–503.
- Campbell, L.; Raikos, V.; Euston, R.S. Modification of Functional Properties of Egg-White Proteins. Nahrung/Food 2003, 47, 369–376.
- Diftis, N.; Kiosseoglou, V. Physicochemical Properties of Dry-Heated Soy Protein Isolate–Dextran Mixtures. Food Chemistry 2006, 96, 228–233.
- Liu, Y.; Zhao, G.; Zhao, M.; Ren, J.; Yang, B. Improvement of Functional Properties of Peanut Protein Isolate by Conjugation with Dextran Through Maillard Reaction. Food Chemistry 2012, 131, 901–906.
- Kasrana, M.; Cui, S.W.; Goff, H.D. Emulsifying Properties of Soy Whey Protein Isolate-Fenugreek Gum Conjugates in Oil-In-Water Emulsion Model System. Food Hydrocolloids 2013, 30, 691–697.
- Aoki, T.; Hiidome, Y.; Kitahata, K.; Sugimoto, Y.; Ibrahim, H.R.; Kato, Y. Improvement of Heat Stability and Emulsifying Activity of Ovalbumin by Conjugation with Glucuronic Acid Through the Maillard Reaction. Food Research International 1999, 32, 129–133.
- Aoki, T.; Hiidome, Y.; Sugimoto, Y.; Ibrahim, H.R.; Kato, Y. Modification of Ovalbumin with Oligogalacturonic Acids Through the Maillard Reaction. Food Research International 2001, 34, 127–132.
- Du,Y.; Shi, S.; Jiang, Y.; Xiong, H.; Woo, M.W.; Zhao, Q.; Bai, C.; Zhou, Q.; Sun, W. Physicochemical Properties and Emulsion Stabilization of Rice Dreg Glutelin Conjugated with κ-carrageenan Through Maillard Reaction. Journal of the Science of Food and Agriculture 2013, 93, 125–133.
- Aminlari, M.; Ramezani, R.; Jadidi, F. Effect of Maillard-Based Conjugation with Dextran on the Functional Properties of Lysozyme and Casein. Journal of the Science of Food and Agriculture 2005, 85, 2617–2624.
- Sato, R.; Katayama, S.; Sawabe, T.; Saeki, H. Stability and Emulsion-Forming Ability of Water-Soluble Fish Myofibrillar Protein Prepared by Conjugation with Alginate Oligosaccharide. Journal of Agriculture and Food Chemistry 2003, 51, 4376–4381.
- Dumoulin, E.; Bimbenet, J.J. Spray Drying and Quality Changes. In The Properties of Water in Foods ISOPOW 6; Reid, D.S.; Ed.; Blackie Academic & Professional: London, 1998; 209–232 pp.
- Tomlinson, A.J.; Mlotkiewicz, J.A.; Lewis, I.A.S. Investigation of the Compounds Produced by Spray-Drying and Aqueous Solution of Glucose and Glycine. Food Chemistry 1993, 48, 373–379.
- Bernard, C.; Broyart, B.; Vasseur, J.; Granda, P.; Relkin, P. Enhancement of Protein Structure-Forming Properties in Liquid Foams by Spray Drying. Dairy Science and Technology 2008, 88, 65–80.
- Choi, K.O.; Ryu, J.; Kwak, H.S.; Ko, S. Spray-Dried Conjugated Linoleic Acid Encapsulated with Maillard Reaction Products of Whey Proteins and Maltodextrin. Food Science and Biotechnology 2010, 19, 957–965.
- Saeki, H.; Inoue, K. Improved Solubility of Carp Myofibrillar Proteins in Low Ionic Strength Medium by Glycosylation. Journal of Agriculture and Food Chemistry 1997, 45, 3419–3422.
- Gornall, A.G.; Bardwill, C.J.; David, M.M. Determination of Serum Proteins by Means of the Biuret Reaction. Journal of Biological Chemistry 1949, 177, 751–766.
- Laemmli, K. Cleavage of Structural Proteins During the Assembly of the Head of Bacteriophage T4. Nature 1940, 227, 680–685.
- Kato, A.; Fujishige, T.; Matsumodi, N.; Kobayashi, K. Determination of Emulsifying Properties Some Proteins by Conductivity Measurement. Journal of Food Science 1985, 50, 56–58, 62.
- Mena-Casanova, E.; Totosaus, A. Improvement of Emulsifying Properties of Milk Proteins with κ Or λ Carrageenan: Effect of pH and Ionic Strength. International Journal of Food Science and Technology 2011, 46, 535–541.
- Pearce, K.N.; Kinsella, J.E. Emulsifying Properties of Proteins: Evaluation of a Turbidimetric Technique. Journal of Agriculture and Food Chemistry 1978, 26, 716–723.
- Der, G.; Everitt, B.S. Handbook of Statistical Analyses Using SAS; Chapman & Hall/CRC: Boca Raton, 2002; 101–116 pp.
- Chevalier, F.; Chobert, J.-M.; Popineau, Y.; Nicolas, M.G.; Haertlé, T. Improvement of Functional Properties of β-Lactoglobulin Glycated Through the Maillard Reaction Is Related to the Nature of the Sugar. International Dairy Journal 2001, 11, 145–152.
- Naranjo, G.B.; Pereyra-Gonzales, A.S.; Leiva, G.E.; Malec, L.S. The Kinetics of Maillard Reaction in Lactose-Hydrolysed Milk Powder and Related Systems Containing Carbohydrate Mixtures. Food Chemistry 2013, 141, 3790–3795.
- Gu, F.-L.; Kim, J.M.; Abbas, S.; Zhang, X.-M.; Xia, S.-Q.; Chen, Z.-X. Structure and Antioxidant Activity of High Molecular Weight Maillard Reaction Products from Casein–Glucose. Food Chemistry 2010, 120, 505–511.
- Handa, A.; Kuroda, N. Functional Improvements in Dried Egg White Through the Maillard Reaction. Journal of Agriculture and Food Chemistry 1999, 47, 1845–1850.
- Álvarez, C.; García, V.; Rendueles, M.; Díaz, M. Functional Properties of Isolated Porcine Blood Proteins Modified by Maillard’s Reaction. Food Hydrocolloids 2012, 28, 267–274.
- Long, G.; Ji, Y.; Pan, H.; Sun, Z.; Li, Y.; Qin, G. Characterization of Thermal Denaturation Structure and Morphology of Soy Glycinin by FTIR and SEM. International Journal of Food Properties 2015, 18, 763–774.
- Haque, M.K.; Roos, Y.H. Differences in the Physical State and Thermal Behavior of Spray-Dried and Freeze-Dried Lactose and Lactose/Protein Mixtures. Innovative Food Science and Emerging Technologies 2006, 7, 62–73.