Abstract
Food-grade phycocyanin was obtained from Spirulina platensis cultured in seawater-based medium and purified by ammonium sulfate precipitation. The stability of phycocyanin under different conditions, including different pH, temperature, light, and edible stabilizing agents, was systematically investigated by spectroscopy methods. The optimum pH range for phycocyanin was found to be 5.0–6.0. Phycocyanin was kept stable at temperatures up to 45ºC over short time periods (i.e., no significant changes were observed in the relative concentration of phycocyanin, CR). In contrast, incubation at a relatively high temperature resulted in a decrease in the CR and half-life in a temperature-dependent manner. Constant exposure to light at 100 μmol m–2 s–1 for 36 h, decreased the CR value of phycocyanin (pH5.0) to 78.4%. Sodium chloride was an effective stabilizing agent for phycocyanin, and its efficacy increased in a concentration-dependent manner for all concentration ranges assessed in this study. Moreover, phycocyanin exhibited concentration-dependent antioxidant activities in 2,2′-azino-bis-(3-ethylbenzthiazoline-6-sulfonic acid) and α,α-Diphenyl-β-pricrylhydrazyl assays. Taken together, our results suggest that the optimal conditions for preserving the stability of food-grade phycocyanin isolated from S. platensis are a pH of 5.0–6.0, low temperature, darkness, and the addition of edible stabilizing agents.
INTRODUCTION
Spirulina platensis, namely Arthrospira platensis, is a non-toxic cyanobacterium widely distributed in tropical and subtropical lakes characterized by a high pH and high concentrations of carbonate and bicarbonate.[Citation1] S. platensis has long been used as a food source and is considered to be safe for human consumption.[Citation2] In addition to its relatively high protein content, S. platensis also contains vitamins, minerals, unsaturated fatty acid, carotenoids, and other nutrients.[Citation2] Therefore, S. platensis is considered a balanced natural food and is widely used as dietary supplement. Various processes have been developed to reduce the production costs and improve the quality of Spirulina for commercial purposes.[Citation3] A large-scale Spirulina cultivation technique in seawater medium was established by the South China Sea Institute of Oceanology, Chinese Academy of Sciences in the early 1990s.[Citation4] Cultivation in seawater medium not only improved the nutritional composition of S. platensis, but also effectively prevented such problems, as the accumulation of excess levels of heavy metals, which is a common occurrence in fresh water culturing.[Citation5] Phycocyanin, the major phycobiliprotein of S. platensis, is an essential functional component. It has recently drawn much attention due to its excellent nutritional value, fluorescence specific-properties, and physiological functions.
Phycocyanin is often used as nutritional supplement, and has great potential benefits for human nutrition and health, as it contains all the essential amino acids.[Citation6] As a water-soluble natural pigment, it is also widely used as a food coloring agent, cosmetic additives, fluorescent reagent for clinical diagnosis, and immunochemistry.[Citation7–Citation9] In addition, phycocyanin has significant antioxidative, anti-inflammatory, hepatoprotective, and radical scavenging properties.[Citation10–Citation12] Phycocyanin also prevents cisplatin-induced nephrotoxicity through the inhibition of oxidative stress.[Citation13] Thus, it is a potential therapeutic or protective agent for the treatment of oxidative stress-induced diseases.[Citation14,Citation15] Moreover, phycocyanin has exhibited high therapeutic value in immunomodulation and cancer therapy.[Citation16] However, the application of phycocyanin in different fields has been hindered by its sensitivity to treatment and storage conditions, which can easily cause denaturation, precipitation, and discoloration.
Phycocyanin, an accessory pigment to chlorophyll, is a pigment-protein complex of the phycobiliprotein family that occurs in many cyanobacteria and some red algae.[Citation17] It is formed by α and β subunits and has linear tetrapyrrole phytochromobilin covalently attached to its polypeptides via a thioether linkage to a conserved Cys residue.[Citation18] Phycocyanin exists as a complex interacting mixture of trimer, hexamer, and decamer aggregates which has been reported to be a function of pH and ionic strength of the medium, protein concentration, and algal origin.[Citation1,Citation19–Citation21] Furthermore, the stage of aggregation affects the molecular weight as well as the position and intensity of the maximum absorbance peak of phycocyanin.[Citation22] The stability of phycocyanin depends on its origin, pH, temperature, light, and some exogenous substances.[Citation22–Citation27] Minor differences in the amino acid composition of phycocyanin affect the stability and structural properties of these proteins. Phycocyanin derived from the thermophiles is generally more stable than that derived from the mesophiles.[Citation23,Citation24] Stabilizing agents are usually added to phycocyanin solutions to ensure the long-term stability.[Citation23,Citation25] Besides optimizing the physical and chemical parameters mentioned above, phycocyanin microencapsulation has been developed to improve the storage stability of phycocyanin.[Citation28]
The phycocyanin used in bioactivity studies usually of the high purity, and few reports have investigated food-grade phycocyanin. To enhance the storage stability and maintain the quality of phycocyanin, the anti-microbial agent sodium azide (NaN3) and the protein reducing agent dithiothreitol (DTT) are commonly used as preservatives in C-phycocyanin (CPC) for analytical purposes.[Citation22] Although these compounds have strong preservative effects, they are also toxic and, therefore, cannot be used in preparations intended for human consumption. Therefore, it is necessary to develop an effective, green, and non-toxic method for the stabilization of food-grade phycocyanin. In the present study, to determine the optimal conditions for maintaining phycocyanin stability, we studied the effects of those factors, including pH, temperature, light, and the use of edible stabilizing agents, on the stability of food-grade phycocyanin derived from S. platensis that cultured in seawater-based medium. The antioxidant activity was also determined. This study provides not only suggestions for maintaining the stability and biological activity of phycocyanin during the storage process, but also useful information for the development and application of S. platensis cultured in seawater-based medium.
MATERIALS AND METHODS
Materials and Culture Conditions
The S. platensis used in this experiment was a seawater domesticated strain and cultured in seawater-based medium in open raceway ponds in Sanya, as previously described.[Citation4] A semi-continuous culture mode was adopted, and the S. platensis cells were harvested by filtration daily.
Extraction and Purification of Phycocyanin
The S. platensis was suspended in phosphate buffer (50 mM, pH 6.8) and subjected to several freezing (–20ºC)/thawing (4ºC) cycles in darkness. The mixture was then centrifuged at 8000 × g (5804R, Eppendorf, Hamburg, Germany) for 30 min at 4ºC to collect the phycocyanin-containing supernatant. The supernatant was subsequently fractionated by precipitation with two saturations of ammonium sulfate at 30 and 65%. The precipitate from the 65% saturation of ammonium sulfate was collected by centrifugation at 8000 × g for 20 min at 4ºC. The precipitate was resuspended in phosphate buffer (50 mM, pH 6.8) and dialyzed against double distilled water at 4ºC for 48 h in darkness by intermittently changing the distilled water at regular intervals. The phycocyanin containing solution was then centrifuged at 10,000 × g for 20 min at 4ºC to remove the denatured protein. A food-grade phycocyanin powder was obtained after lyophilization.
Assessment of Phycocyanin Stability
For the stability assessment, the food-grade phycocyanin powder was solubilized in phosphate-citrate buffer to a final concentration of 0.4 mg/mL.
pH Stability
Phycocyanin solutions were prepared in phosphate-citrate buffer at pH values of 3.0, 4.0, 5.0, 6.0, 7.0, and 8.0. The ultraviolet-visible (UV-Vis) absorption spectra of phycocyanin solutions were recorded on a UV-Vis spectrophotometer (UV-2550, Shimadzu, Tokyo, Japan) between 250 and 700 nm with a 1-cm-path-length quartz cuvette. The purity ration of phycocyanin (A620/A280) was calculated using the UV-Vis absorption spectra data. Based on the UV-Vis absorption spectra, phycocyanin solutions at pH 5.0, 5.5, 6.0, 6.5, and 7.0 were chosen to further investigate the effects of pH at two temperatures (55 and 65ºC) for the indicated durations.
Temperature Stability
Phycocyanin solutions at pH 5.0, 6.0, and 7.0 were incubated in a hot water bath for 30 min at 11 temperatures between 25 and 75ºC in 5ºC intervals. To further investigate the effects of short-term incubation, the phycocyanin solution was incubated at four temperatures (60, 65, 70, and 75ºC) for 1, 2, and 4 min.
Light Stability
Phycocyanin solutions at pH 5.0, 6.0, and 7.0 were exposed to lamps emitting light of 50 and 100 μmol m–2 s–1 total intensity at room temperature (25ºC) for 2, 4, 8, 12, 24, and 36 h.
Stability in the Presence of Stabilizing Agents
Sucrose, glucose, or sodium chloride were added to the phycocyanin solutions at pH 5.0, 6.0, 7.0, and 8.0 at a concentration of 20% (w/v). The phycocyanin solutions containing stabilizing agents were incubated in a hot water bath at 65ºC for 30 min. To further investigate the effect of the stabilizing agents, the phycocyanin solution at pH 6.0 with the indicated concentrations of sucrose, glucose, or sodium chloride within their maximum permissible levels was incubated at 65ºC for 30 min.
Analysis
The absorbance of each sample was measured at 620 and 652 nm. The C-phycocyanin (CPC) and allophycocyanin (APC) concentration of the solution were calculated using the following equations:[Citation29,Citation30]
The phycocyanin concentration in the solution was the sum of the CPC and APC concentrations. The relative concentration of phycocyanin (CR) used to quantify the stability was expressed as the percentage of the remaining phycocyanin concentration of the treated samples over that of the initial concentration, as previously described.[Citation25]
In the temperature stability assay, the degradation rate constant (κ) used to estimate the thermal degradation of phycocyanin solution and half-life value (T1/2) which is the time required for phycocyanin concentration to fall to half its initial concentration was calculated as described by Chaiklahan.[Citation25]
Determination of Antioxidant Activity of Phycocyanin
2,2′-azino-bis-(3-ethylbenzthiazoline-6-sulfonic acid) (ABTS) radical-scavenging assay
The ABTS scavenging activity was measured using spectrophotometric method with minor modifications.[Citation31,Citation32] Briefly, the ABTS stock solution was prepared by mixing ABTS (7 mM) and potassium persulfate (2.45 mM) in equal quantities. After being stored in the dark for 18 h, the stock solution was diluted with phosphate buffer saline (PBS) (0.02 M, pH 7.4) to a working solution yielding an absorbance of 0.70 ± 0.05 at 734 nm. To measure the antioxidant capacity, 20 μL aliquots of the phycocyanin solutions of different concentrations were mixed with 180 μL of the ABTS working solution. The absorbance of the mixture was then measured at 734 nm after 6 min of incubation in darkness at room temperature. The ABTS radical-scavenging activity of the samples was expressed as S% = ([Acontrol – Atest – Asample] /Acontrol]) × 100, where Acontrol is the absorbance of the blank control (ABTS working solution with PBS), Atest is the absorbance of ABTS working with sample solution, and Asample is the absorbance of sample solution with PBS. In this experiment, Trolox was used to generate a standard curve. The Trolox equivalent antioxidant capacity (TEAC) of sample was calculated from the Trolox standard curve and expressed as millimoles of Trolox equivalents (TEs) per gram of sample (mmol TE/g). The IC50, defined as the concentration of sample necessary to cause 50% inhibition, was calculated using the relationship between the percentage scavenging activity and the sample concentration.
α,α-Diphenyl-β-pricrylhydrazyl (DPPH) radical-scavenging assay
The DPPH scavenging activity was measured as previously described with minor modifications.[Citation33] First, 20 μL aliquots of phycocyanin solution at different concentrations were mixed with 180 μL of DPPH in methanol solution (0.06 mM). An equal amount of PBS and DPPH served as the control. After incubation for 30 min in darkness at room temperature, the absorbance was recorded at 517 nm. The calculations of the DPPH radical-scavenging activity and IC50 were conducted analogously to the ABTS scavenging calculations previously described.
Statistical Analysis
The data obtained were expressed as the mean ± standard deviation, and all experiments were completed in triplicate. Differences of p < 0.05 and p < 0.01 were considered statistically significant. All statistical analyses were performed using OriginPro 8.6 software.
RESULTS AND DISCUSSION
Effect of pH on Phycocyanin Stability
Phycocyanin is generally graded according to its purity ratio (A620/A280), which is indicative of the purity of the preparation with respect to contaminating proteins.[Citation34] A purity of 0.7 is considered to be food grade, 3.9 is considered to be reactive grade and 4.0 or greater is considered to be analytical grade.[Citation35] Various methods have been developed for the separation and purification of phycocyanin, such as chromatography, gradient centrifugation, ammonium sulfate precipitation, and membrane process.[Citation36–Citation38] Food-grade phycocyanin with a purity ratio (A620/A280) of approximately 1.5 was obtained in this study due to the more effective removal of contaminating proteins in the ammonium sulfate precipitation step. UV-Vis spectroscopy is a popular analytical method used for the characterization of phycocyanin. The decrease in the relative intensity of the peaks at 620 and 652 nm for the same protein concentration indicates decrease in the phycocyanin content of the aqueous solution. The pH of the dissolvent is one of the most important factors influencing the stability of phycocyanin. Therefore, in the present study, UV-Vis spectroscopy was used to determine the stability of phycocyanin dissolved in phosphate-citrate buffer at different pH values to determine the optimal pH range for the stability of phycocyanin isolated from S. platensis cultured in seawater-based medium. All the phycocyanin solution samples exhibited a characteristic absorption peak at 620 nm and strong absorbance at approximately 280 nm, as shown in . At the same concentration of phycocyanin, the relative intensity of the peaks at 620 and 652 nm was lowest at pH 3.0, followed by pH 4.0. In acidic solutions (pH ≤ 4.5), the phycocyanin structure is unfolded, resulting in protein precipitation.[Citation35] This phenomenon explains why the phycocyanin solutions at pH 3.0 and 4.0 exhibited lower intensities at 620 and 652 nm for the same protein concentration. There were no significant differences among the spectra of phycocyanin at pH 5.0, 6.0, and 7.0. As shown in , the purity ration (A620/A280) calculated using the UV-Vis absorption spectra data exhibited a similar trend.
FIGURE 1 Effect of pH on the spectra of phycocyanin in phosphate-citrate buffer at A: room temperature and B: the corresponding purity ratios (A620/A280). CR values of phycocyanin in phosphate-citrate buffer at C: different pH values after incubation in a water bath at 55ºC and D: 65ºC for different durations in darkness.
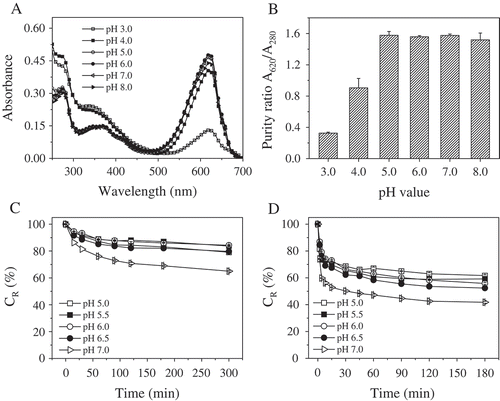
The CR values of the phycocyanin solution at five different pH levels (5.0, 5.5, 6.0, 6.5, and 7.0) incubated at 55 and 65ºC were shown in and . The phycocyanin at pH 7.0 showed the lowest stability, as evidenced by its lowest CR value. After 30 min of incubation at 55 and 65ºC, the CR values of phycocyanin at pH 7.0 decreased to 81.4 and 50.2%, respectively, which were significantly different from the values for the other treated groups (p < 0.05). At 65ºC, phycocyanin was found to be more stable at pH 5.0, but it was more stable at pH 5.5 and 6.0 at 55ºC. This result consistent with the fingdings of Antelo et al., who reported that phycocyanin was more stable at pH 6.0 between 50 and 55ºC, but at pH 5.0 between 57 and 65ºC.[Citation26] Taken together, these results indicate that the optimal pH range for maintaining the stability of food-grade phycocyanin is between 5.0 and 6.0, in agreement with previous work.[Citation22]
Effect of Temperature on Phycocyanin Stability
Temperature is also a decisive factor in determining the stability of phycocyanin. Phycocyanin in aqueous buffers at pH 5.0, 6.0, and 7.0 was thermally stressed from 25 to 75ºC for 30 min. Phycocyanin was found to be stable at temperatures of up to 45ºC, with no significant changes in the CR values observed. The CR values of phycocyanin decreased in a temperature-dependent manner. When the temperature was increased from 45 to 75ºC, the CR values of phycocyanin at pH 5.0, 6.0, and 7.0 decreased from 99.0, 99.1, and 94.8% to 61.0, 55.8, and 48.4%, respectively, as shown in . The phycocyanin solution at pH 7.0 showed the lowest stability of all the tested temperatures. This was particularly evident in the temperature range between 55 to 75ºC. Between 45 and 60ºC, the phycocyanin solution at pH 6.0 was more stable than that at pH 5.0, but above 60ºC the phycocyanin solution at pH 5.0 was more stable. These results were similar to those reported by Antelo et al., who reported that the relationship between the degradation temperature and the pH is inversely proportional with respect to the degradation of the phycocyanin protein.[Citation26] The degradation rate constant (κ) at the indicated temperature increased in a temperature-dependent manner, as shown in . At 50ºC, the half-life values of phycocyanin at pH 5.0, 6.0, and 7.0 were 321.3, 495.0, and 243.6 min, respectively, but the corresponding values decreased to 42.1, 35.7, and 28.6 min at 75ºC, as shown in . The phycocyanin solution at pH 7.0 showed the most degradation at all the temperatures studied, as supported by the decrease in CR and half-life values.
TABLE 1 Degradation rate (κ) and half-life (T1/2) of phycocyanin in phosphate-citrate buffer at pH 5.0, 6.0, and 7.0
FIGURE 2 CR values of phycocyanin in phosphate-citrate buffer at pH 5.0, 6.0, and 7.0 after incubation in a water bath at various temperatures for 30 min in darkness.
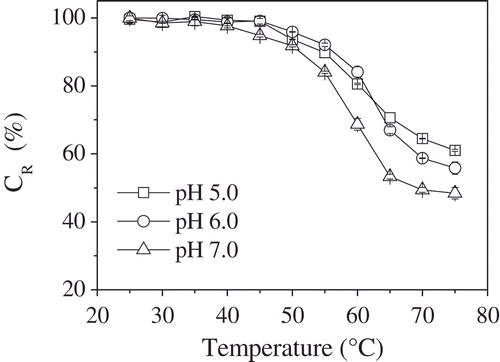
Phycocyanin is very sensitive to heat and undergoes rapid changes when exposed to high temperatures.[Citation27] The CR values of the phycocyanin solution at pH 5.0, 6.0, and 7.0 incubated between 60 and 75ºC for short periods are shown in . The CR values decreased in a time- and temperature-dependent manner. The CR values of phycocyanin at pH 5.0, 6.0, and 7.0 were in the range of 96.3–98.9% after incubation at 60ºC for 1 min. At 75ºC, the CR values of the phycocyanin solution at pH 7.0 decreased to 81.9, 57.9, and 51.4% for 1, 2, and 4 min of treatment, respectively. Between 60 and 65ºC, the phycocyanin solution at pH 6.0 was most stable, but at 70 and 75ºC it was most stable at pH 5.0, as evidenced by the increased CR values. This result is consistent with those for the 30 min treatment previously described.
TABLE 2 CR values of phycocyanin in phosphate-citrate buffer at pH 5.0, 6.0, and 7.0 at the indicated temperatures for short-term treatment in darkness
Effect of Light Exposure on Phycocyanin Stability
The light sensitivity of the phycocyanin solution was studied using light exposure at room temperature (25ºC). After exposure to lamps emitting light at 50 and 100 μmol m–2 s–1 total intensity, the photochemical effect was measured by spectrophotometry. The CR values of the phycocyanin solution at pH 5.0, 6.0, and 7.0 decreased in a time-dependent manner, as shown in . At the same light intensity, the phycocyanin solution at pH 6.0 showed a slightly lower degradation than that at pH 5.0 and 7.0. A slightly higher level of degradation was observed after exposure to 100 μmol m–2 s–1 light than after exposure to 50 μmol m–2 s–1 light. Although light influenced the stability of phycocyanin, the CR values of phycocyanin at pH 5.0, 6.0, and 7.0 were still approximately 80% after continuous exposure to 100 μmol m–2 s–1 light for 36 h.
Effect of Stabilizing Agents on Phycocyanin Stability
The degradation of the protein fraction was reported to have a significant impact on the color maintenance of phycocyanin.[Citation39] To preserve the color and avoid phycocyanin denaturation due to adverse chemical changes, stabilizing agents are often used as preservatives to protect the structural order of the protein chains. Glucose, sucrose, citric acid, sorbic acid, sodium chloride, ascorbic acid, and sodium azide are commonly used as stabilizing agents for phycocyanin.[Citation23,Citation25,Citation26] The addition of sodium azide and sorbic acid did not have a significant effect on the stability of phycocyanin extracted from Spirulina sp.[Citation25] Considering the desired applications and the cost of various stabilizing agents, we selected sodium chloride, sucrose, and glucose as promising stabilizing agents for food-grade phycocyanin derived from S. platensis. The effects of these edible stabilizing agents on the CR values of phycocyanin solution are shown in . The addition of sodium chloride, sucrose or glucose at a concentration of 20% (w/v) resulted in a reduction of the CR value compared to the control, which did not include stabilizing agents. The addition of sodium chloride had the least effect on the CR value, causing a reduction of less than 8% at various pH levels. Nevertheless, after exposure to 65ºC for 30 min, the CR values of these samples with the addition of stabilizing agents was greater than that of the control sample. The CR values of the phycocyanin solutions at pH 5.0, 6.0, 7.0, and 8.0 with the addition of sodium chloride were significantly higher than those of the control and the solutions with other stabilizing agents (p < 0.05).
FIGURE 4 CR values of the phycocyanin solution at pH 5.0, 6.0, 7.0, and 8.0 with 20% sucrose, glucose or NaCl (w/v) after heating at A: 65ºC for 30 min. CR values of the phycocyanin solution at B: pH 6.0 with sucrose, glucose or NaCl at each concentration after incubation at 65ºC for 30 min.
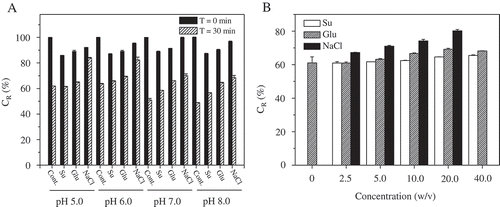
To examine the effects of these stabilizing agents at various concentrations within their maximum permissible levels, the CR values of treated phycocyanin were measured. After incubation at 65ºC for 30 min, the CR values of the phycocyanin solution at pH 6.0 with the addition of stabilizing agents of various concentrations increased to different degrees, as shown in . The CR values of the phycocyanin solution with the addition of sodium chloride were significantly higher than those of the control and increased in a concentration-dependent manner. The CR values were 67.3, 71.1, 74.2, and 80.2% for 2.5, 5.0, 10.0, and 20.0% (w/v) sodium chloride, respectively, while no significant changes were observed after sucrose addition. It was obvious that sodium chloride was a suitable stabilizing agent for protecting phycocyanin from degradation.
Antioxidant Activity of Phycocyanin
CPC, the major phycobiliprotein in S. platensis, contained high level of glutamic acid (GLU), aspartic acid (ASP), alanine (ALA), leucine (LEU), arginine (ARG), isoleucine (ILE), serine (SER), glycine (GLY), and threonine (THR),[Citation40] most of which have been reported to be related to higher antioxidant activity levels.[Citation41] The antioxidant activities of the food-grade phycocyanin solution were evaluated by the ABTS and DPPH methods, which are often used in the determination of the antioxidant abilities of natural compounds.[Citation32,Citation42] The ABTS radical-scavenging activity of phycocyanin solution at different concentrations is shown in . As seen, the ABTS radical-scavenging activity increased in a concentration-dependent manner. The IC50 value and the TEAC for ABTS radical scavenging activity were found to be 0.56 ± 0.02 mg/mL and 0.75 ± 0.01 mmol TE/g phycocyanin, respectively (). The DPPH radical-scavenging activities of the phycocyanin solution at different concentrations are shown in . The activities increased in a concentration-dependent manner. The IC50 value for the DPPH radical scavenging activity was 1.86 ± 0.18 mg/mL (). It is known that oxidative reactions and reactive oxygen species (ROS) are involved in various diseases including atherosclerosis, diabetes, and Alzheimer’s disease.[Citation43,Citation44] Data from both assays clearly demonstrated that food-grade phycocyanin possesses significant antioxidant properties, suggesting that food-grade phycocyanin maybe improve human health upon consumption.
TABLE 3 Antioxidant activity of phycocyanin as determined by TEAC and DPPH methods
CONCLUSIONS
The present study, demonstrated that the optimal pH range for food-grade phycocyanin obtained from S. platensis cultured in seawater-based medium was influenced by the working temperature; the higher working temperature, the lower the optimal pH within a certain range. The food-grade phycocyanin solution remained stable at temperatures up to 45ºC but was light-sensitive at room temperature. Stabilizing agents helped maintain the stability of phycocyanin. The optimal conditions for preserving the stability of food-grade phycocyanin obtained from S. platensis were found to be a pH of 5.0–6.0, low temperature, darkness, and the addition of edible stabilizing agents, such as sodium chloride. Food-grade phycocyanin also exhibited good antioxidant capacities, making it an excellent health food for human consumption.
FUNDING
This project was financially supported by the Knowledge Innovation Program of the Chinese Academy of Sciences (No. SQ201213), the National Natural Science Foundation of China (No. 31402320), the Guangdong Province and Chinese Academy of Science Cooperation Foundation (No. 2012B091100268), the Ocean Public Welfare Scientific Research Project (No. 201305018-3), and the Shenzhen Innovative Development of Marine Economy Regional Demonstration Foundation (SZHY2012-B01-003).
Additional information
Funding
REFERENCES
- Ciferri, O. Spirulina, the Edible Microorganism. Microbiology Reviews 1983, 47, 551–578.
- Kulshreshtha, A.; Zacharia, A.J.; Jarouliya, U.; Bhadauriya, P.; Prasad, G.B.; Bisen, P.S. Spirulina in Health Care Management. Current Pharmaceutical Biotechnology 2008, 9, 400–405.
- Costa, J.A.; Colla, L.M.; Duarte Filho, P.F. Improving Spirulina Platensis Biomass Yield Using a Fed-Batch Process. Bioresource Technology 2004, 92, 237–241.
- Wu, B.T.; Tseng, C.K.; Xiang, W.Z. Large-Scale Cultivation of Spirulina in Seawater Based Culture-Medium. Botanica Marina 1993, 36, 99–102.
- Zhang, F.; Xiang, W.Z.; Xiao, B.; Chen, P.Y. CO2 Sequestration Coupled with Industrial Cultivation of Microalgae. Acta Microbiologica Sinica 2012, 52, 1378–1384.
- Shih, C.M.; Cheng, S.N.; Wong, C.S.; Kuo, Y.L.; Chou, T.C. Antiinflammatory and Antihyperalgesic Activity of C-Phycocyanin. Anesthesia and Analgesia 2009, 108, 1303–1310.
- Seo, Y.C.; Choi, W.S.; Park, J.H.; Park, J.O.; Jung, K.H.; Lee, H.Y. Stable Isolation of Phycocyanin from Spirulina Platensis Associated with High-Pressure Extraction Process. International Journal of Molecular Sciences 2013, 14, 1778–1787.
- Glazer, A.N. Phycobiliproteins a Family of Valuable, Widely Used Fluorophores. Journal of Applied Phycology 1994, 6, 105–112.
- Singh, P.; Kuddus, M.; Thomas, G. Isolation and Binding Affinity of C-Phycocyanin to Blood Cells and Genomic DNA as Well as Its Diagnostic Applications. Journal of Biotechnology and Pharmaceutical Research 2011, 2, 001–008.
- Romay, C.; González, R.; Ledón, N.; Remirez, D.; Rimbau, V. C-Phycocyanin: A Biliprotein with Antioxidant, Anti-Inflammatory, and Neuroprotective Effects. Current Protein & Peptide Science 2003, 4, 207–216.
- Remirez, D.; Fernández, V.; Tapia, G.; González, R.; Videla, L.A. Influence of C-Phycocyanin on Hepatocellular Parameters Related to Liver Oxidative Stress and Kupffer Cell Functioning. Inflammation Research 2002, 51, 351–356.
- Bertolin, T.E.; Farias, D.; Guarienti, C.; Petry, F.T.S.; Colla, L.M.; Costa, J.A.V. Antioxidant Effect of Phycocyanin on Oxidative Stress Induced with Monosodium Glutamate in Rats. Brazilian Journal of Medical and Biological Research 2011, 54, 733–738.
- Fernández-Rojas, B.; Medina-Campos, O.N.; Hernández-Pando, R.; Negrette-Guzmán, M.; Huerta-Yepez, S.; Pedraza-Chaverri, J. C-Phycocyanin Prevents Cisplatin-Induced Nephrotoxicity Through Inhibition of Oxidative Stress. Food Function 2014, 5, 480–490.
- Khan, M.; Varadharaj, S.; Shobha, J.C.; Naidu, M.U.; Parinandi, N.L.; Kutala, V.K.; Kuppusamy, P. C-Phycocyanin Ameliorates Doxorubicin-Induced Oxidative Stress and Apoptosis in Adult Rat Cardiomyocytes. Journal of Cardiovascular Pharmacology 2006, 47, 9–20.
- Farooq, S.M.; Boppana, N.B.; Asokan, D.; Sekaran, S.D.; Shankar, E.M. C-Phycocyanin Confers Protection Against Oxalate-Mediated Oxidative Stress and Mitochondrial Dysfunctions in MDCK Cells. Plos One 2014, 9, e93056.
- Oliveira, E.G.; Rosa, G.S.; Moraes, M.A.; Pinto, L.A.A. Phycocyanin Content of Spirulina Platensis Dried in Spouted Bed and Thin Layer. Journal of Food Process Engineering 2008, 31, 34–50.
- Glazer, A.N. Light Guides. Directional Energy Transfer in a Photosynthetic Antenna. Journal of Biological Chemistry 1989, 264, 1–4.
- De Marsac, N.T.; Cohen-Bazire, G. Molecular Composition of Cyanobacterial Phycobilisomes. Proceedings of the National Academy of Sciences of the United States of America 1977, 74, 1635–1639.
- Gantt, E. Phycobilisomes. Annual Review of Plant Physiology 1981, 32, 327–347.
- Berns, D.S.; Maccoll, R. Phycocyanin in Physical-Chemical Studies. Chemical Reviews 1989, 89, 807–825.
- Maccoll, R. Cyanobacterial Phycobilisomes. Journal of Structural Biology 1999, 124, 311–334.
- Mishra, S.K.; Shrivastav, A.; Mishra, S. Effect of Preservatives for Food Grade C-PC from Spirulina Platensis. Process Biochemistry 2008, 43, 339–345.
- Chen, C.H.; Berns, D.S. Comparison of the Stability of Phycocyanins from Thermophilic, Mesophilic, Psychrophilic, and Halophilic Algae. Biophysical Chemistry 1978, 8, 203–213.
- Kao, O.H.; Edwards, M.R.; Berns, D.S. Physical-Chemical Properties of C-Phycocyanin Isolated from an Acido-Thermophilic Eukaryote, Cyanidium-Caldarium. Biochemical Journal 1975, 147, 63–70.
- Chaiklahan, R.; Chirasuwan, N.; Bunnag, B. Stability of Phycocyanin Extracted from Spirulina sp.: Influence of Temperature, pH, and Preservatives. Process Biochemistry 2012, 47, 659–664.
- Antelo, F.S.; Costa, J.A.V.; Kalil, S.J. Thermal Degradation Kinetics of the Phycocyanin from Spirulina Platensis. Biochemical Engineering Journal 2008, 41, 43–47.
- Jespersen, L.; Stromdahl, L.D.; Olsen, K.; Skibsted, L.H. Heat and Light Stability of Three Natural Blue Colorants for Use in Confectionery and Beverages. European Food Research and Technology 2005, 220, 261–266.
- Yan, M.Y.; Liu, B.; Jiao, X.D.; Qin, S. Preparation of Phycocyanin Microcapsules and Its Properties. Food and Bioproducts Processing 2014, 92, 89–97.
- Bennett, A.; Bogorad, L. Complementary Chromatic Adaptation in a Filamentous Blue-Green Alga. Journal of Cell Biology 1973, 58, 419–435.
- Patel, A.; Mishra, S.; Pawar, R.; Ghosh, P.K. Purification and Characterization of C-Phycocyanin from Cyanobacterial Species of Marine and Freshwater Habitat. Protein Expression and Purification 2005, 40, 248–255.
- Zou, Y.P.; Chang, S.K.C.; Gu, Y.; Qian, S.Y. Antioxidant Activity and Phenolic Compositions of Lentil (Lens Culinaris var. Morton) Extract and Its Fractions. Journal of Agricultural and Food Chemistry 2011, 59, 2268–2276.
- Ko, H.J.; Ang, L.H.; Ng, L.T. Antioxidant Activities and Polyphenolic Constituents of Bitter Bean Parkia Speciosa. International Journal of Food Properties 2014, 17, 1977–1986.
- Sun, Z.; Liu, J.; Zeng, X.; Huangfu, J.; Jiang, Y.; Wang, M.; Chen, F. Astaxanthin is Responsible for Antiglycoxidative Properties of Microalga Chlorella Zofingiensis. Food Chemistry 2011, 126, 1629–1635.
- Antelo, F.S.; Anschau, A.; Costa, J.A.V.; Kalil, S.J. Extraction and Purification of C-Phycocyanin from Spirulina Platensis in Conventional and Integrated Aqueous Two-Phase Systems. Journal of the Brazilian Chemical Society 2010, 21, 921–926.
- Patil, G.; Chethana, S.; Sridevi, A.S.; Raghavarao, K.S. Method to Obtain C-Phycocyanin of High Purity. Journal of Chromatography A 2006, 11279, 76–81.
- Bryant, D.A.; Cohen-Bazire, G. Effects of Chromatic Illumination on Cyanobacterial Phycobilisomes. Evidence for the Specific Induction of a Second Pair of Phycocyanin Subunits in Pseudanabaena 7409 Grown in Red Light. European Journal of Biochemistry 1981, 119, 415–424.
- Chaiklahan, R.; Chirasuwan, N.; Loha, V.; Tia, S.; Bunnag, B. Separation and Purification of Phycocyanin from Spirulina sp. Using a Membrane Process. Bioresource Technology 2011, 102, 7159–7164.
- Yan, S.G.; Zhu, L.P.; Su, H.N.; Zhang, X.Y., Chen, X.L.; Zhou, B.C.; Zhang, Y.Z. Single-Step Chromatography for Simultaneous Purification of C-Phycocyanin and Allophycocyanin with High Purity and Recovery from Spirulina (Arthrospira) Platensis. Journal of Applied Phycology 2011, 23, 1–6.
- Fukui, K.; Saito, T.; Noguchi, Y.; Kodera, Y.; Matsushima, A.; Nishimura, H.; Inada, Y. Relationship Between Color Development and Protein Conformation in the Phycocyanin Molecule. Dyes Pigments 2004, 63, 89–94.
- Yin, G.; Liu, Z.; Liu, F.; Li, C.; Ding, F. X.; Yuan, N. J. Isolation and Characterization of C-Phycocyanin from Spirulina Platensis. Chemical Research in Chinese Universities 1999, 15, 35–38.
- Tounkara, F.; Bashari, M.; Le, G.W.; Shi, Y.H. Antioxidant Activities of Roselle (Hibiscus Sabdariffa L.) Seed Protein Hydrolysate and Its Derived Peptide Fractions. International Journal of Food Properties 2014, 17, 1998–2011.
- Wang, W.; Zhang, J.; Peng, J.; Liu, T.; Xin, Z. Isolation, Identification, and Antioxidant Activity of Bound Phenolic Compounds Present in Rice Bran. Food Chemistry 2015, 171, 40–49.
- Mohesnzadegan, M.; Mirshafiey, A.; The Immunopathogenic Role of Reactive Oxygen Species in Alzheimer Disease. Iranian Journal of Allergy, Asthma, and Immunology 2012, 11, 203–216.
- Sharma, A.; De Haan, J.B. Reactive Oxygen Species and Diabetes-Associated Atherosclerosis—Evidence from Experimental Models and Targeted Antioxidant Therapy. In Systems Biology of Free Radicals and Antioxidants; Laher, I.; Ed.; Springer-Verlag: Berlin, Heidelberg, Germany. 2014; 3467–3491.