Abstract
In this study, biodegradable and biocompatible zein films were prepared by electrophoretic deposition using indium tin oxide electrodes with two different modes: vertical and horizontal electric fields. The effects of the different mode of the electric field in the presence of ethanol on mechanical, hydrophobicity, water resistance properties, microstructures, and thermal stability of zein films were investigated. The results indicated that the physiochemical properties (tensile strength, hydrophobicity, water vapor permeability, water absorption, and thermal stability) of zein films prepared from the vertical electric field were superior to those of zein film produced from the horizontal electric field and the control. The results of scanning electron microscope demonstrated the presence of the compact and homogenous structures of zein films manufactured in electric fields. In summary, the knowledge achieved from this study could facilitate to prepare the zein-based films using a readily and scale-up method.
INTRODUCTION
For more than a century, petroleum propelled the economic progress in the world. In the past three decades, the energy crisis stunted world economic growth due to the limited supply of petroleum. Scientists in the academia and industrial sector have dedicated their efforts to the development of new substitutes for petroleum-based fuels and chemical intermediates.[Citation1] Compared with conventional petroleum based materials, consumer interest in plant-derived polymers achieved from renewable resources has dramatically increased in recent years, due to their biocompatibility, biodegradability, and commercial availability at relatively low cost, for instance, lipids,[Citation2–Citation7] proteins,[Citation8–Citation13] and polysaccharides.[Citation14–Citation17] Zein, a yellowish, odorless, and tasteless set of prolamine proteins, generally contains polypeptides α-, β-, γ-, and δ-zeins.[Citation18] It has been commercially isolated from corn gluten meal, a byproduct of the corn wet-milling process, which is commonly used as a non-toxic and natural weed suppressant and fertilizer for grass lawns.[Citation19] The main structure of zein is composed of a helical wheel configuration with nine homologous identical units in an anti-parallel state,[Citation20] containing hydrophobic (>50%) and hydrophilic (<50%) domains on the surfaces of molecules.[Citation21] Thus, the different concentrations of ethanol were generally used as a common organic solvent since it is non-toxic and readily to be evaporated away from the reaction media.[Citation9] In the literature, zein-based biopolymers have been investigated as packaging zein films,[Citation22–Citation25] or zein-based film combining with lysozyme,[Citation26] thymol,[Citation27] and oleic acid.[Citation28]
Two methods (solvent-casting and hot-melt extrusion) have been predominantly utilized in the manufacture of edible thin films. Solvent-casting is considered as a traditional and classical film-making approach. In general, polymers are carefully suspended or dissolved in a volatile solvent and dispersed onto a continuous roll of release media. The coated media is conveyed through a drying device for the evaporation of the solvents. For the hot-melt extrusion method, polymers are homogenized and heated in an extruder screw. The melted and homogeneous mixture is propelled through a die and coated on a rolling media. Both methods require expensive equipment or an apparatus for the scale-up production of packaging films. Moreover, the high temperature in hot-melt extrusion could negatively affect the appearances of the films and lead to the thermal degradation of the substances in the film.[Citation29–Citation31]
The electrophoretic deposition (EPD) technique has been commercially used in industrial-scale process with a wide range of new applications in traditional and advanced materials, including solar cells,[Citation32] photoelectrodes,[Citation33] phosphor screens,[Citation34,Citation35] and ceramic coatings.[Citation36] EPD allows for the migration of colloidal particles suspended in a liquid medium.[Citation37] Those charged particles deposited onto an electrode, resulting in the formation of a film with greatly homogeneity and packing density. It is a simple, efficient, automated, cost-effective, and high-throughput process. In addition, solvent-casting could consume a large amount of organic solvent, raising environmental awareness. For EPD, the organic solvent could be recycled, reducing the environmental impact. Furthermore, the thickness, packing density, and the amount of colloids fabricated using EPD can be readily regulated by altering some EPD parameters for instance, the electric field, concentration of electrolyte, and deposition time.[Citation32] Indium tin oxide (ITO) is a solid state of indium (III) oxide (In2O3) and tin (IV) oxide (SnO2), which is generally used in electrodes due to their wide electrochemical potential windows, high electrical conductivity, and optical transparency.[Citation38]
In literature, the formation of the film from edible resources using EPD technique is scarcely reported. In addition, most of electric fields applied are vertical and the impacts of horizontal electric fields are rarely released. Herein, the primary objective of this study was to prepare zein films by using the EPD technique. The secondary goal of this investigation was to characterize and to compare the physicochemical properties of zein films prepared at the vertical and horizontal electric fields, including tensile strength (TS), elongation at break, water vapor permeability (WVP), surface hydrophobicity, microstructures, and thermal stability.
EXPERIMENTAL
Materials
Zein, regular grade, was obtained from Gaoyurixing Industries Inc. (Jiangsu, China) with its composition mainly being 94.7% protein, 2.1% moisture, and 1.4% lipid. Ethyl alcohol (high-performance liquid chromatography [HPLC] grade), isopropanol (HPLC grade), glycerol were purchased from Sigma-Aldrich (Shanghai, China). Other chemicals were achieved from Tianyi (Tianjin, China). All commercial chemicals were used without further purification.
Preparation of Zein Film by Using EPD Techniques
Zein stock solutions contained zein (0.15 g/mL) and 75–95% ethanol solution. The solution was continuously stirred and maintained at 60°C for 20 min. EPD was conducted in the stock solutions consisting of zein (0.15 g/mL) and ethanol–water (75–95%) solvent. In this study, the glycerol was used at a ratio of 20% (glycerol/ zein, w/w) for all groups. The schematic of the EPD device is shown in . The EPD device included the substrate and ITO electrodes (5 cm × 2.5 cm). The distance between the substrate and counter electrode was 25 mm. The deposition process was performed at a constant voltage of 100 V for 10 min. The films achieved from the surface of the electrodes were placed in 50% relative humidity (RH) desiccator under 23 ± 2°C before further use. The control was produced based on the method in our previous article with a different concentration.[Citation9] The stock solution was carefully cast onto a polyethylent film (150 × 250 mm) using an applicator (Model CS-194AV, Toyoseiki Ltd. Japan). The zein films were conditioned in 50% RH desiccator under 23 ± 2°C before further use. After equilibrium is reached, the water content of film is approximately 15%.
Mechanical Properties
The mechanical properties (TSs and elongations to break of the films) of films were evaluated by an electronic universal testing machine (Shenzhen Reger Instrument Co., Ltd., Shenzhen, China), following the protocol.[Citation9] The rectangular test strips of the films were modified into a shape with a length of 80 mm and a width of 25 mm, and the cross-head speed was set at 10 mm/min. The temperature of the laboratory was constantly maintained at 20–23°C, and the RH was 50 ± 4%. Minimally four replications were used to determine the ultimate TS and elongation to break. TS was calculated by dividing the maximum load at break by the area of cross-section. Elongation at break (E, %) was expressed as:
where L0 (mm) is the initial length of the film and L1 (mm) is its length at break.
Contact Angle Measurements
Contact angle measurements were performed using a Data Physics OCA-15 plus setup according to the early publication from our group.[Citation9] Sample liquids were gently dropped onto the surface of hydrophilic glass slides. The contact angle values from droplet images where a best fit between a theoretical Laplacian curve and the experimental profiles were automatically determined and recorded. Four measurements of contact angle were carried out and averaged for each sample.
Water Absorption Measurement
Water absorption was measured by the ASTM D87016 following the method previously described in the literature.[Citation9] Initially, films were incubated at 25°C and 50% RH. After being chopped into three 30 × 30 mm specimens, samples were stored at 25°C and 50% RH for 2 days to reach equilibrium. Beakers were pre-dried at 60°C for 48 h and the weight was recorded. The chopped sample was immersed in distilled water in a beaker at 25°C for up to 3 h. Subsequently, after the surface water was carefully wiped off, the weight of the sample was monitored. Water absorption was calculated as a percentage ratio of submerged water to initial dry weight. Loss of soluble materials from the samples after 3 h of soaking was recorded by evaporating away immersing water in the beaker at 60°C for 1 day and then weighing the alternation of the beaker.
WVP Measurement
The WVP of the zein films was measured using the method reported by our group.[Citation9] In this method, the zein film was cut to 2 cm in diameter, and the film was used to seal a testing cup containing calcium chloride. This cup was placed in a controlled chamber at 25°C and 90% RH. The weight of the cup was measured intermittently at intervals of 24 h, up to 96 h. The WVP of the zein films was calculated as follows:
where WVP: water vapor permeability (×109 g/m•s•Pa), W is increase in cup weight (g), L is the thickness of film (m), t is measuring time (s), A is the measuring area (m2), and P is the difference in pressure between outside of the cup and inside of the cup (Pa).
Differential Scanning Calorimetry (DSC)
DSC analysis was conducted on a DSC-60A Thermal Analyzer (Shimadzu Inc., Japan) supplied with compressed nitrogen gas. The procedure followed the published method.[Citation31] An empty pan was used as a reference. The instrument was calibrated with indium (ΔHfusion = 28.59 J/g, melting point Tonset = 156.60°C). Samples of approximately 15 to 25 mg were placed in aluminum pans and sealed hermetically. Afterward, samples were heated from room temperature to 200°C at a rate of 10°C/min.
Scanning Electron Microscopy (SEM)
The scanning electron images of samples were observed and collected using a scanning electron microscope (SU1510, Hitachi, Japan). The sample was cut into 2 × 2 mm according to a previously published method.[Citation31] In brief, the sample was fixed on a specimen holder with double-sided scotch tape and sputter-coated with gold (5 min, 2 mbar). Afterward, each sample was transferred to the scanning electron microscope at an acceleration voltage of 20 kV and a magnification of 10,000×.
Statistical Analysis
Averages and standard deviations (SD) from at least four measurements of each sample were reported in this study. Data are expressed as mean ± SD. Student’s t-test was used for analysis of the test results (least significant difference) at the significance level of p-value <0.05 using the SAS Software (SAS Institute Inc., Cary, NC, USA).
RESULTS AND DISCUSSION
is a schematic representation of the proposed effect of EPD using ITO electrodes on the formation of zein-based films. It is well-recognized that the hydrophobic feature of zein is highly linked with the high concentration of non-polar amino acid residues, for instance, leucine, proline, and alanine.[Citation18] In general, binary mixtures of aqueous ethanol (60–95, v/v %) and water facilitate the solubility and dispensability of zein particles[Citation39,Citation40] since the solubility extent of zein is greatly related with the high proportion (>50%) of hydrophobic amino acid residues.[Citation11,Citation39]
Initially, the concentration and surface potential of well-dispersed zein nanoparticles in ethanol are carefully programmed to achieve a stable suspension without any aggregation and precipitation. EPD is partly associated with a two-step process (electrophoresis and deposition) conducted in a two-electrode cell (shown in ). In the first step (electrophoresis), charged zein particles in the suspension (hydrophilic part) were migrated toward the deposition electrode triggered by an electric field. The second step (deposition), the deposition process is the sedimentation process of zein suspension. Due to the electric field pushed zein particles in suspension toward the electrode, zein particles accumulate on the surface of the ITO electrode, thereby forming the zein-based film. The vertical () or horizontal () position of ITO electrode electric fields could generate the corresponding electric filed, respectively.
Effects of the Electric Fields on Mechanical Properties of Zein Film
The TS and elongation at break of zein films prepared by vertical and horizontal electric fields, respectively, in the presence of different concentrations of ethanol are illustrated in & . TS refers to the maximal tensile force maintained per the cross sectional area of the film prior to breakage. As shown in , TS of the sample either prepared by vertical electric field or horizontal electric field increased with the increase of the concentration of ethanol from 80 to 90% w/w. The maximal value of TS in vertical electric field (59.4 Mpa) or horizontal electric field (46.8 Mpa) was obtained at 90% of ethanol, which were significantly higher than that of the control (p < 0.05). When the ethanol concentration reached upon 95%, TS significantly decreased for all samples. This trend was in agreement with the zein films prepared by ethanol.[Citation9] It was well-known that amino acid residues of zein were rearranged in ethanol with different concentrations because of the varied polarities of solvents.[Citation11,Citation40] It was proposed that when the electric field was employed, zein particle was closely integrated together and allowed for the formation of the films with uniform and compact structures, thereby leading to the increase of TS. The TS of the sample prepared in horizontal electric field was higher than that prepared in vertical electric field, probably due to the different intensities of internal hydrophobic interactions; and also the gravity of zein particle under the different electric field mode could contribute to the different physicochemical properties of zein films. Mechanical properties could be altered by controlling the orientation of polymer, leading to the formation of the ordered structure, and the crystallinity, thus resulting in the enhancement of TS.[Citation41]
FIGURE 2 Effects of vertical and horizontal electric fields on A: tensile strength and B: elongation percentage of zein films prepared by electrophoretic deposition in ethanol (80–95%, v/v). Black/Left (control); Blue/Middle (vertical); Red/Right (horizontal).
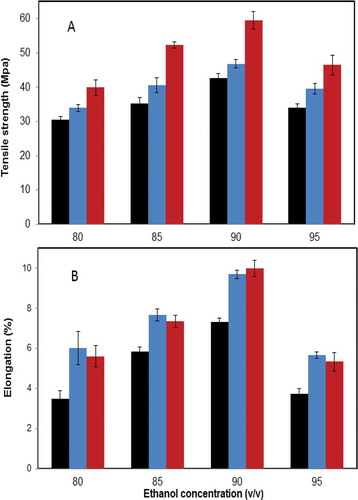
At the initial stage, the elongation of the sample was significantly increased with the improvement of the concentration of ethanol (p < 0.05). The maximal elongation of each sample was achieved when the sample was prepared in 90% ethanol in , regardless of the uses of vertical and horizontal electric fields. No significant differences (p > 0.05) were observed between the vertical electric field and the horizontal electric field when the samples were formed at the same concentration of ethanol. The changes of the distribution of intra- and intermolecular hydrogen bonding of polar and non-polar residues induced by the electric field allowed the alignment of the chains in the field direction, resulting in the enhancement of the mobility of zein films.[Citation20] García et al. reported the formation of hydrogen bonds modified the elongation of the chitosan film when the electric field was employed.[Citation42] Their results illustrated that the electrical treatment increased the mechanical and stretching profiles of films as well as their flexibility.
Effects of Electric Fields on Contact Angles of Zein Films
The contact angle between the surface of film and a drop of water is referred to the angle caused by the liquid-vapor and solid-liquid interfaces.[Citation9] The large contact angle demonstrated the weak attraction between the surfaces of one drop of water and the film. The contact angle is proportional to the extent of hydrophobicity of the film. To better understand the effects of different electric fields on the surface hydrophobicity of the zein film, zein films were measured using the contact angle measurement. As plotted in , the effects of vertical and horizontal electric fields on the static contact angles of zein films prepared from the different concentrations of ethanol. The maximal contact angle of the surface of zein films was observed when the film was manufactured using the horizontal electric field, followed by the sample treated with the vertical electric field and the control in the presence of 90% ethanol, indicating that zein films obtained from the vertical electric field are more significantly hydrophobic than those from the horizontal electric field and the control (p < 0.05). It was hypothesized that hydrophobic amino acids of zein molecules were exposed to the exterior of the surface of zein film when subjected to electric fields. Therefore, zein films treated with electric fields tended toward being more hydrophobic than that of the control. The alternations of the trend of the contact angle of the surface of zein films formed in electric fields were highly in agreement with those prepared in ethanol and isopropanol (80–95%, v/v).[Citation9] The difference of the surface hydrophobicity of films might be attributed to the impact of the different directions of the combination of the gravity of zein particles and electric field.
Effects of Electric Fields on WVP and Water Absorption of Zein Films
The water transport features of the films are considered to be highly correlated with the quality of foods. Hence, the study of WVP and water absorption of packaging films could contribute to the application of the packaging materials. As depicted in , the WVP of zein films significantly decreased with the increase of the concentration of ethanol (up to 90%). When the concentration of ethanol was elevated to 95%, the WVP was slightly enhanced, compared to the minimal value obtained at 90% ethanol regardless of the type of the electric field. The identical trends were perceived for the water absorption of zein films prepared by the vertical electric field and horizontal electric field, evaluated as the proportion of the weight ratio of the treated sample to the control in . Significant differences of both water absorption and WVP of zein films were listed as follows: the horizontal electric field < the vertical electric field < the control at each concentration (p < 0.05) presumably because of the various intensities of internal hydrophobic interactions of zein molecules and the effects of the gravity of zein particles at different electric field modes. The formation of the compact structure in zein films under electric fields could readily reduce the cavities of the porous matrix, hindering the diffusion of water molecules. It was demonstrated that the addition of nanoparticles, for instance montmorillonite (MMT)[Citation43] and TiO2[Citation44] facilitated a decrease of WVP, due to the presence of the compact and well-distributed silicate layer. In addition, the trends of WVP and water absorption of zein films are highly in agreement with the trend of contact angles in this study.
Effects of Electric Fields on Microstructures of Zein Films
Further insight into the microstructure of the deposition of zein film under vertical and horizontal electric fields can be gained by observing –. The control displayed extraordinarily coarse, lumpy, and rugged surface in and cross-section in . In contrast, the treated samples possessed compact and homogenous textures in the surfaces (–) and in the cross-sections (–) regardless of the electric field modes. This might be attributed to the fact that zein particles were orderly rearranged and firmly packed together under the electric field, resulting in the production of the zein film with a uniform and compact pattern. The results were highly consistent with the conclusions achieved from the previous sections in this study. In literature, the similar homogeneous orientation of the nanocomposite coatings of cellulose nanocrystals and alginate prepared by EPD were obtained.[Citation45]
FIGURE 5 Microstructures of zein films prepared by electrophoretic deposition: The surface of the control; A: zein film prepared from vertical electric field; B: horizontal electric field; C: the cross-section of D: the control, zein film prepared from vertical electric field E: and horizontal electric field F: in ethanol (90%, v/v).
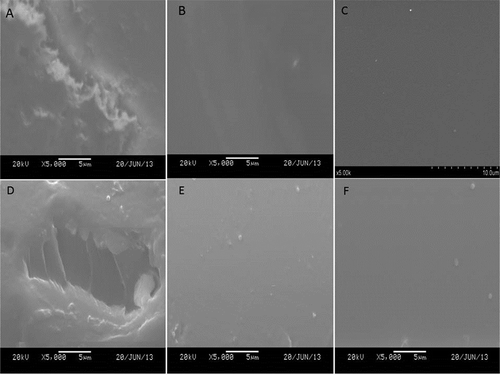
Effects of Electric Fields on Thermal Properties of Zein Films
The differential scanning calorimeter (DSC) is applied to radically understand the thermal capacity of the material regarding the molecular weight, covalent or non-covalent interaction within the molecular structure, amorphous and crystalline regions, and side branches.[Citation9] Therefore, the inherently thermal abilities of zein films prepared by EPD in ethanol were studied by DSC in . The endothermic peaks of zein films correlated with the disassembly of disulfide bridges, hydrophobic interactions, and the denaturation of protein ranged from 80.32 to 87.31°C (Tm). Tm of the zein film treated with the horizontal electric field was higher than those of the samples prepared by the vertical electric field, followed by the control, probably due to the different extents of hydrophobic interactions and the compact structures induced by the electric field. In addition, Tm of the zein film prepared in 90% ethanol was higher than that of the sample prepared in 80% ethanol regardless of the preparation method. It was reported that the high order structure of the protein film corresponded to the higher denaturation temperature.[Citation46] Moreover, the improvement of intermolecular interactions between proteins could cause the enhancement of thermal properties of protein-based films.[Citation8,Citation47]
FIGURE 6 DSC scans of zein film prepared by electrophoretic deposition 1: The control produced from 90% ethanol; 2: The control produced from 80% ethanol; 3: The sample produced from 90% ethanol in the vertical electric field; 4: The sample produced from 80% ethanol in the vertical electric field; 5: The sample produced from 90% ethanol in the horizontal electric field; 6: The sample produced from 80% ethanol in the horizontal electric field.
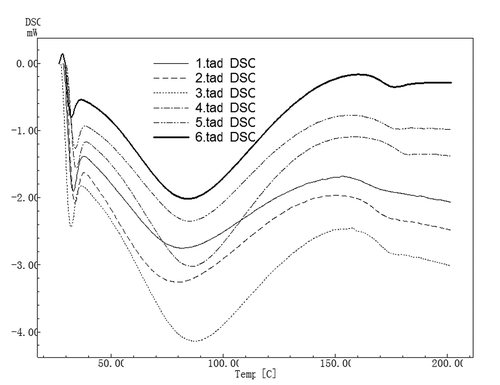
The underlying reasons of the difference of the vertical and horizontal electric fields
When the ethanol solution is evaporated away from the stock solution, zein macromolecules would be randomly rearranged and interconnected in a state of disorder with huge intervals, leading to the formation of different-sized holes in the zein film. In contrast, in the electric field, the charged zein macromolecules orderly move toward the electrode with the opposite charges. Therefore, this arrangement would contribute to the compact structure of zein film, resulting in the improvement of physicochemical properties. The difference between the vertical and horizontal electric fields might be caused by the different drafting-forces which are the combinations of the gravity of zein particle and the electric field force. It is hypothesized that the gravity of zein particle in the horizontal electric field is highly superimposed over the horizontal electric field force due to the identical direction, compared to the vertical filed, thereby leading to its superior physicochemical properties.
CONCLUSIONS
In summary, zein-based films with uniform distribution of fine zein particles were successfully manufactured by EPD with ITO electrodes. In addition, the effects of electric fields with two different modes (vertical and horizontal electric fields) on the physicochemical properties of zein films were investigated. The film prepared in the horizontal electric field displayed the superior TS, water resistance ability, and hydrophobic property. In addition, the employment of EPD allowed for the production of the films with compact and homogeneous structures. The knowledge achieved from this study would prominently contribute to the manufacture of edible films in the food and pharmaceutical industries.
FUNDING
Financial support for this study was kindly supplied by the National Science Foundation of China (grant number: 31271974).
Additional information
Funding
REFERENCES
- Feng, T.; Ye, R. Rheological Behavior of Biopolymer Systems, Handbook of Biopolymer-Based Materials: From Blends and Composites to Gels and Complex Networks 2013, 22, 673–698.
- Ye, R; Pyo, S.-H.; Hayes, D.G. Lipase-Catalyzed Synthesis of Saccharide–Fatty Acid Esters Using Suspensions of Saccharide Crystals in Solvent-Free Media. Journal of the American Oil Chemists’ Society 2010, 87, 281–293.
- Ye, R.; Hayes, D.G. Optimization of the Solvent-Free Lipase-Catalyzed Synthesis of Fructose-Oleic Acid Ester Through Programming of Water Removal. Journal of the American Oil Chemists’ Society 2011, 88, 1351–1359.
- Ye, R.; Hayes, D.G. Lipase-Catalyzed Synthesis of Saccharide-Fatty Acid Esters Utilizing Solvent-Free Suspensions: Effect of Acyl Donors and Acceptors, and Enzyme Activity Retention. Journal of the American Oil Chemists’ Society 2012, 89, 455–463.
- Ye, R.; Hayes, D.G. Solvent-Free Lipase-Catalysed Synthesis of Saccharide-Fatty Acid Esters: Closed-Loop Bioreactor System with in Situ Formation of Metastable Suspensions. Biocatalysis and Biotransformation 2012, 30, 209–216.
- Hayes, D.G.; Mannam, V.K.; Ye, R.; Zhao, H.; Ortega, S.; Montiel, M.C. Modification of Oligo-Ricinoleic Acid and Its Derivatives with 10-Undecenoic Acid Via Lipase-Catalyzed Esterification. Polymers 2012, 4, 1037–1055.
- Zhao, H.; Liu, J.; Lv, F.; Ye, R.; Bie, X.; Zhang, C.; Lu, Z. Enzymatic Synthesis of Lard-Based Ascorbyl Esters in a Packed-Bed Reactor: Optimization by Response Surface Methodology and Evaluation of Antioxidant Properties. LWT–Food Science and Technology 2014, 57, 393–399.
- Wang, Y.; Liu, A.; Ye, R.; Wang, W.; Li, X. Transglutaminase-Induced Crosslinking of Gelatin-Calcium Carbonate Composite Films. Food Chemistry 2015, 1, 414–422.
- Chen, Y.; Ye, R.; Liu, J. Effects of Different Concentrations of Ethanol and Isopropanol on Physicochemical Properties of Zein-Based Films. Industrial Crops and Products 2014, 53, 140–147.
- Ye, R.; Harte, F. Casein Maps: Effect of Ethanol, pH, Temperature, and Cacl2 on the Particle Size of Reconstituted Casein Micelles. Journal of Dairy Science 2013, 96, 799–805.
- Chen, Y.; Ye, R.; Liu, J. Understanding of Dispersion and Aggregation of Suspensions of Zein Nanoparticles in Aqueous Alcohol Solutions after Thermal Treatment. Industrial Crops and Products 2013, 50, 764–770.
- Chen, Y.; Ye, R.; Li, X.; Wang, J. Preparation and Characterization of Extruded Thermoplastic Zein–Poly (Propylene Carbonate) Film. Industrial Crops and Products 2013, 49, 81–87.
- Romero, A.; Cordobés, F.; Guerrero, A.; Puppo, M.C. Influence of Protein Concentration on the Properties of Crayfish Protein Isolated Gels. International Journal of Food Properties 2014, 17, 249–260.
- Feng, T.; Ye, R.; Zhuang, H.; Rong, Z.; Fang, Z.; Wang, Y.; Gu, Z.; Jin, Z. Physicochemical Properties and Sensory Evaluation of Mesona Blumes Gum/Rice Starch Mixed Gels As Fat-Substitutes in Chinese Cantonese-Style Sausage. Food Research International 2013, 50, 85–93.
- Feng, T.; Ye, R.; Zhuang, H.; Fang, Z.; Chen, H. Thermal Behavior and Gelling Interactions of Mesona Blumes Gum and Rice Starch Mixture. Carbohydrate Polymers 2012, 90, 667–674.
- Feng, T.; Su, Q.; Zhuang, H.; Ye, R.; Gu, Z.; Jin, Z. Ghost Structures, Pasting, Rheological, and Textural Properties Between Mesona Blumes Gum and Various Starches. Journal of Food Quality 2014, 37, 73–82.
- Zhang, W.; Zhang, J.; Xia, W. Effect of Ball-Milling Treatment on Physicochemical and Structural Properties of Chitosan. International Journal of Food Properties 2014, 17, 26–37.
- Shukla, R.; Cheryan, M. Zein: The Industrial Protein from Corn. Industrial Crops and Products 2001, 13, 171–192.
- Lawton, J.W. Zein: A history of Processing and Use. Cereal Chemistry 2002, 79, 1–18.
- Argos, P.; Pedersen, K.; Marks, M.; Larkins, B. A Structural Model for Maize Zein Proteins. Journal of Biological Chemistry 1982, 257, 9984–9990.
- Lai, H.M.; Geil, P.; Padua, G. X‐Ray Diffraction Characterization of the Structure of Zein–Oleic Acid Films. Journal of Applied Polymer Science 1999, 71, 1267–1281.
- Biswas, A.; Selling, G.W.; Woods, K.K.; Evans, K. Surface Modification of Zein Films. Industrial Crops and Products 2009, 30, 168–171.
- Ozcalik, O.; Tihminlioglu, F. Barrier Properties of Corn Zein Nanocomposite Coated Polypropylene Films for Food Packaging Applications. Journal of Food Engineering 2013, 114, 505–513.
- Panchapakesan, C.; Sozer, N.; Dogan, H.; Huang, Q.; Kokini, J.L. Effect of Different Fractions of Zein on the Mechanical and Phase Properties of Zein Films at Nano-Scale. Journal of Cereal Science 2012, 55, 174–182.
- Yoshino, T.; Isobe, S.; Maekawa, T. Influence of Preparation Conditions on the Physical Properties of Zein Films. Journal of the American Oil Chemists’ Society 2002, 79, 345–349.
- Güçbilmez, Ç.M.; Yemenicioğlu, A.; Arslanoğlu, A. Antimicrobial and Antioxidant Activity of Edible Zein Films Incorporated with Lysozyme, Albumin Proteins, and Disodium EDTA. Food Research International 2007, 40, 80–91.
- Del Nobile, M.; Conte, A.; Incoronato, A.; Panza, O. Antimicrobial Efficacy and Release Kinetics of Thymol from Zein Films. Journal of Food Engineering 2008, 89, 57–63.
- Scramin, J.A.; de Britto, D.; Forato, L.A.; Bernardes‐Filho, R.; Colnago, L.A.; Assis, O.B. Characterisation of Zein–Oleic Acid Films and Applications in Fruit Coating. International Journal of Food Science and Technology 2011, 46, 2145–2152.
- Verreck, G.; Six, K.; Van den Mooter, G.; Baert, L.; Peeters, J.; Brewster, M.E. Characterization of Solid Dispersions of Itraconazole and Hydroxypropylmethylcellulose Prepared by Melt Extrusion—Part I, International Journal of Pharmaceutics 2003, 251, 165–174.
- Maniruzzaman, M.; Boateng, J.S.; Snowden, M.J.; Douroumis, D. A Review of Hot-Melt Extrusion: Process Technology to Pharmaceutical Products. International Scholarly Research Notices 2012, 2012, 1–9.
- Chen, Y.; Ye, R.; Yin, L.; Zhang, N. Novel Blasting Extrusion Processing Improved the Physicochemical Properties of Soluble Dietary Fiber from Soybean Residue and in Vivo Evaluation. Journal of Food Engineering 2014, 120, 1–8.
- Yum, J.-H.; Kim, S.-S.; Kim, D.-Y.; Sung, Y.-E. Electrophoretically Deposited Tio 2 Photo-Electrodes for Use in Flexible Dye-Sensitized Solar Cells. Journal of Photochemistry and Photobiology A: Chemistry 2005, 173, 1–6.
- Miyasaka, T.; Kijitori, Y.; Murakami, T.N.; Kimura, M.; Uegusa, S. Efficient Nonsintering Type Dye-Sensitized Photocells Based on Electrophoretically Deposited TiO2 Layers. Chemistry Letters 2002, 7, 1250–1251.
- Shane, M.J.; Talbot, J.B.; Schreiber, R.D.; Ross, C.L.; Sluzky, E.; Hesse, K. Electrophoretic Deposition of Phosphors: I. Conductivity and Zeta Potential Measurements. Journal of Colloid and Interface Science 1994, 165, 325–333.
- Russ, B.E.; Talbot, J.B. A study of the Adhesion of Electrophoretically Deposited Phosphors. Journal of the Electrochemical Society 1998, 145, 1245–1252.
- Ferrari, B.; Moreno, R.; Sarkar, P.; Nicholson, P. Electrophoretic Deposition of Mgo from Organic Suspensions. Journal of the European Ceramic Society 2000, 20, 99–106.
- Seuss, S.; Boccaccini, A.R. Electrophoretic Deposition of Biological Macromolecules, Drugs, and Cells. Biomacromolecules 2013, 14, 3355–3369.
- Minami, T. Transparent Conducting Oxide Semiconductors for Transparent Electrodes. Semiconductor Science and Technology 2005, 20, S35.
- Wang, Y.; Padua, G.W. Formation of Zein Microphases in Ethanol-Water. Langmuir 2010, 26, 12897–12901.
- Kim, S.; Xu, J. Aggregate Formation of Zein and Its Structural Inversion in Aqueous Ethanol. Journal Of Cereal Science 2008, 47, 1–5.
- Souza, B.; Cerqueira, M.; Martins, J.; Casariego, A.; Teixeira, J.; Vicente, A. Influence of Electric Fields on the Structure of Chitosan Edible Coatings. Food Hydrocolloids 2010, 24, 330–335.
- Garcia, M.; Pinotti, A.; Martino, M.; Zaritzky, N. Electrically Treated Composite FILMS Based on Chitosan and Methylcellulose Blends. Food Hydrocolloids 2009, 23, 722–728.
- Sothornvit, R.; Hong, S.-I.; An, D.J.; Rhim, J.-W. Effect of Clay Content on the Physical and Antimicrobial Properties of Whey Protein Isolate/Organo-Clay Composite Films. LWT–Food Science and Technology 2010, 43, 279–284.
- Zolfi, M.; Khodaiyan, F.; Mousavi, M.; Hashemi, M. The Improvement of Characteristics of Biodegradable Films Made from Kefiran–Whey Protein by Nanoparticle Incorporation. Carbohydrate Polymers 2014, 109, 118–125.
- Chen, Q.; de Larraya, U.P.; Garmendia, N.; Lasheras-Zubiate, M.; Cordero-Arias, L.; Virtanen, S.; Boccaccini, A.R. Electrophoretic Deposition of Cellulose Nanocrystals (Cns) and Cns/Alginate Nanocomposite Coatings and Free Standing Membranes. Colloids and Surfaces B: Biointerfaces 2014, 118, 41–48.
- Ghanbarzadeh, B.; Oromiehi, A. Thermal and Mechanical Behavior of Laminated Protein Films. Journal of Food Engineering 2009, 90, 517–524.
- Sessa, D.J.; Mohamed, A.; Byars, J.A.; Hamaker, S.A.; Selling, G.W. Properties of Films From Corn Zein Reacted with Glutaraldehyde. Journal of Applied Polymer Science 2007, 105, 2877–2883.