Abstract
The objective of this study was to determine how the addition of two cocoa butter equivalents and cocoa butter improver affect the physical and sensory properties of chocolate. The laboratory-made chocolate samples were tempered at three different pre-crystallization temperatures (25, 27, and 29°C), using different concentrations (3, 5, and 7%) of two commercial cocoa butter equivalents as well as commercial cocoa butter improver of the chocolate. The nucleation time of the chocolate mass primarly depended on pre-crystallization temperature while the value of maximum torque of chocolate mass were influenced by both, pre-crystallization temperature and concentration of fats. Sensory evaluation revealed that cocoa butter equivalents were acceptable in chocolate formulation without producing a negative impact on the sensory quality, while usage of improver required adjustment of raw formulations or process parameters. The results of the instrumentally measured hardness revealed that addition of cocoa butter improver significantly (p > 0.05) increased hardness of chocolate samples.
INTRODUCTION
Cocoa butter (CB) is the continuous lipid phase in chocolate and it surrounds the non-fat components. It has an affect on chocolate properties, such as snap, gloss, appearance, mouthfeel, flavor, on hardness at room temperature, and on fastness and completion of the melting in the mouth.[Citation1,Citation2] At the same time, processing conditions, such as tempering and cooling, depend on characteristics of CB.[Citation3] In the phase of tempering (pre-crystallization) of chocolate mass the aim is to produce stabile V crystal polymorphs of CB. The applied temperature of pre-crystallization is crucial for achieving chocolate of optimal quality with good shape, color, glossy surface, contraction from the mold, better stable product hardness, more heat resistance, rapid melting in the mouth, and longer shelf life. If the chocolate is not tempered properly, it could be over-tempered, under-tempered, or un-tempered.[Citation4,Citation5] Its unusual triacylglycerol composition is causing its unusual behavior.[Citation6]
In order to get the chocolate with the best texture and sensory characteristics, CB must be tempered, because of his triacylglycerol composition. This treatment causes correct crystallization status during processing and determines the quality parameters of the chocolate, such as texture, sensory properties, and shelf life.[Citation3] CB production is very complicated due to difficult cultivation, low productivity, and pest attacks. Also, there is variability in quality.[Citation7] A comprehensive overview of the composition of CB and climatic influences on the composition is published by Chalseri and Dimick.[Citation8] Furthermore, world cocoa prices are on the rise in the last years due to increasing demand.[Citation9] For those reasons, food researchers developed fats alternative to CB.[Citation10] Cocoa butter equivalents (CBEs) are vegetable fats.
They are not altering the physicochemical characteristics of CB because they are very similar to the CB in terms of composition and behavior.[Citation7] Commercial CBEs are blends of fats rich in 2-oleodipalmitin (POP) and 2-oleodistearine (SOS) triglycerides and need tempering, like CB.[Citation11] However, CBEs cost noticeably less and have a more uniform nature than CB.[Citation12] Cocoa butter improvers (CBIs) belong to the same group of CB analogues as well as CBEs. Compared to CBEs and CB, they have a higher content of solid triglycerides and they are used for improving soft CBs.[Citation13] Such vegetable fats are able to blend in CB in any proportion, but legally permitted level is up to 5% in the European Union (EU) for a product to be sold as chocolate.[Citation14] At certain temperatures these fats have a different solid fat content (SFC) values then CB, and their addition changes SFC values in chocolate mass, which have an effect on the physical properties of chocolate.[Citation15] Determination of SFC values at various temperatures is very useful when creating product’s physical attributes and predicting the behavior of the final product. Nuclear magnetic resonance (NMR) is the only technique that makes a direct measurement of SFC in fats and has become dominant technique for these determination.[Citation16]
Consumers acceptability depend on numerous factors, but for chocolate, unique sensory properties are perhaps the most important.[Citation9] Pleasant characteristics of flavor, appearance, aroma, and texture are very important for consumers.[Citation17] Three textural characteristics such as hardness, meltiness, and smoothness, play an important role in the sensory assessment of chocolate.[Citation18] Continuous lipid phase is very important for sensory properties.[Citation19] So, it is very important that CB analogues do not change the sensory characteristics of the product because negative changes in these characteristics of chocolate might cause potential aversive consumer reactions.[Citation20,Citation21]
Different sensory analysis methods can be used to investigate the effects of ingridients and processing variables on the final sensory quality of a product.[Citation22] There are many studies dedicated to sensory evaluation of different food products.[Citation23,Citation24] Descriptive techniques are frequently used as the most appropriate method for sensory evaluation. Descriptive sensory analysis enables us to obtain complete sensory description of products, and helps identifying underlying ingredient and process variables. These techniques can provide complete sensory description of products, determine how ingredient or process changes affect product characteristics, and identify key sensory attributes that promote product acceptance.[Citation25] Beside the quantitative descriptive analysis (QDA), a scoring method is a suitable procedure for the assessment of products’ sensory quality based on define quality level defining.[Citation26,Citation27]
The objective of this study was to examine the possibility of using different concentrations (3, 5, and 7%, calculated on chocolate mass) of two commercial CBEs as well as commercial CBI of different fatty acid compositions at three different temperatures of pre-crystallization (25, 27, and 29°C) for the laboratory chocolate production. Regarding this, the current investigation was focused on the influence of these vegetable fats and previously mentioned process parameters on sensory and textural properties of chocolates. Also, the article shows how the same amount of all three fats affect the change in the SFC values of chocolate at precryistallization temperatures.
MATERIAL AND METHODS
Material
CB sample—pressed CB of moderate hardness (Ghana)
Fats analogues to CB—commercial samples (Aarhus Karlshamn AB, Sweden):
Cocoa butter equivalent F1 – CBE F1
Cocoa butter equivalent F2 – CBE F2
Cocoa butter improver F3 – CBI F3
shows physical characteristics of the tested CB sample and of the CBEs (F1 and F2) and CBI (F3) samples.
TABLE 1 Physical properties of the cocoa butter (CB), cocoa butter equivalents (F1 and F2), and cocoa butter improver (F3)
Preparation and Pre-Crystallization Process of Chocolate Mass Samples
The ground samples of chocolate mass (34.55% fat content) were initially melted at the temperature of 70°C and after 30 min of mixing, the appropriate amount of CBEs was added. Therefore, the final products had 3, 5, and 7% of CBE. The content of fat in all tested samples was adjusted to the same level by the addition of CB and it was 39.13%. The process of pre-crystallization was performed in the laboratory pre-crystallizer—a modified Brabender farinograph. The process of pre-crystallization was controlled indirectly by the changes of the mass resistance on the occasion of mixing, which is registered on a force/time diagram—the thermorheogram. The applied pre-crystallization temperatures were: 25, 27, and 29°C.[Citation13]
The pre-crystallized chocolate mass samples were molded into plastic forms 80 mm × 80 mm × 8 mm in size, previously heated to the molding temperature (35°C). All molded samples were exposed to hardening conditions (controlled cooling to 20°C over 180 min) in order to avoid undesirable fat blooming. Samples of chocolate without the CBE addition were prepared at each applied temperature of pre-crystallization and used as the reference samples.
Fatty Acids Composition
Fatty acid composition was determined using gas chromatography–mass spectrometry (GC–MS) analysis. The methylation of fatty acid was carried out as described by Kravić et al.[Citation28] The analyses of fatty acid methyl esters (FAMEs) were performed on a Hewlett-Packard (HP) 5890 gas chromatograph coupled with a HP 5971A mass spectrometer detector. A fused silica capillary column (Supelco) was used for the FAMEs analysis; SP-2560, 100 m × 0.25 mm i.d., 0.20 μm film thickness. The carrier gas was helium with a constant flow rate of 0.62 cmCitation3/min. The GC oven temperature was programmed from 100°C (5 min), to 240°C at the rate of 6°C/min, held at 240°C for 10 min. The injection temperature was 230°C and the injection volume was 1.0 μL, split ratio 1:30. The mass spectrometer was operated in the electron ionization mode with ion source temperature of 180°C. Data acquisition was carried out in the scan mode (m/z range 40 to 400), solvent delay time was 12 min. The identification of FAMEs was based on mass spectra and retention time. Quantification of each individual FAME was performed following the procedure of AOAC (2000) using calculated response factor of FAME.[Citation29] Standard solution (37 component Mix, 47885-U, Supelco) was used for the calculation of the response factor.
Solidification Curve
The method of determining the solidification curve included measuring the temperature of fat during cooling from 40°C to the temeperature of complete solidification. The determination is carried out at cooling medium temperature of 0°C and the temperature of fat was read and recorded every minute. Obtained data was a base for plotted curve of temperature/time dependence. Solidification curves represent: Tmax, hardened point of CB (°C); Tmin, pre-crystallization temperature (°C); τmax, time of hardened (min); τmin, pre-crystallization time (min); ΔT/Δτ, temperature quotient which indirectly indicates the rate of crystallization.[Citation30]
Thermorheograms
The process of pre-crystallization was controlled indirectly by the changes of the mass resistance on the occasion of mixing, which was registered on a force/time diagram—the thermorheogram.
Characteristic values of thermorheographic curve are: τ1, nucleation time (min); τ2, time to the achieving the torque maximum (min); Mo, initial torque (Nm); Momax, torque maximum, (Nm); Mo°bl, torque of pre-crystallization chocolate mass (Nm).
SFC
Determination of SFC was performed by using the Bruker minispec 20 mq NMR. The curves of SFC in CB from Ghana and three tested fats were determined according to IUPAC 2.150.[Citation31] The curves of SFC in chocolate samples with the addition of CBE were compared to curves of SFC in referent chocolate samples. These results were presented in one diagram of SFC profiles of chocolate samples without CBEs and with addition of the same concentration of investigated CBEs and tempered at the same pre-crystallization temperature (25, 27, and 29°C). Samples preparation and measurement parameters were defined according to Petersson.[Citation32]
Texture Measurement
The hardness of products was measured using TA-XT Plus Texture Analyzer equipped with 3-Point Bending Rig HDP/3PB and a 50 kg load cell. Maximum force (from the force–distance graph expressed in N) needed to fracture chocolate through a sample (80 mm × 80 mm × 8 mm) was determined with three replications at a pre-speed of 1.0 mms, test of 3.0 mm/s, post-speed of 10.0 mm/s, distance 40 mm. Measurements were done at the temperature of 20°C, 7 days after chocolate stabilization.
Sensory Evaluation
Sensory testing of chocolate samples was conducted by panel of eight expert assessors with years of experience in chocolate production and with wide experience in QDA. All assessors were selected from academic staff of the Faculty of Technology, Novi Sad, aged between 30 and 45 years. Sensory evaluations were carried out in individual air-conditioned (22°C) sensory booths designed according to ISO 8589 standards.[Citation33]
The chocolate textural properties and overall chocolate quality were characterized throughout two methodologies. The QDA method[Citation34] provided a complete sensory description of experimental products. This method was used to identify the presence of particular chocolate textural properties and to define its intensity. For this purpose, besides experimental chocolate products, we purchased commercially available milk and dark chocolate products were used as well in order to cover wide range of textural specificities. The assessors had to write all possible terms that might be used to describe the textural properties that they individually perceived during consumption of samples. The panel leader collected all the descriptors and throughout a group discussion, all redundant, vague, and affective terms were eliminated from the list. The final list of the descriptors was established by multivariate statistical analyses. This technique enabled the estimation of importance and contribution of descriptors to differentiate between the samples. The descriptors with small contribution (lower than 0.5)[Citation35] to the first two principal components were removed from the further processing. In addition, the panel discussed the meaning of each descriptor from the final list and how to assess the products. During the following sessions the panel assessed the selected descriptors with respect to their intensities of different chocolate samples to achieve better understanding on how to measure all attributes on chosen scale. It was conducted in 10, 1-hour training sessions until the panel was homogeneous in assessments.
The descriptive attributes were evaluated by using 9-point category scale, where 1 refers to minimum and 9 refer to maximum intensity of appropriate attribute. Sensory attributes subjected to texture, their definitions, and scale extremes are given in . Scoring procedure was used to give an insight into overall chocolate quality. For each sample, assessors scored the appearance, structure and brake, chewiness, odor, and taste on category 1–5 point scale, where point 5 means “extremely desired quality,” point 4 “desired quality,” point 3 “medium quality,” point 2 “low quality,” and point 1 “very low quality.” As the importance of each sensory attribute on the overall quality of the chocolate is different, the weight of each one was discussed and defined by the panel (importance coefficients: for odor 0.6; for appearance, structure, and brake, and for chewiness 0.8 each; for taste 1.0). The overall quality score was calculated by applying a weighting factor to each parameter and chocolate samples were classified into quality categories: unacceptable (<2.60), acceptable (2.70–3.20), good (3.30–3.80), very good (3.90–4.40) and excellent (>4.5).[Citation27]
TABLE 2 Sensory attributes, scale extremes, and definitions used in descriptive sensory analysis of the chocolate samples by trained panel
Both, quality assessment and the QDA method were carried out as complete block experiment. Every assessor received two pieces of each chocolate sample. Samples were tempered to room temperature, cut into cubes 20 × 20 mm and delivered individually on a white plastic plate coded with three randomly chosen numbers. The order of a sample presentation was specified by the experiment design for sensory analysis with XLSTAT-MX. The assessors were instructed to clean their mouths between samples by rinsing with warm water.
Statistical Analysis
Sensory and instrumental data were analyzed using three-way analysis of variance (ANOVA) with the temperature, concentration, and fat type as main effects as well as the interaction between the main effects. The ANOVA was followed by Fisher’s least significant difference (LSD) test to identify statistical differences among samples at a 5% significance level. In order to discriminate chocolate samples based on the textural properties, the principal component analysis (PCA) was used. Statistical analyses were performed using XLSTAT system software.
RESULTS AND DISCUSSION
Fatty Acids Composition
CB from Ghana and all three used CB analogues showed differences in fatty acid composition (). The content of palmitic and stearic acid is equalized in CB and in fat F2. In the fat F1 dominates palmitic, while in the fat F3 dominates stearic acid in total saturated fatty acids.
TABLE 3 Fatty acid composition of cocoa butter (CB), cocoa butter equivalents (F1 and F2), and cocoa butter improver (F3)
Solidification Curve
Based on values from the curve () CBE F1 had a longer pre-crystallization time, a lower rate of solidification, and the least expressed polymorphic properties in relation to the other fats. CBE F2 has a shorter pre-crystallization time, a shorter total time of solidification, and a lower rate of solidification compared to the CB. In comparison to the other two tested fats, CBE F2 was most similar to CB from Ghana regarding its crystallization properties. Both CBEs have less ΔT and ΔT/Δτ values then CB and twice lower rate of pre-crystallization which indicates weaker expressed polymorphism. CBI F3 had largest share of stearic and the smallest share of palmitic acid compared to other tested fats (), so it hardens faster as can be seen from the highest values of the temperature quotient.
Thermorheogram Parameters
The value of nucleation time (τ1) of chocolate mass
The torque value is a criterion for the viscous behavior of the chocolate mass and it depends on the crystallization extent of the chocolate mass. The results showed that nucleation time of the chocolate mass primarily depended on cooling temperature (, , and ). At a temperature of 25°C nucleation time did not change. At 27°C, the time was shortened by increasing concentration of all three fats, at 29°C was shortened slightly by increasing the concentration of CBE F1, it was shortened more by increasing concentration of CBE F2, while addition of CBI F3 meaningly reduced the nucleation time of the chocolate mass. This showed that all three fats under certain conditions affected the change of crystallization characteristics of CB, and the most pronounced changes were at 29°C. The reduction of nucleation time is desirable characteristic from the standpoint of production process of chocolate.
The value of maximum torque (Momax) of chocolate mass
At all three pre-crystallization temperatures Momax decreased proportionally to the increase of the concentration of fat CBE F1 (, , and ). Fat CBE F2 practically did not change Momax values of the chocolate at all three pre-crystallization temperatures. At 25 and 29°C the addition of fat CBI F3 increased Momax proportionally to the concentration of fat, while at 27°C led to decreasing of Momax. At 29°C fat CBE F1 caused the lowest Momax change compared to the fats CBE F2 and CBI F3. Sublimating obtained results from thermorheogram, it is obvious that a number of combinations of the final products with desired characteristics can be created by combination of indicators—nucleation time and torque maximum.
SFC
The percentage of liquid and solid phase at a certain temperatures was measured covering the interval between ambience temperature and body temperature. CB has a characteristic melting profile. At 10–20°C SFC is almost constant at 80–90%, depending on the composition and origin. The melting starts at 25–28°C and is almost complete at 32–35°C. Residual solid fat is easily detected by the tongue and as low as 2–3% solid fat can be perceived as a waxy, fatty note. SFC values at certain temperatures have shown the properties of chocolate (hardness, stability when heated, waxy taste, cooling sensation in the mouth, melting intensity when eating).[Citation3,Citation16] SFC values below 25°C show hardness of product, while values between 25 and 30°C represent the resistance to heating. In the temperature interval from 27 to 33°C mainly melting occurs, creating a cooling sensation in the mouth and flavor releasing. High SFC values at 20°C represent brittle and hard chocolate, and high values at 30°C mean that product is resistant to heating. A short melting interval means that solid fat becomes liquid after a certain, small increase of the temperature, and results in a cooling sensation when this phenomenon occurs at a few degrees below body temperature. Fats with melting point above body temperature (high SFC values at temperatures above 35°C) cause a waxy taste when eating the chocolate.
SFC of F1 was lower than CB SFC, except in the temperature interval from 35 to 40°C (). Comparing to CB, CBE F2 had smaller values of SFC in temperature interval from 10 to 30°C, and higher values in temperature range 30 to 40°C.[Citation13] According to the measurements, SFC values of CBI F3 were higher than SFC values of CB from Ghana in the whole temperature range. Influence of addition of fats CBE F1 and CBE F2 on physical characteristics of chocolate was shown in the earlier publications.[Citation13] However, in this article, that influence was examined from different point-of-view. The influence of the same amount of all three fats on the comparative diagrams was observed. Also, it was shown how the same amount of all three fats affects the change of SFC values of chocolate at pre-crystallization temperatures 25, 27, and 29°C (, , and ).
FIGURE 2 (A–C) SFC curves of chocolates depending on precrystallization temperature and concentration of two CBEs and CBI.
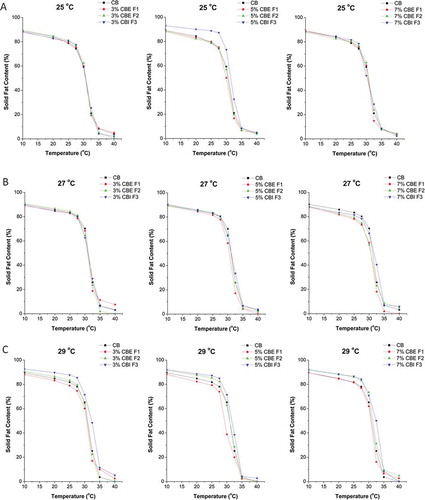
The amount of fats in concentration of 3% at pre-crystallization temperatures 25 and 27°C changed SFC values in temperature range 32.5 to 40°C, and had influence on cooling sensation in the mouth and waxy taste ( and ). At pre-crystallization temperature of 29°C all three fats had different influence on SFC values throughout the measuring temperature range in accordance with the influence on CB, except in temperature interval 35–40°C, when practically they did not change these values, i.e., did not affect the change in intensity of waxy taste (). The addition of fat CBE F1 resulted in the decrease of SFC values, fat CBE F2 did not change these values, while addition of fat CBI F3 increased SFC values of chocolate, which was reflected on a reduction, respectively, on an increase in hardness of chocolate (20–25°C). This is in accordance with the proportion of stearic and palmitic acids in total fatty acid content, as mentioned earlier ().
At a concentration of 5% at a pre-crystallization temperature of 25°C, the addition of fat CBI F3 increased SFC values in temperature range 10–35°C, which affected the hardness of chocolate, intensity of melting in the mouth and resistance to heating. At pre-crystallization temperature of 27°C, SFC values changed in the temperature range 30–32.5°C and had an effect on intensity of cooling sensation in the mouth. At 29°C only the addition of fat F1 decreases SFC values of chocolate. CBE F2 addition at concentration of 5% did not cause major changes compared to the control samples (chocolate without CBE or CBI addition) with exception of SFC increase in temperature range 20–27.5°C at pre-crystallization temperature of 29°C.
In this work, the addition of 7% CBE and CBI was investigated too, although according to the current European Directive (2003) the permitted maximum is 5% based on the chocolate mass. In available scientific literature there are no data about the influence of CBE/CBI addition on the chocolate quality in amounts greater than the permitted (5%) by the EU Directive (2003).[Citation36] Referring to this, the authors wanted to examine whether the limitations in terms of addition of higher amounts of CB analogues are justified from a technological point-of-view. At this concentration of fats, minimum changes of SFC values occurred at pre-crystallization temperature of 25°C—i.e., they had insignificant effect on the hardness of chocolate. At 27°C SFC values were different throughout the measuring temperature range, while at 29°C SFC values of control sample (chocolate sample without any CBE/CBI) and sample of chocolate with fat CBE F1 were practically matched in temperature range 10 to 27.5°C, which means that fat CBE F1 did not change the hardness, resistance to heating, and cooling sensation in the mouth when eating chocolate ( and ). Furthermore, the addition of 7% of CBE F2 and CBI F3, at pre-crystallization temperature of 25°C the SFC values were not changed, at pre-crystallization temperature of 27°C SFC was decreased while at pre-crystallization temperature of 29°C SFC was increased.
Sensory Analysis
Overall sensory quality was defined by appearance, structure and brake, chewiness, odor, and taste. The parameter which contributes the most to the overall quality was taste, as recommended by Dürrschmid et al.,[Citation37] while the other parameters had almost the same contribution. Thus, the overall quality score for all evaluated chocolate was in the range of 4.95 to 3.10 ().
FIGURE 3 (A, B) Overall sensory quality of chocolate samples and influence of main factors on overall sensory quality of chocolate samples.
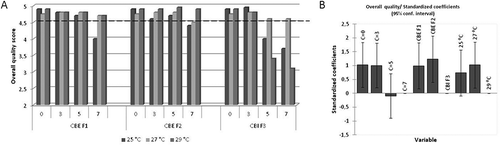
Obtained results for overall sensory quality indicated that the use of the CB equivalents F1 and F2 in chocolate formulation is in general acceptable without producing a negative impact on the sensory quality. All chocolate made with these equivalents was evaluated with excellent and very good sensory quality. It should be emphasized that the use of higher concentration of these equivalents is limited with pre-crystallization temperatures, whereas the chocolate prepared at 25°C had lower sensory quality for both types of equivalents. Based on these results, it can be concluded that the production of the chocolate with a higher content of equivalent than the legally permissible amount of 5% is justified without malformation of the sensory quality of the final product.
While those mentioned equivalents could be used in all tested concentrations, in terms of excellent sensory quality, the CB improver F3 could be used only at the concentration of 3%. These results are fully expected considering the fact that our chocolate formulations were without milk and this improver is precisely designed for the production of chocolate made from soft CB and with high milk content. In order to achieve the same sensory quality at higher concentration (5 and 7%) of F3 it is necessary to perform pre-crystallization at 27°C.
Results of the ANOVA for the scoring data are summarized in . These results show the significant influence of every single main factor on overall sensory quality of chocolate samples (concentration: F(3, 36) = 72.2, p = 0.000; additive: F(2, 36) = 55.6, p = 0.000; temperature: F(2, 36) = 9.5, p = 0.001), wherein the highest influence showed concentration. Furthermore, the results showed that there was statistically significant three-way interaction between concentration, additive type, and temperature (F(12, 36) = 17.3, p = 0.000). A three-way interaction can be explained in terms of two-way interactions wherein a difference in the simple main effects of one of the variables is explained as you change levels of the other variable. If results of two-way interactions are analyzed (concentration × additive: F(6, 36) = 27.0, p = 0.000; additive × temperature: F(4, 36) = 44.9, p = 0.000; concentration temperature: F(6, 36) = 9.5, p = 0.000) it can be concluded that they can explain more variation of data than interaction of all three factors, and the most important is interaction between temperature and CB analogue. As we concluded earlier, CBEs F1 and F2 are more stable at all applied temperatures and provide better quality of chocolate than CBI F3.
In order to observe the differences in the textural properties of chocolate samples the PCA was applied ( and ). The results were presented in loading and score plots. A PCA was performed on original sensory data (data are not standardized), since intensity of descriptors measurements were performed on the same scale (9-point category scale). Number of PCs was determined on the basis of the relevant information they provided.[Citation38]
FIGURE 4 (A, B) PCA score and loading plots of chocolate samples distinction based on the textural properties.
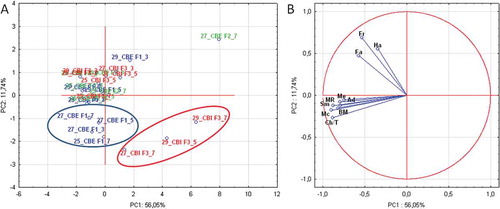
The first two principal components explained about 70% of the variance in the data matrix values. PC1 was strongly related to the adhesiveness, chewiness, smoothness, melting, beginning of melting, and melting rate, while PC2 was strongly related only to the hardness, fracturability, and fatness. The score plot showed clear distinction in terms of evaluated textural parameters of investigated analogue fats and processing parameters. Generally, the most similar textural profile to the control samples (chocolate without CBE or CBI addition) possessed chocolates with addition of CBE F2, regardless of the concentration level and pre-crystallization temperature. These samples possessed the most uniform textural profile which is reflected in high level of hardness and fracturability together with short time necessary for beginning of melting and high ability to quickly become a semiliquid. Addition of CBE F1 at all concentrations (3, 5, and 7%) in chocolate masses prepared at 29°C had a similar textural profile to chocolates with added CBE F2. Furthermore, for this cocoa analogue pre-crystallization temperature of 27°C was not adequate since it influenced reduction of chocolate hardness. On the other hand, addition of CBE F3 at higher concentration (5 and 7%) in chocolate masses prepared at 29°C had a significant negative effect (p < 0.05) on the textural profile and these samples were with the lowest hardness and fracturability followed by prolonged beginning of melting and longer melting rate. Taking into account the results of sensory quality evaluation, this textural profile could be considered as inappropriate. The absence of the expected enhancing effect of a CB improver was probably because of the usage of inadequate CB.
Texture Measurement
Hardness plays an important role in quality assessment of final products and present force necessary to attain a given deformation. For chocolate, hardness was influenced by several factors including composition, its processing technique, and most of all by tempering.[Citation39] Therefore, hardness can be used as an indicator of a good tempering process.[Citation40] In the current study, the ANOVA results for hardness revealed that the effect of the CBE type was significant (p = 0.017) unlike the effect of the pre-crystallization temperature. The interaction of these two main factors was significant (p = 0.039) as well ().
FIGURE 5 Effect of cocoa butter equivalent/improver, precrystallization temperature, and cocoa butter equivalent/improver concentration on hardness of chocolate.
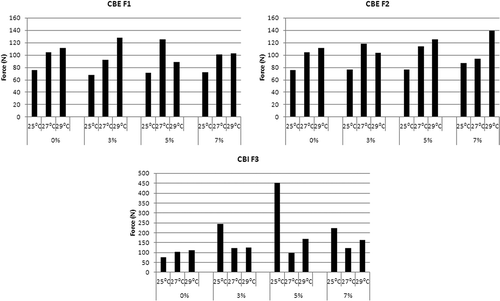
Instrumental texture measurements of chocolate samples showed that the hardness of control and samples with addition of CBEs F1 and F2 rise with the increase of the pre-crystallization temperatures. However, this trend was different for the samples with 5% of F1 and 3% of F2 tempered at 29°C, which had lower values of hardness, in comparison with those prepared at 27°C. In general, chocolate samples with addition of these types of CBEs had slightly lower hardness compared to the control samples, although it was not statistically significant (p > 0.05). These findings were in agreement with the previous results reported by Torbica et al.[Citation16] who found that the usage of the CBE (F1) had softening effect on CB. Unlike them, the addition of the CBI F3 led to the significantly (p < 0.05) harder chocolate. This increase of hardness was more noticeable for the samples tempered at 25°C, and with addition of 5% of this equivalent. Taking into account the low concentration of the CBI (F3), there is a possibility that these differences were caused by ratio of amount of stearic acid/palmitic acid which for CB is 1.12, for CBE F1 0.49, for CBE F2 1.14, and for CBI F3 1.49 () and, therefore, these samples had apparently higher values of hardness.
CONCLUSIONS
On the basis of the thermorheograms, the nucleation time of the chocolate mass primarly depended of pre-crystallization temperature while the value of maximum torque (Momax) of chocolate mass were influenced by both, pre-crystallization temperatures, and concentration of fats. Determination of crystallization behavior showed that SFC was also conditioned by pre-crystallization temperatures and concentration of fats to a different extent. The results of sensory evaluation showed that CB equivalents F1 and F2 in general can be used in chocolate formulation without producing a negative impact on the sensory quality since all chocolate made with these equivalents was in the range of excellent and very good quality. However, in order to achieve the acceptable sensory quality of chocolate with CBI F3, it is necessary to use lower concentration (up to 3%) or to perform the pre-crystallization process at 27°C, or to use softer CB and addition of milk, or a higher content of milk (milk powder). The findings of this study in terms of evaluated textural parameters indicated that the most uniform results were obtained when the chocolate mass was tempered at 25°C. Furthermore, the results of the instrumentally measured hardness revealed that addition of CBEs F1 and F2 did not significantly (p > 0.05) affect the hardness of chocolate samples, in contrast to the CBI F3 whose addition led to the significantly (p < 0.05) harder chocolate.
FUNDING
This article is a result of the research within the project TR 31014 financed by the Ministry of Education, Science, and Technological Development, Republic of Serbia.
Additional information
Funding
REFERENCES
- Bootello, M.A.; Hartel, R.W.; Garcés, R.; Martínez-Force, E.; Salas, J.J. Evaluation of High Oleic-High Stearic Sunflower Hard Stearins for Cocoa Butter Equivalent Formulation. Food Chemistry 2012, 134, 1409–1417.
- Çiftçi, O.N.; Fadıloğlu, S.; Göğüs, F. Conversion of Olive Pomace Oil to Cocoa Butter-Like Fat in a Packed-Bed Enzyme Reactor. Bioresource Technology 2009, 100, 324–329.
- Lidefelt, J.O. (Ed.) Handbook: Vegetable Oils and Fats. Karlshamn, Sweden: Karlshamns AB, 2002.
- Svenstrup, G.; Heimdal, H.; Nørgaard, L. Rapid Instrumental Methods and Chemometrics for the Determination of Pre-Crystallization In Chocolate. International Journal of Food Science Technology 2005, 40, 953–962.
- Afoakwa, O.E.; Paterson, A.; Fowler, M. Factors Influencing Rheological and Textural Qualities in Chocolate—A Review. Trends Food Science Technology 2007, 18, 290–298.
- Wang, H.-X.; Wu, H.; Ho, C.-T.; Weng, X.-C. Cocoa Butter Equivalent from Enzymatic Interesterification of Tea Seed Oil and Fatty Acid Methyl Ester. Food Chemistry 2006, 97, 661–665.
- Shekarchizadeh, H.; Kadivar, M.; Ghaziaskara, H.S.; Rezayat, M. Optimization of Enzymaticsynthesis of Cocoa Butter Analog from Camel Hump Fat in Supercritical Carbon Dioxide by Response Surface Method (RSM). Journal of Supercritical Fluids 2009, 49, 209–215.
- Chalseri, S.; Dimick, P.S. Cocoa Butter—Its Composition and Properties. The Manufacturing Confectioner 1987, 9, 115–122.
- Afoakwa, E.O. Chocolate Production and Consumption Patterns. In Chocolate Science and Technology; Afoakwa, E.O.; Ed.; Wiley & Blackwell: West Sussex, UK, 2010; pp 1–10.
- Lipp, M.; Anklam, E. Review of Cocoa Butter and Alternative Fats for Use in Chocolate. Food Chemistry 1998, 62, 73–99.
- Kang, K.K.; Kim, S.; Kim, I.H.; Lee, C.; Kim, B.H. Selective Enrichment of Symmetric Monounsaturated Triacylglycerols from Palm Stearin by Double Solvent Fractionation. Food Science and Technology 2013, 51, 242–252.
- Cidell, J.L.; Alberts, H.C. Constructing Quality: The Multinational Histories of Chocolate. Geoforum 2006, 37, 999–1007.
- Torbica, A.; Pajin, B.; Omorjan, R. Influence of Soft Cocoa Butter Equivalents on Color and Other Physical Attributes of Chocolate. Journal of the American Oil Chemists Society 2011, 88, 937–947.
- FDA. Cocoa and Chocolate Products Regulations Cocoa and Chocolate Products Regulations; Food Standard Agency: UK, 2003.
- Ačkar, Đ.; Škrabal, S.; Šubarić, D.; Babić, J.; Miličević, B.; Jozinović, A. Rheological Properties of Milk Chocolates As Influenced by Milk Powder Type, Emulsifier, and Cocoa Butter Equivalent Additions. International Journal of Food Properties 2015, 18, 1568–1574.
- Torbica, A.; Jovanovic, O.; Pajin, B. The Advantages of Solid Fat Content Determination in Cocoa Butter and Cocoa Butter Equivalents by the Karlshamns Method. European Food Research and Technology 2006, 222, 385–392.
- Cardoso, J.M.P.; Bolini, H.M.A. Descriptive Profile of Peach Nectar Sweetened with Sucrose and Different Sweeteners. Journal of Sensory Studies 2008, 23, 804–816.
- Viane, J.; Januszewska, R. Quality Function Deployment in the Chocolate Industry. Food Quality and Preference 1999, 10, 377–385.
- El-Kalyoubi, M.; Khallaf, M.F.; Abdelrashid, A.; Mostafa, E.M. Quality Characteristics of Chocolate: Containing Some Fat Replacer. Annals of Agricultural Sciences 2011, 56, 89–96.
- Melo, L.L.M.M.; Bolini, H.M.A.; Efraim, P. Sensory Profile, Acceptability, and Their Relationship for Diabetic/Reduced Calorie Chocolates. Food Quality and Preference 2009, 20, 138–143.
- Ares, G.; Barreiro, C.; Deliza, R.; Giménez, A.; Gámbaro, A. Consumer Expectations and Perception of Chocolate Milk Desserts Enriched with Antioxidants. Journal of Sensory Studies 2010, 25, 243–260.
- Murray, J.M.; Delahunty, C.M.; Baxter, I.A. Descriptive Sensory Analysis: Past, Present, and Future. Food Research International 2001, 34, 461–471.
- Belščak-Cvitanović, A.; Komes, D.; Benković, M.; Karlović, S.; Hečimović, I.; Ježek, D.; Bauman, I. Innovative Formulations of Chocolates Enriched with Plant Polyphenols from Rubus Idaeus L. Leaves and Characterization of Their Physical, Bioactive, and Sensory Properties. Food Research International 2012, 48, 820–830.
- Lenfant, F.; Hartmann, C.; Watzke, B.; Breton, O.; Loret, C.; Martin, N. Impact of the Shape on Sensory Properties of Individual Dark Chocolate Pieces. Food Science and Technology 2013, 51, 545–552.
- Phang, Y.L.; Chan, H.K. Sensory Descriptive Analysis and Consumer Acceptability of Original “Kaya” and “Kaya” Partially Substituted with Inulin. International Food Research Journal 2009, 16, 483–492.
- Etaio, I.; Albisu, M.; Ojeda, M.; Gil, P.F.; Salmerón, J.; Pérez Elortondo, F.J. Sensory Quality Control for Food Certification: A Case Study on Wine. Method Development. Food Control 2010, 21, 533–541.
- Popov-Raljić, J.; Laličić-Petronijević, J. Sensory Properties and Color Measurements of Dietary Chocolates with Different Compositions During Storage for up to 360 Days. Sensors 2009, 9, 1996–2016.
- Kravić, S.; Marjanović, N.; Suturović, Z.; Švarc-Gajić, J.; Stojanović, Z.; Pucarević, M. Determination of Trans Fatty Acid Content of Serbian Shortening by Gas Chromatography–Mass Spectrometry. Acta Aliment Hung 2010, 39, 413–423.
- AOAC. Official Method 963.22. Methyl Esters of Fatty Acids in Oils and Fats; AOAC: Washington, DC, 2000.
- IOCCC. Analytical Method 110. Determination of the Cooling/Solidification Curve of Cocoa Butter and of Other Fats Used in Chocolate and Confectionery Products; IOCCC—International Office of Cocoa, Chocolate, and Sugar Confectionery: Brussels, Belgium, 1998.
- IUPAC Standard Methods for the Analysis of Oils, Fats, and Derivatives 2.150. Solid Content Determination in Fats by NMR (Low Resolution Nuclear Magnetic Resonance), 7thEd. ; Blackwell Scientific: Oxford, United Kingdom, 1992.
- Petersson, B. Pulsed NMR Method for Solid Fat Content Determination in Tempering Fats, Part II: Cocoa Butters and Equivalents in Blends with Milk Fat. Fett Wiss Technol 1986, 4, 128–136.
- ISO 8589 Sensory Analysis. General Guidance for the Design of Test Rooms; ISO—International Organization for Standardization: Geneva, Switzerland, 1988.
- Stone, H.; Bleibaum, R.; Thomas, H.A. Descriptive Analysis (Chapter 6). In Sensory Evaluation Practices; Stone, H.; Bleibaum, R.; Thomas, H.A., Eds.; 4th ed. Elsevier Academic Press: San Diego, CA, 2012; 233–289 pp.
- Bower, J.A. Principal Component Analysis. In Statistical Methods for Food Science: Introductory Procedures for the Food Practitioner. Wiley-Blackwell Ltd.: Oxford, United Kingdom, 2009.
- European Communities (Marketing of Cocoa and Chocolate Products) Regulations; S.I. No. 236/2003, 2003.
- Dürrschmid, K.; Albrecht, U.; Schleining, G.; Kneifel, W. Sensory Evaluation of Milk Chocolates As An Instrument of New Product Development. In Food is Life; Proceedings of the World Congress of Food Science & Technology, 2006; IUFoST: Nantes, 2006.
- Pestorić, M.; Šimurina, O.; Filipčev, B.; Jambrec, D.; Belović, M.; Mišan, A.; Nedeljković, N. Relationship of Physicochemical Characteristics with Sensory Profile of Cookies Enriched with Medicinal Herbs. International Journal of Food Properties 2015, DOI:10.1080/10942912.2015.1004586.
- Afoakwa, E.O.; Paterson, A.; Fowler, M.; Vieira, J. Effects of Tempering and Fat Crystallisation Behaviour on Microstructure, Mechanical Properties, and Appearance in Dark Chocolate Systems. Journal of Food Engineering 2008, 89, 128–136.
- Keogh, K.; Twomey, M.; O’Kennedy, B.; Mulvihill, D. Effect of Milk Composition on Spray-Dried High-Fat Milk Powders and Their Use in Chocolate. Lait 2002, 82, 531–539.