Abstract
Fruits of Sonneratia apetala (Buch.-Ham.) are widely used as food and in treating various diseases in the tropical coastal areas. This study evaluated nutrient compositions in the pericarp and seed of this fruit. Each pericarp and seed was successively fractionated into n-hexane, diethyl ether, chloroform, ethyl acetate, and methanol. Polyphenols contents and antioxidant activities of different pericarp and seed fractions were measured in different in vitro methods and phenolic compounds were determined by HPLC. Carbohydrates, proteins, lipids, and ash contents were 29.6, 8.8, 2.8, and 25.5% of dry weight in pericarp, whereas 28.3, 11.5, 4.2, and 22.7% in seed, respectively. Among the mineral macro-elements, K content was the highest followed by Na, Ca, Mg, P, and S while in micro-elements, Fe was at the largest followed by Mn, Zn, and Cu. The methanol fraction of seed showed the highest polyphenols content (221.9 mg gallic acid equivalent/g fraction), 1,1-diphenyl-2-picrylhydrazyl (DPPH) (IC50 = 2.1 μg/mL) and NO (IC50 = 490.8 μg/mL) free radical scavenging. Similarly, methanol fraction of seed also attained very strong reducing power (OD = 1.67 at 100 µg/mL), Fe2+ chelating and total antioxidant capacity. When subjected to high-performance liquid chromatogram analysis, six polyphenols namely caffeic acid, (+)-catechin, (-)-epicatechin, ellagic acid, gallic acid, and quercetin were detected and quantified as 88.1, 1459.3, 310.1, 616.9, 416.7, and 71.8 mg/100 g of methanol fraction of seed, respectively. Therefore, the fruit of S. apetala, especially its seeds could be of great use in preparation of functional foods and dietary supplements.
Introduction
Phytochemicals are plant originated bioactive components that have beneficial effects on human body in preventing or treating diseases or promoting physiological health. In fact, many of the diseases affecting human beings are the result of lacking phytonutrients in their diet. Among the dietary intake of phytochemicals, polyphenols are at the top, and more than 5000 polyphenolics have already been identified. They show high redox potentials and act as important antioxidants, reducing agents, hydrogen donors, singlet oxygen quenchers, and metal chelators resulting in protection against pathogenesis of various metabolic and infectious diseases.[Citation1] Various epidemiological studies have suggested that consumption of fruits and vegetables is associated with reduced risk of cardiovascular diseases and cancer,[Citation2] neurodegenerative diseases, such as Parkinson’s and Alzheimer’s diseases,[Citation3] as well as inflammation and aging.[Citation4] Activity in erythrocyte plasma membrane redox system was also reported to have increased when rats were fed with pomegranate peel extract.[Citation5] In previous studies, we reported polyphenols contents and beneficial effects of edible fruits,[Citation6] antidiabetic plants,[Citation7,Citation8] fruity, and leafy vegetables.[Citation9,Citation10] We also reported antihyperglycemic effects of (+)-catechins (CH).[Citation11,Citation12] However, various surveys show that about 7000 plants species across the world are cultivated or harvested apart from the wild for food production.[Citation13] Many plants used as ethnic foods are getting attention currently for food security, income generation, maintaining health, and prevention of adverse effects of climate change.
The mangrove fruit Sonneratia apetala (Buch.-Ham.), locally known as keora, is abundantly grown in the world largest mangrove forest, the Sundarbans and also along the coastal areas in Bangladesh, India, Myanmar, Malaysia, China, Papua New Guinea, and some parts of Africa. People in coastal Bangladesh, India, and Myanmar extensively consume the fruit by cooking and through other preparations. The ripe fruits are eaten by people from Africa to the Malayas and Javanese, and are said to taste like cheese. In many regions, the fruits are also processed to produce sour sauce which is marketed. Fermented juice of this fruit is useful in arresting haemorrhage. Fruits and barks of the plants belonging to genus Sonneratia have remedial activities against asthma, febrifuge, ulcers, swellings, sprains, bleeding, hemorrhages, and piles.[Citation14] Patra et al.[Citation15] reported antibacterial, antioxidant, antidiabetic, and anticancer activities of leaf and bark extracts of S. apetala. Nevertheless, a little scientific attention on the fruit has been paid until today. We reported antioxidant, antidiabetic, and antibacterial activities of methanol extract of this fruit.[Citation16] Moreover, analgesic, antidiarrhoeal, anthelmintic, and cytotoxic activities of ethanol extract of the fruit were also reported.[Citation17] However, food insecurity is one of the major causes of hunger and malnutrition in tropical coastal population. To fulfill food security and primary health care, it is essential to explore wild food plants and for the tropical coastal countries, edible mangrove fruits could be an attractive source in this regard since they could easily be planted in vast saline water intruded areas outside the coastal embankments. Fruit of S. apetala is such an important fruit as has untapped potential and, therefore, the current study was undertaken.
Materials and Methods
Materials
Chemicals and reagents
1,1-Diphenyl-2-picrylhydrazyl (DPPH) was purchased from Wako Pure Chemical Industry, Ltd. (Osaka, Japan). Ascorbic acid (AA), bovine serum albumin, caffeic acid (CA), (+)-catechin hydrate (CH), p-coumaric acid (PCA), (-)-epicatechin (EC), ellagic acid (EA), ferrozine, Folin-Ciocalteu’s phenol reagent, gallic acid (GA), rutin hydrate (RH), quercetin (QU), and vanillic acid (VA) were purchased from Sigma-Aldrich Co (St. Louis, MO, USA). n-Hexane, diethyl ether, chloroform, ethyl acetate, methanol, dimethyl sulfoxide (DMSO), HNO3, HClO4, acetic acid, H2SO4, phenol, HCl, high-performance liquid chromatography (HPLC) grade acetonitrile, methanol, acetic acid, and ethanol were obtained from Merck (Darmstadt, Germany).
Plant material
The fruits of Sonneratia apetala (Buch.-Ham.) were collected from the world’s largest mangrove forest, the Sundarbans. After the collection, the pericarps and seeds of the fruits were separated and shed dried. Then, each dried sample was ground by a grinding machine into powdered form and stored in an air-tight container.
Methods
Extraction
Ten grams (10 g) of powder of each type (pericarp and seed) was taken in separate air-tight container. They were then extracted by adding 200 mL of 100% n-hexane to each on vigorouly shaking the mixtures for 30 min. Hereafter, the mixtures were filtered through Whatman filter paper no. 1. The filtrates were air-dried and the extracts were stored in a refrigerator as the n-hexane fraction labeled discretely for the pericarp and the seed. Similarly, diethyl ether, chloroform, ethyl acetate, and methanol fractions were also successively prepared following the same procedure using the residues on the filter paper. The n-hexane, diethyl ether, chloroform, ethyl acetate, and methanol fractions of pericarp of S. apetala were designated as hexane fraction of pericarp (HP), diethyl ethyl ether fraction of pericarp (DP), chloroform fraction of pericarp (CP), ethyl acetate fraction of pericarp (EP), and methanol fraction of pericarp (MP) while those for seed were marked as hexane fraction of seed (HS), diethyl ether fraction of seed (DS), chloroform fraction of seed (CS), ethyl acetate fraction of seed (ES), and methanol fraction of seed (MS), respectively. Finally 10 mg of the solid was dissolved in 1 mL DMSO (10 mg/mL) to conduct the experiments.
Determination of nutritional compositions
Moisture contents in fresh pericarp and seed were determined by oven drying at 105°C for 5 h until a constant weight was obtained subtracting the dry weight (d.w.) values from the wet weights. Carbohydrate contents in dried powder of pericarps and seeds were determined by the phenolic sulphuric acid colorimetric method using glucose as a standard.[Citation18] Protein contents were calculated by the Lowry method.[Citation19] Total lipids were determined by extracting the powders with chloroform:methanol (1:2) as described by Bligh and Dyer.[Citation20] Total ash contents were determined by calcination in a muffle furnace at 550°C for 8 h until a constant weight was attained. When residues of black unincinerated carbon material remained after pre-incineration, ion-exchange-resin-purified water was added to dissolve them, and incineration was continued.
The percent calories in both of pericarp and seed were determined by multiplying the percentage of crude protein and carbohydrate with four and crude fat with nine. The values were then converted to calories per 100 g of pericarps and seeds.[Citation21] Mineral contents in pericarp and seed were estimated as described by Hoenig and de Kersabiec[Citation22] with slight modification. Concentrations of calcium (Ca), copper (Cu), iron (Fe), magnesium (Mg), manganese (Mn), and zinc (Zn) were determined by flame atomic absorption spectrophotometry (AAS). Each of the dried powder of pericarp and seed (1 g) was taken in 50 mL flask and added 15 mL of mixed acid solution of nitric acid (HNO3) and perchloric acid (HClO4) as a ration of 2:1. They were then heated in acid solution in a fume hood (Esco Frontier Acid Digestion, Singapure) on a hot plate (model VWR VELP Scientifical, Germany). Generation of white fumes from the flasks indicated the completion of digestion and the flasks were allowed to cool. These digested samples were transferred into 100 mL volumetric flasks and the volume was adjusted to 100 mL by adding distilled water. Then the extract was filtered with filter paper (Whatman no. 42) and the filtrate was collected in labeled plastic bottles. The solutions were analyzed for the content of elements using an atomic absorption spectrophotometer (Shimadzu AA-7000, Japan) with suitable hollow cathode lamps. The concentrations of different elements in pericarp and seed were determined by the corresponding standard calibration curves obtained by using standard analytical reagent grade solutions of the elements, Ca, Cu, Fe, Mg, Mn, and Zn. A 0.5 M chloride solution containing 20% trichloroacetic acid and 10% lanthanum chloride (w/v) was added to the sample used for Ca measurement to prevent interference by coexisting elements. A 0.5 M chloride solution containing 10% lanthanum chloride was added to the sample used for Mg measurement.
Digested powders of pericarp and seed were used to determine the concentration of sodium (Na) and potassium (K) by using flame photometer. Phosphorus (P) and sulphur (S) contents in dried powders of pericarps and seeds were quantified by ultra violet (UV)-visible spectrophotometric method. Phosphorus content was measured by phosphovanadomolybdate yellow color method in nitric acid system at 420 nm wavelength using by double beam UV-visible spectrophotometer (Model 205, Jena, Germany). Sulphur content was measured by turbidometric method with BaCl2 and tween-80 reagent at 420 nm wavelength.
Determination of total polyphenols (TPH)
The total concentration of phenolics (TPH) in the fractions was determined according to the Folin-Ciocalteu method[Citation23] with GA as the standard and expressed (mg) as gallic acid equivalents (GAE)/g of fraction. One milliliter (1 mL) of diluted fraction was mixed with 1 mL of Folin-Ciocalteu’s reagent and vortexed for 5 s. Then, 1 mL of a 10% (w/v) sodium carbonate aqueous solution was added to the mixture. The mixture was incubated at room temperature for 1 h, after which colorimetric measurements were made at 700 nm. Each experiment was conducted three times.
DPPH free radical scavenging activity
The reaction mixture (total volume, 3 mL), consisting of 0.5 mL of a 0.5 M acetic acid buffer solution at pH 5.5, 1 mL of 0.2 mM DPPH in ethanol, and 1.5 mL of a 50% (v/v) ethanol aqueous solution, was shaken vigorously with the fraction.[Citation24] After incubation at room temperature for 30 min, the amount of DPPH remaining was determined by measuring absorbance at 517 nm. Mean values were obtained from triplicate experiments.
NO free radical scavenging activity
The scavenging effect of the fractions on nitric oxide was measured according to the slightly modified method of Marcocci et al.[Citation25] Four milliliters of fraction solutions of different concentrations were then added in the test tubes to 1 mL sodium nitroprusside solution (10 mM), and the test tubes were incubated at 37°C for 3 h. The same reaction mixture, without the fraction but with an equivalent amount of DMSO, served as control. An aliquot (0.5 mL) of the incubation solution was removed, diluted with 0.5 mL Griess reagent (1% sulfanilamide in 5% H3PO4 and 0.1% naphthylethylenediamine dihydrochloride) and the absorbance of the chromophore was immediately read at 570 nm.
Reducing power capacity
The reducing power of the fractions was determined according to the method of Oyaizu.[Citation26] Briefly, different concentrations of the fractions were mixed with 2.5 mL of 0.2 M phosphate buffer, pH 6.6 and 2.5 mL of 1% potassium ferricyanide solution. After incubation at 50°C for 20 min, the mixtures were mixed with 2.5 mL of 10% trichloroacetic acid followed by centrifugation at 650 g for 10 min. The supernatant (2.5 mL) was mixed with 2.5 mL of distilled water and 0.5 mL of 0.1% ferric chloride. The absorbance of this solution was measured at 700 nm. AA served as positive control.
Ferrous (Fe2+) ion chelating activity
The chelating activity of different fractions of pericarp and seed, on Fe2+ was measured according to the method of Carter.[Citation27] Briefly, each fraction was incubated with 0.05 mL of FeCl2.4H2O (2 mM). Then 0.2 mL ferrozine (5 mM) was added to the mixture and the volume was adjusted to 1 mL with methanol. After 10 min incubation, the absorbance was taken at 562 nm. Ethylenediaminetetraacetic acid (EDTA) served as the positive control, and untreated samples were served as the negative control. Triplicate samples were run for each set and mean values were used.
Total antioxidant capacity (TAC)
The assay was done according to the method described by Prieto et al.[Citation28] The tubes containing fraction and reagent solution (0.6 M sulfuric acid, 28 mM sodium phosphate, and 4 mM ammonium molybdate) were incubated at 90°C for 90 min. After cooling at room temperature, the absorbance was taken at 695 nm against a blank. The antioxidant capacity was expressed as mg ascorbic acid equivalent (AAE/g fraction) and GAE/g fraction.
Detection and quantification of polyphenols by HPLC
Detection and quantification of selected phenolic compounds in the fraction were performed by HPLC- diode array detector (DAD) analysis as described by Chuanphongpanich and Phanichphant.[Citation29] It was carried out on a Dionex UltiMate 3000 system equipped with quaternary rapid separation pump (LPG-3400RS) and photodiode array detector (DAD-3000RS). Acclaim® C18 (5µm) Dionex column (4.6 × 250 mm) at 30°C with a flow rate of 1 mL/min and an injection volume of 20 µL was used for the separation of compounds. The mobile phase consisted of acetonitrile (solvent A), acetic acid solution pH 3.0 (solvent B), and methanol (solvent C) with the gradient elution program of 10%A/80%B/10%C (0–9 min), 20%A/60%B/20%C (10–19 min), and 100%A (20–30 min) with the post-run equilibration of the system with 5%A/95%B (5 min). The UV detector was set to 280 nm for 18 min, changed to 320 nm for 6 min, and finally to 380 nm and held for the rest of the analysis period while the DAD was set at an acquisition range from 200 to 700 nm. A standard stock solution was prepared in methanol containing GA, VA, CH, EC, PCA, RH, EA (20 µg/mL each), CA (8 µg/mL), and QU (6 µg/mL) for the preparation of calibration curve. A solution of MS of S. apetala was prepared at 5 mg/mL. Prior to HPLC analysis, all solutions (mixed standards, sample, and spiked solutions) were filtered through 0.20 µm nylon syringe filter (Sartorius, Germany) and then degassed in an ultrasonic bath (Hwashin, Korea) for 15 min. Data acquisition, peak integration, and calibrations were performed with Dionex Chromeleon Software (Version 6.80 RS 10).
Statistical Analysis
Results were expressed as mean ± standard deviation (SD) for a given number of observations, n = 3–5. The level of significance was set at p-value of 0.05.
Results
Nutritional Compositions in Pericarp and Seed
Moisture contents in pericarp and seed of S. apetala fruit were determined. Pericarp showed high content of moisture, 84.2 ± 1.4%, whereas seed showed 55.6 ± 1.4% (). also showed the amount of carbohydrates, proteins, lipids, and ash in dried powders of pericarp and seed of the fruit. Similar amounts of carbohydrates were found present in pericarp and seed. Amounts of proteins and lipids were higher in seed than in pericarp which had the higher content of ash. The nutritive values of pericarp and seed were 178.6 ± 2.3 and 197.1 ± 14.9 Kcal/100 g, respectively.
TABLE 1 Nutrient compositions of pericarp and seed of S. apetala fruit
Concentrations of mineral-elements in both pericarp and seed of the fruits were shown in . Mineral elements can be classified into two groups: macro-elements such as Ca, K, Mg, Na, P, and S and micro-elements such as Cu, Fe, Mn, and Zn. On an average, pericarp had higher content of minerals than seed. Pericarp and seed contained nearly the same amount of S, Cu, and Mn, whereas about two times higher content of Zn, Mg, and P were found in seeds, but the contents of Fe, Ca, Na, and K were found higher in the pericarps. Potassium (K) was the most abundant macro-element followed by Na, Ca, Mg, P, and S, whereas among the micro-elements, Fe was at the top followed by Mn, Zn, and Cu of the fruit ().
Total Phenolic Content
The total phenolic contents in different pericarp and seed fractions of S. apetala (HP, DP, CP, EP, MP, HS, DS, CS, ES, and MS) were determined (). The amount was the highest in MS (221.9 mg GAE/g fraction), which also demonstrated a very high significant result compared to the rest of the fractions. It was followed by DS (33.4 mg GAE/g fraction), which showed an average phenolic content. The rest of the fractions exhibited a relatively low total phenolic content.
TABLE 2 Total polyphenols content, DPPH, and NO free radicals scavenging activities, reducing power, and Fe2+ ions chelating of different fractions of pericarp and seed of S. apetala fruit
Antioxidant Activity
Free radical scavenging activity
The DPPH and NO free radical scavenging activities of the fractions of pericarp and seed of S. apetala (HP, DP, CP, EP, MP, HS, DS, CS, ES, and MS) were determined (). The percentage scavenging of DPPH free radical ranged from 93.4 to 1.9% at 100 µg/mL. At this concentration, DS, ES, and MS showed the larger DPPH radical scavenging activity (all were above 80%), whereas HS showed no activity. Dose-dependent DPPH free radical scavenging activities of DS, ES, and MS were shown in where AA was used as positive control. MS showed the highest activity in scavenging DPPH free radicals with the lowest IC50 value of 2.1 µg/mL, which was smaller than AA (IC50 4.3 µg/mL) followed by ES (30.3 µg/mL) and DS (38.2 µg/mL). For NO free radicals scavenging, MS showed the highest activity (51.8%) followed by DS (37.8%) and MP (16.5%) at the concentration of 800 µg/mL. Dose-dependent NO scavenging activities of DS, ES, MS, and MP were shown in , where GA served as positive control. Of the fractions, MS had the lowest IC50 value (490.8 µg/mL) for NO scavenging followed by DS (858 µg/mL).
FIGURE 1 Antioxidant activity of different fractions of pericarp, and seed of S. apetala fruits. Dose-dependency of (A): the DPPH; (B): NO free radical scavenging activities of potential fractions (AA: ascorbic acid; GA: gallic acid, positive control); (C) inhibitory concentration 50 (IC50) for chelating Fe2+ ions by the fractions (EDTA: ethylenediaminetetra acetic acid, positive control); and (D) comparison of total antioxidant capacity of the fractions (AAE: ascorbic acid equivalent, GAE: gallic acid equivalent). Data are presented as mean ± SD (bar), n = 3–5. H, D, C, E, and M represent for n-hexane, diethyl ether, chloroform, ethyl acetate, and methanol, respectively; S: fraction of seed and P: fraction of pericarp.
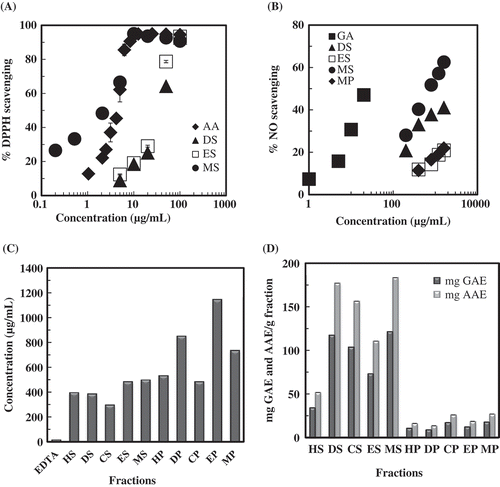
Reducing power
The more antioxidant compounds reduce the more oxidized form of ferric iron (Fe3+) to ferrous iron (Fe2+). In the present study, reducing powers of different fractions of both pericarp and seed were determined using the potassium ferricyanide reduction method as shown in . At 100 µg/mL concentration, MS had the highest absorbance (OD 1.67) followed by ES (OD 0.35), DS (OD 0.32), and CS (OD 0.12). They showed dose-dependent increase in their reducing power (data not shown).
Ferrous (Fe2+) ion chelating activity
Fe2+ ion chelating activity of the fractions was evaluated (). At the 250 µg/mL, CS showed the highest ferrous ion chelating activity (48.7%). Dose-dependent Fe2+ ion chelating activities of the fractions were measured and depending on the curves their IC50 values were calculated (). CS was found to be the most promising chelator, with the lowest IC50 value (296.4 µg/mL) followed by DS (385.7 µg/mL) and HS (396.8 µg/mL).
Total antioxidant capacity (TAC)
TAC was expressed as the mg AAE/g fraction, and as the mg GAE/g fraction of different fractions of pericarp and seed as shown in , MS had the highest antioxidant capacity (183.3 mg AAE/g; 121.4 mg GAE/g) followed by DS (177.1 mg AAE/g; 117.3 mg GAE/g), CS (156.4 mg AAE/g; 103.4 mg GAE/g), and ES (110.5 mg AAE/g; 73.2 mg GAE/g).
Determination of polyphenols in methanol seed fraction (MS) of S. apetala
The chromatographic separations of polyphenols in MS by HPLC were given in . The content of each phenolic compound was calculated from the corresponding calibration curve and was presented as the mean of five determinations as shown in . MS contained high level of GA, CH, EC, and EA (416.7, 1459.3, 310.1, and 616.9 mg/100 g of fraction, respectively). CA and QU were also detected but at lower concentrations (88.1 and 71.8 mg/100 g of fraction, respectively). The HPLC chromatogram also showed peaks in regions which represented simple polyphenols, anthocyanins, flavonoid aglycones and flavonoid glycosides ().
FIGURE 2 (A): HPLC chromatogram of mixed standards. Peaks: 1: gallic acid; 2: (+)-catechin; 3: vanillic acid; 4: caffeic acid; 5: (–)-epicatechin; 6: p-coumaric acid; 7: rutin; 8: ellagic acid; 9: quercetin. (B): HPLC chromatogram of MS. Peaks: 1: gallic acid; 2: (+)-catechin hydrate; 3: caffeic acid; 4: (–)-epicatechin; 5: ellagic acid; 6: quercetin.
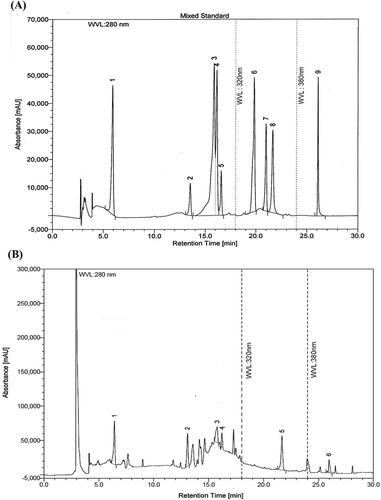
TABLE 3 Amount of polyphenolic compounds in the methanol fraction (MS) of S. apetala seeds (n = 5)
Discussion
The fruit of S. apetala is largely consumed in coastal Bangladesh, India, Myanmar, Malaysia, and other countries. It is necessary to explore this fruit for providing food security, nutraceuticals, and income generation of coastal population. It was found that the moisture contents of pericarp and seed of S. apetala were 84.2 and 55.6%, respectively. Moisture content of edible unripe mangrove fruit of Nypa fruticans had 90%.[Citation30] Determination of moisture content is one of the most important measurements for processing and testing of foods. High amount of moisture decreases the stability and quality of fruits due to rapid growth of microbes and reduced amount of proximate content. Carbohydrates, proteins, lipids, ash, and elemental mineral contents of both pericarp and seed of S. apetala were analyzed (). Pericarp and seed of S. apetala had higher amount of carbohydrates and proteins than both ripe and unripe fruits of N. fruticans.[Citation30] Therefore, fruits of S. apetala might be considered a good source of carbohydrates and proteins, at least for the coastal vulnerable people in the tropical regions. Gunawan et al.[Citation31] reported high amount of carbohydrates (52.42–63.32%), proteins (4.76–10.14%), lipids (10.65–11.09%), and ash (10.07–11.59%) in the seeds of inedible Xylocarpous moluccensis fruits. Among the mineral macro-elements, K content was found the highest and among the micro-elements, Fe was at the top in S. apetala fruit. Sulaiman et al.[Citation32] reported K as the major element in banana pulps and peels. Reportedly, mineral elements are involved in various physiological and metabolic processes, especially in bone formation, blood clotting, muscles contraction, enzymes activity, etc. Therefore, fruit of S. apetala may be used as a good source of nutritional supplements, at least for the coastal tropical people living adjacent to the mangroves.
Among the various bioactive components in fruits, phenolic compounds are the largest group of phytochemicals that have shown potentiality in preventing various diseases and promoting both physical and mental health. Although we reported polyphenols content and antioxidant activity of S. apetala fruits,[Citation16] the present study is different with respect to extracts-used, antioxidant assay-conduct and detection and quantification of phenolic compounds. Among the fractions, MS showed very high content of polyphenols (221.9 mg GAE/g fraction) followed by DS (33.4 mg GAE/g fraction). Antolovich et al.[Citation33] reported that the extraction of phenolic compounds from the fruit is commonly achieved with methanol or aqueous methanol. Seeds are propagation organ of the plant and the larger content of polyphenols in it may effectively act as an adaptive and defense molecules in adverse condition such as high salinity and sunlight exposure. Among the fractions, MS had the lowest IC50 values of 2.1 and 490.8 μg/mL for scavenging DPPH and NO free radicals, respectively. The lowest IC50 means that it had the strongest DPPH and NO free radical scavenging activities. It may be due to the presence of high amount of polyphenols in MS since positive correlation between total phenolics and radical scavenging activity was shown in various reports. However, it was reported that only phenolics with a certain structure and with hydroxyl groups at specific positions can show radical scavenging activity.[Citation34] In previous reports, we showed the IC50 values for DPPH and/or NO free radical scavenging of various common edible fruits,[Citation6] antidiabetic medicinal plants,[Citation7]and common fruity and leafy vegetables.[Citation9,Citation10] It has been found that cysteine, glutathione, AA, tocopherol, polyhydroxy aromatic compounds, and aromatic amines reduce and decolorize DPPH by their hydrogen donating ability.[Citation24]
Reportedly, the activity of antioxidants is concomitant with the development of reducing power. MS showed very high reducing power (OD 1.67 at 100 µg/mL), probably because of its high content of polyphenols as well as hydrogen donating capacity. In metal ion (Fe2+) chelating, CS showed the lowest IC50 (296.4 µg/mL) followed by DS and HS. ES, MS, CP, and HP showed near to similar IC50 values for Fe2+ ions chelation. Therefore, the fruit consists of both hydrophilic and lipophilic metal chelating properties, which may decrease metal-catalyzed radical reactions such as lipid peroxidation. Jacobsen[Citation35] reported that scavenging of free lipid radicals, peroxyl, and alkoxyl radicals is the most important mechanism for preventing lipid oxidation. Furthermore, MS and DS showed near to similar as well as the highest TAC followed by CS and ES, . Therefore, it is noteworthy that the fruit possesses functional components, which are able to scavenge free radicals and sequester metal ions via the metal catalyzed route. Arab-Tehrany et al.[Citation36] reported that some antioxidants are able to contribute to more than one antioxidant mechanism like metal chelation and scavenging of reactive oxygen species. Antioxidant properties of the seeds of S. apetala are not only strictly distributed in polar fraction, methanol (MS) but also to non-polar fractions. Therefore, seeds of S. apetala could be used as a potential source of both hydrophilic and lipophilic antioxidants. Lipophilic antioxidants could easily penetrate into the lipophilic organs such as brain, liver, and skin, thereby preventing them from oxidation. In previous reports, we categorized common fruity and leafy vegetables depending on their content of both hydrophilic and lipophilic antioxidants.[Citation9,Citation10]
Chemical compounds such as polyphenols, flavonoids, saponins, tannins, alkaloids, steroids, etc. found in mangroves are of great use, interest and demand to modern industry and medicine.[Citation37] Reportedly, polyphenolic compounds contribute significantly to the taste, flavor, and color of various foods, vegetables, fruits, and drinks. In this experiments, we have chosen the nine polyphenolic compounds since they are commonly found in fruits, vegetables, teas, and mangrove plants. MS had large amounts of GA, CH, EC, and EA and relatively a small amount of CA and QU. Polyohenols like CA, CH, EA, EC, GA, and QU have various beneficial physiological activities. In fact, they are well-known polyphenols and exhibit strong antioxidant activity. In most cases, they showed synergistic effects and their antioxidant potency varied with structural disimilarities.[Citation38] On the contrary, no synergistic effects of polyphenolic interactions were observed in pineapple peels.[Citation39] showed a few unidentified peaks in the chromatogram because we used only nine phenolic standards to quantify them in the MS of S. apetala fruit. The unidentified peaks appeared in the regions which recognized the presence of simple polyphenols, catechins, anthocyanins, and flavonoid glycosides[Citation40] in MS. Patra et al.[Citation15] reported catechol-like phenolic compounds in the methanol extracts of leaf and bark of S. apetala. However, attention should be paid to any potential cytotoxic effect that might result in using MS for the preparation of dietary supplements, and in the enrichment of beverages or foods, since some polyphenols have also been found to perturb the membrane structure.[Citation11,Citation12]
Conclusions
Edible mangrove fruit of S. apetala is a natural treasure of functional phytochemicals, dietary nutrients, and mineral elements. MS of S. apetala comprised very high polyphenolic compounds and thus, showed strong antioxidant activity. Various bioactive activities of the fruit might be, at least to some extent, the results from synergistic effects of the identified polyphenols, CA, CH, EA, EC, GA, and QU. Furthermore, seeds of S. apetala could be used as an enriched source of both hydrophilic and lipophilic functional dietary components for promoting physiological health in humans, and the present HPLC method could be used effectively to identify and quantify polyphenols in dietary substances. The fruit could easily be cultivated in the tidal sea-water intruded vast tropical coastal regions of the world for the purposes of food security, primary health care, environmental protection, income generation, and to prepare functional foods or dietary supplements.
Funding
The present research was supported by the grant in 2012–2013 from the Ministry of Science and Technology, Govt. of the Peoples Republic of Bangladesh, which is gratefully acknowledged. The authors report no conflict of interest.
Additional information
Funding
References
- Pandey, K.B.; Rizvi, S.I. Plant Polyphenols As Dietary Antioxidants in Human Health And Disease. Oxidative Medicine and Cellular Longevity 2009, 2, 270–278.
- Kris-Etherton, P.M.; Hecker, K.D.; Bonanome, A.; Coval, S.M.; Binkoski, A.E.; Hilpert, K.F.; Griel, A.E.; Etherton, T.D. Bioactive Compounds in Foods: Their Role in the Prevention of Cardiovascular Diseases and Cancer. The American Journal of Medicine 2002, 113, 71S–88S.
- Di Matteo, V.; Esposito, E. Biochemical and Therapeutic Effects of Antioxidants in the Treatment of Alzeimer’s Disease, Parkinson’s Disease, and Amyotrophic Lateral Sclerosis. Current Drug Targets-CNS Neurological Disorders 2003, 2, 95–107.
- Ames, B.N.; Shigenaga, M.K.; Hagen, T.M. Oxidants, Antioxidants, and the Degenerative Diseases of Aging. Proceedings of the National Academy of Sciences of the USA 1993, 90, 7915–7922.
- Kumar, D.; Singh, S.; Singh, A.K.; Rizvi, S.I. Pomegranate (Punica granatum) Peel Extract Provides Protection Against Mercuric Chloride-Induced Oxidative Stress in Wistar Strain Rats. Pharmaceutical Biology 2013, 51, 441–446.
- Hossain, S.J.; Tsujiyama, I.; Takasugi, M.; Islam, M.A.; Biswas, R.S.; Aoshima, H. Total Phenolic Content, Antioxidative, Anti-Amylase, Anti-Glucosidase, and Antihistamine Release Activities of Bangladeshi Fruits. Food Science and Technology Research 2008, 14, 261–268.
- Basar, M.H.; Hossain, S.J.; Sadhu, S.K.; Rahman, M.H. A Comparative Study of Antioxidant Potential of Commonly Used Anti-Diabetic Plants in Bangladesh. Oriental Pharmacy and Experimental Medicine 2013, 13, 21–28.
- Arif, K.M.T.; Datta, R.K.; Sarower, M.G.; Hossain, S.J. Comparison of Phenolics and Antibacterial Activity of Commonly Used Antidiabetic Medicinal Plants in Bangladesh. Pharmacologyonline 2014, 1, 7–15.
- Hossain, S.J.; Sultana, M.S.; Taleb, M.A.; Basar, M.H.; Sarower, M.G.; Hossain, S.A. Antioxidant Activity of Ethanol and Lipophilic Extracts of Common Fruity Vegetables in Bangladesh. International Journal of Food Properties 2014, 17, 2089–2099.
- Hossain, S.J.; Sultana, M.S.; Iftekharuzzaman, M.; Hossain, S.A.; Taleb, M.A. A Comparative Study on Antioxidant Potential of Common Leafy Vegetables in Bangladesh. Bangladesh Journal of Botany 2015, 44, 51–57.
- Hossain, S.J.; Kato, H.; Aoshima, H.; Yokoyama, Y.; Yamada, M.; Hara, Y. Polyphenol-Induced Inhibition of the Response of Na+/Glucose Cotransporter Expressed in Xenopus Oocytes. Journal of Agricultural and Food Chemistry 2002, 50, 5215–5219.
- Hossain, S.J.; Aoshima, H.; Koda, H.; Kiso, Y. Review of Functional Studies of Beverage Components Acting on the Recombinant GABAA Neuroceptor, and Na+/Glucose Cotransporter-Response Using the Xenopus Oocyte Expression System and Electrophysiological Measurements. Food Biotechnology 2007, 21, 237–270.
- International Plant Genetic Reesources Institute (IPGRI), 2001–2005. Conserving and Increasing the Use of Neglected and Underutilized Crop Species. http://www.ipgri.cgiar.org/institute/siteinfo.html ( accessed October 15, 2005).
- Bandaranayake, W.M. Traditional and Medicinal Uses of Mangroves. Mangroves and Salt Marshes 1998, 2, 133–148.
- Patra, J.K.; Das, S.K.; Thatoi, H. Phytochemical Profiling and Bioactivity of a Mangrove Plant, Sonneratia Apetala, from Odisha Coast of India. Chinese Journal of Integrated Medicine 2015, 21, 274–285.
- Hossain, S.J.; Basar, M.H.; Rokeya, B.; Arif, K.M.T.; Sultana, M.S.; Rahman, M.H. Evaluation of Antioxidant, Antidiabetic, and Antibacterial Activities of the Fruit of Sonneratia Apetala (Buch.-Ham.). Oriental Pharmacy and Experimental Medicine 2013, 13, 95–102.
- Shefa, A.A.; Baishakhi, F.S.; Islam, S.; Sadhu, S.K. Phytochemical and Pharmacological Evaluation of Fruits of Sonneratia Apetala. Global Journal of Medical Research: B Pharma, Drug Discovery, Toxicology, and Medicine 2014, 14, 1–6.
- Dubois, M.; Giles, K.A.; Hamilton, K.S.; Rebers, P.A.; Smith, F. Colorimetric Method for the Determination of Sugar and Related Substances. Analytical Chemistry 1956, 18, 350–356.
- Lowry, O.H.; Rosebrough, N.J.; Farr, A.L.; Randall, R.J. Protein Measurement with the Folin Phenol Reagent. Journal of Biological Chemistry 1951, 193, 265–275.
- Bligh, E.G.; Dyer, W.J. A Rapid Method of Total Lipid Extraction and Purification. Canadian Journal of Biochemistry and Biophysiology 1959, 37, 911–915.
- Gul, S.; Safdar, M. Proximate Composition and Mineral Analysis of Cinnamon. Pakistan Journal of Nutrition 2009, 8, 1456–1460.
- Hoenig, M.; de Kersabiec, A.M. Sample Preparation Steps for Analysis by Atomic Absorption Spectroscopy Methods: Present Status. Spectrochimica Acta Part B: Atomic Spectroscopy, 1996, 51, 1297–1307.
- Ough, C.S.; Amerine, M.A. Methods for Analysis of Musts and Wine; John Wiley & Sons: New York, NY, 1988.
- Blois, M.S. Antioxidant Determinations by the Use of a Stable Free Radical. Nature 1958, 181, 1199–1200.
- Marcocci, L.; Maguire, J.J.; Droy-Lefaix, M.T.; Packer, L. The Nitric-Oxide Scavenging Properties of Ginkgo Biloba Extract EGb 761. Biochemical and Biophysical Research Communications 1994, 201, 748–755.
- Oyaizu, M. Studies on Products of Browning Reaction: Antioxidative Activity of Products of Browning Reaction Prepared from Glucosamine. Japanese Journal of Nutrition 1986, 44, 307–315.
- Carter, P. Spectrophotometric Determination of Serum Iron at the Submicrogram Level with a New Reagent (Ferrozine). Analytical Biochemistry 1971, 40, 450–458.
- Prieto, P.; Pineda, M.; Aguilar, M. Spectrophotometric Quantitation of Antioxidant Capacity Through the Formation of a Phosphomolybdenum Complex: Specific Application to the Determination of Vitamin E. Analytical Biochemistry 1999, 269, 337–341.
- Chuanphongpanich, S.; Phanichphant, S. Method Development and Determination of Phenolic Compounds in Broccoli Seeds Samples. Chiang Mai Journal of Science 2006, 33, 103–107.
- Sum, P.C.; Khoo, H.E.; Azlan, A. Comparison of Nutrient Composition of Ripe and Unripe Fruits of Nypa Fruticans. Fruits 2013, 68, 491–498.
- Gunawan, S.; Darmawan, R.; Nanda, M.; Setiawan, A.D.; Fansuri, H. Proximate Composition of Xylocarpous Moluccensis Seeds and Their Oils. Industrial Crops and Products 2013, 41, 107–112.
- Sulaiman, S.F.; Yusoff, N.A.M.; Eldeen, I.M.; Seow, E.M.; Sajak, A.A.B.; Supriatno, Ooi, K.L. Correlation Between Total Phenolic and Mineral Contents with Antioxidant Activity of Eight Malaysian Bananas (Musa sp.). Journal of Food Composition and Analysis 2011, 24, 1–10.
- Antolovich, M.; Prenzler, P.; Robards, K.; Ryan, D. Sample Preparation in the Determination of Phenolic Compounds in Fruits. The Analyst 2000, 125, 989–1009.
- Nguelefack, T.B.; Mbakam, F.H.K.; Tapondjou, L.A.; Watcho, P.; Nguelefack-Mbuyo, E.P.; Ponou, B.K.; Kamanyi, A.; Park, H.J. A Dimeric Triterpenoid Glycoside and Flavonoid Glycosides with Free Radical-Scavenging Activity Isolated from Rubus Rigidus Var. Camerunensis. Archives of Pharmacal Research 2011, 34, 543–550.
- Jacobsen, C. Challenges when Developing Omega-3 Enriched Foods. Oleagineoux Corps Gras Lipids 2010, 17, 251–258.
- Arab-Tehrany, E.; Jacquot, M.; Gaiani, C.; Imran, M.; Desobry, S.; Linder, M. Beneficial Effects and Oxidative Stability of Omega-3 Long-Chain Polyunsaturated Fatty Acids. Trends in Food Science and Technology 2012, 25, 24–33.
- Bandaranayake, W.M. Bioactivities, Bioactive Compounds, and Chemical Constituents Of Mangrove Plants. Wetlands Ecology and Management 2002, 10, 421–452.
- Meyer, A.S.; Heinonen, M.; Frankel, E.N. Antioxidant Interactions of Catechin, Cyanidin, Caffeic Acid, Quercetin, and Ellagic Acid on Human LDL Oxidation. Food Chemistry 1998, 61, 71–75.
- Li, T.; Shen, P.; Liu, W.; Liu, C.; Liang, R.; Yan, N.; Chen, J. Major Polyphenolics in Pineapple Peels and Their Antioxidant Interactions. International Journal of Food Properties 2014, 17, 1805–1817.
- Sakakibara, H.; Honda, Y.; Nakagawa, S.; Ashida, H.; Kanazawa, K. Simultaneous Determination of All Polyphenols in Vegetables, Fruits, and Teas. Journal of Agricultural and Food Chemistry 2003, 51, 571–581.