Abstract
This study evaluated and correlated the viscoelastic and nutritional properties of carrots after freezing (–20, –70, and –196oC) and thawing (4 and 18oC) treatments. Results showed that all samples exhibited a solid behavior (storage modulus G′ > loss modulus G″) dominating the viscoelastic response. After treatments, G′, G″, hardness, fracturability, springiness, and chewiness of carrots significantly decreased, whereas loss tangent (Tanδ) increased. Compared with other treatments, fast freezing (–196oC) and thawing (18oC) better maintained G′, G″, hardness, and fracturability of carrots, and intermediate freezing (–70oC) better retained springiness, cohesiveness, and chewiness. For nutritional parameters, fast freezing and thawing conditions also contributed to preserving the contents of carotenoids of carrots. Partial least squares regression analysis revealed that β- and α-carotene, lycopene, and soluble sugars were positively correlated with hardness, fracturability, G′, and G″, and negatively with Tanδ in carrots. The variation of viscoelastic parameters could well predict the changes at nutritional levels.
INTRODUCTION
Recently, freezing is recognized as an effective method to preserve foods. However, the occurrence of crystallization/re-crystallization during freezing/thawing processes may negatively influence the qualities of plant foods. Although the pretreatments (e.g., blanching, cryoprotectant effect, and pulsed electric field) can limit the freezing degradation,[Citation1–Citation4] these pretreatments may modify the initial texture and composition of plant foods. Besides, food qualities should be dependent on not only freezing means but also thawing conditions.[Citation5–Citation7] Therefore, a fundamental understanding of the effect of freezing/thawing processes themselves on food properties can better meet the consumers’ desire for unadulterated foods.
Plant foods can exhibit a viscoelastic property. Its changes can reflect the changes of the tissue structure and cause tissue softening. It is a crucial contributor to the deterioration of foods during frozen storage.[Citation8] Torres and Canet[Citation9] have showed that thawing conditions may distinctly influence the rheological properties of frozen vegetable purees. Rapid freezing and microwave-thawing have obtained ideal oscillator parameters (e.g., storage and loss modulus and complex viscosity) of mashed potatoes, which are more similar to the freshly made.[Citation10] Varying the temperature and geometry can result in different oscillatory rheological properties of mashed potatoes.[Citation11] However, these works are limited to frozen vegetable purees. Very few studies on the viscoelastic properties of frozen vegetables have been reported. Freezing/thawing processes can decrease the elastic properties of vegetables by one or two orders of magnitude.[Citation12] To our knowledge, the comparison of viscoelastic behaviors of vegetables at different freezing/thawing conditions has not been investigated. Monitoring their detailed information could be in favor of providing an optimum condition and a maximum product quality.
Moreover, Tomlins, Owori, Bechoff, Menya, and Westby[Citation13] have indicated that the content of total carotenoid is highly correlated with a wide range of sensory characteristics, involving texture, odor, and taste of sweet potatoes. Carotenoid-absorptions (400–500 nm) link to penetrometer firmness, with a correlation coefficient equal to −0.69.[Citation14] Besides, the texture and β-carotene bio-accessibility in vitro of carrots are inversely correlated.[Citation15] The more material mass of vegetables is lost, the higher maximal cutting force is used.[Citation16] Hence, we suggest that there seems to be a relationship between the nutritional and mechanical properties in plant tissues. A better understanding of their relationship could be conductive to improving the quality control and process design in the food industry and the marketplace.
Along these lines, our study was conducted to assess the effect of different freezing/thawing temperatures on the viscoelastic characterizations at large and small deformations (from large-scale texture profile analysis [TPA] and small-scale dynamic mechanical analysis [DMA]), the contents of carotenoid and soluble sugar (from high-performance liquid chromatography [HPLC]), and cell microstructure (from scanning electronic microscopy) of carrots. Furthermore, the relationship between viscoelastic and nutritional parameters in frozen/thawed carrots was explored using partial least squares (PLS) regression analysis
MATERIALS AND METHODS
Materials and Sample Preparation
Carrots were harvested in September in Shanghai, China. Fresh carrots (Daucus carota L.) without any physical damage were purchased from a local market. Cubic samples (about 8 mm wide, 8 mm thick, and 8 mm long) were shaped from the parenchyma of selected roots using a sharp razor blade. Freezing processes were performed according to the method[Citation17] with some modifications. Three methods were applied: slow freezing at –20oC in a chest freezer (MDF-135, Sanyo Electric Co. Ltd., Japan), intermediate freezing at –70oC in a cryogenic freezer (Revco, Asheville, NC), and rapid freezing at –196oC by submerging in liquid nitrogen. After samples were completely frozen, they were placed in airtight plastic bags and stored at –20oC for 30 d. Subsequently, frozen samples were thawed at 4oC for 4 h in a refrigerator (Hisense, Hisense Co. Ltd., China) and at 18oC (room temperature) for 2 h, respectively. Raw samples were analyzed as the control group.
Instrumental TPA
The TPA was conducted in a TA-XT Plus Texture Analyzer (Stable Micro Systems Ltd., Surrey, UK) using a 500 N load cell. A two cycle compression test was performed using an aluminum cylinder probe (50 mm diameter), which was used to compress samples to 75% of their original thickness at a compression rate of 1 mm/s. Hardness1 and hardness2 during the first and second compression cycles, respectively, springiness, cohesiveness, chewiness, and resilience were obtained from the force-time curves.[Citation18] Twelve measurements were performed for each treatment.
Dynamic Mechanical Analysis (DMA)
According to our previous method,[Citation19] the storage (G′) and loss (G″) modulus and loss tangent (Tanδ) were measured in a compression configuration on Perkin Elmer DMA 8000 (Waltham, MA, USA). To meet the requirement of the compression test, rectangular slices (about 5 mm wide, 6.5 mm thick, and about 6 mm long) were shaped from the treated samples using a sharp razor blade. The frequency sweep test was performed at 1–55 Hz and at 21.5 ± 0.2°C. The average value of all points on a sweep curve was considered as the value of one measurement. Data were reported as the mean value of six measurements for each treatment.
Soluble Sugar Determination
For the extraction, deionized water (5 mL) was added to freeze-dried powder (100 mg) and stirred in a hot bath at 80oC for 20 min. The mixture was centrifuged at 12,000 rpm for 20 min to gain the supernatant. The resulting residues were re-extracted using 4 mL of deionized water. Two supernatants were collected and diluted to 10 mL with deionized water for quantification. After being filtered with 0.45 μm filter unit (Beijing Bomex Co., Beijing, China), a 10 μL aliquot was injected into a HPLC system (Series 200, Perkin Elmer, Inc., USA) equipped with a refractive index detector (PE 200, Perkin Elmer, Inc., USA) and an Inertsil amino column (250 × 4.6 mm ID, GL Sciences, Japan). Acetonitrile/water (75:25, v/v) was used as the solvent at a flow rate of 1 mL min−1 at 40oC. Sucrose, fructose and glucose was quantified using the external standard method (i.e., a mixture of HPLC-grade sucrose, glucose, and fructose) and expressed as mg g−1 dry matter. Determinations were performed in triplicate.
Carotenoid Determination
Methanol (6.3 mL) and 60% potassium hydroxide solution (700 μL) were added to 100 mg freeze-dried powder, swirled, and put in a hot bath at 60oC for 30 min. Subsequently, 6 mL of Tris Buffer grade (containing 50 mM Tris solution and 1 M sodium chloride solution, pH 7.5) was added to the mixture and swirled. After standing at 4oC for 10 min, 16 mL of chloroform was added to the mixture and swirled. The mixture was centrifuged at 4000 rpm at 4°C for 15 min after standing at a cold ice for 10 min. These procedures were repeated twice to ensure the maximum extraction. Finally, the organic phase, containing carotenoids, was dried under nitrogen and dark conditions. The extracts were re-dissolved with hexane and filtered through a 0.22 μm filter unit before HPLC analysis. Carotenoids were separated on a YMC-carotenoid S-5column (250 × 4.6 mm; YMC Co., Ltd.). The injection volume was 60 μL. The mobile phase consisted of three different solvent mixtures: A, methanol; B, 0.2% ammonium acetate solution: methanol (28:80, v/v); and D, methyl tert-butyl ether. The gradient elution was used at a flow rate of 1 mL/min: 0–12 min, Isocratic elution (95%A and 5%B); 12–17 min, 95–80%A, 5%B, and 0–15%D; 17–27 min, 80–50%A, 5%B, and 15–45%D; 27–37 min, 50–25%A, 5%B, and 45–70%D; 37–50 min, 25%A, 5%B, and 70%D; 50–55 min, 25–95%A, 5%B, and 70%-0D; and 55–70 min, 95%A and 5%B. The detection was carried out at 446 nm at 25°C. Quantification was conducted by the external standard method (i.e., a mixture of HPLC-grade β-carotene, α-carotene, Lycopene, and lutein) and expressed as mg kg−1 dry matter. Determinations were performed in triplicate.
Scanning Electron Microscopy (SEM)
Prior to the microstructure observation, the samples were freeze dried in a freezer dryer (Sihuan LGJ-25C, Sihuan Co. Ltd, Beijing, China) for 24 h. Subsequently, the dried samples were coated with a fine gold layer and analyzed using a SEM (Sirion 200, FEI, Holland) at 100 × magnification.
Statistical Analysis
One-way analysis of variance was performed to evaluate the difference among the treatments. Significance level was set at p < 0.05. Data were expressed as mean ± standard deviation (SD). Following data standardization and co-linearity diagnostics, linear PLS was performed by combining the data from all treatments to address associations between x-variables (viscoelastic parameters including the TPA and DMA parameters) and y-variables (the contents of carotenoids and soluble sugars). Statistical analyses were performed with SAS v 9.1 (SAS Institute, Cary, NC, USA).
RESULTS AND DISCUSSION
Microstructure
Raw samples exhibited well-organized and honeycomb-like structure. Pore size was small and homogeneous (). Cryogenic freezing at –20°C induced large holes and excessive collapse of cell walls (). Few regular-shaped cells in the structure were observed. At –70°C (), the porous structure were still clear. Pore size was small and inhomogeneous. The structure differences indicated that freezing at –70°C generated less damages to the samples than those at –20°C. One main cause was that fast freezing (–70°C) induce the formation of small ice crystals, whereas slow freezing (–20°C) generate large ice crystals. Large ice crystals can cause more severely mechanical disruption of the cells. In our case, no large pores appeared in the samples frozen at –196°C (). The low temperature instantly freezes the samples, without the occurrence of heat emanating. Under these conditions, there was not enough time to generate ice crystals. Hence, no damages from ice crystals appeared in this structure. However, some parts in the structure presented long tissue cracks. These cracks could be because that quite rapid freezing may cause the inconformity of freezing rates between the external and internal tissues. Thus, samples (i.e., carrots) with high water content (about 88%) could be prone to rupturing. Similarly, long tissue cracks were also found in the apple tissues and bellflower root frozen in liquid nitrogen.[Citation17Citation20]
TPA Parameter
After treatments, hardness1, hardness2, fracturability, springiness, and chewiness of samples significantly decreased, whereas cohesiveness increased in most of case (p < 0.05, ). Hardness1 and fracturability of treated samples increased with decreasing freezing temperature (p < 0.05). Freezing at –196oC and thawing at 18oC obtained the highest values of hardness1, hardness2, and fracturability in all of the processed samples (p < 0.05). The changes occurring at the cell structure level () could explain these results. As known, the loss of cell turgidity was a main contributor to tissue softening. When cells lose their turgidity, they are more deformable, producing a softer and rubbery texture. The structure at –20 and –70oC both exhibited large pores and destroyed cell walls. These cell damages induced a dramatic loss of cell turgidity and then lower the texture qualities of samples. Similarly, the degradation of cell walls due to freezing/thawing damage lowers the firmness values of mangoes.[Citation21] Although large cracks between cells were observed in samples at –196oC, the cells themselves were intact in most parts. This structure seemed to better maintain the hardness and fracturability of samples. Their lower values at 4°C might be because that long-time thawing might generate further deterioration to the cell structures through enhancing the actions of hydrolytic enzymes (released from the ruptured vacuoles).[Citation17] Besides, the recrystallization process can occur because of the formation of temperature gradients within the samples during freezing or thawing, or because the temperature fluctuates during storage or transportation.[Citation22] The ice crystals may grow larger through this process, resulting in more severe mechanical damages of samples. Park et al.[Citation17] have reported that thawing at 4oC could induce the occurrence of migratory recrystallization and, consequently, lower the hardness of vegetables. Therefore, the migratory recrystallization process might be another crucial factor.
TABLE 1 Texture properties of raw and carrots stored at –20oC for 30 d
Additionally, only a freezing temperature distinctly affected other TPA parameters. In treated samples, springiness at –20 and –70oC were greater than those at –196oC (p < 0.05). Cohesiveness values at –70oC were highest, followed by –20oC, and those at –196oC were lowest (p < 0.05). Chewiness at –70oC were greatest (p < 0.05). These larger values at –70°C might be due to the conjunction effect of relatively well-maintained structures and increased gumminess materials released from damaged cells.[Citation16] Similarly, the more the changes of material mass of vegetables are, the higher the maximal cutting force is used.[Citation16] Besides, long cracks between cells at –196°C might cause a great loss of cohesive strength, especially after a severe disruption from the first compression. In our case, all samples exhibited very low values of resilience. These could be related to the shapes of tested samples and processing conditions. However, its largest values at –70oC were still observed.
DMA Parameter
The G′ and G″ represent the elastic and viscous (fluid) part of material toughness, respectively. The Tanδ expresses the ratio of viscous part to elastic one, showing the dissipation of materials and the irreversibility of internal changes. In all samples, G′ exceeded G″ over the entire frequency range, showing a dominant solid behavior in carrots ( and ). This feature indicated an elastic and cross-linked structure in carrots. The G′ of all samples had a weaker dependency on frequency and increased slightly with increasing frequency (). On the other hand, in frozen/thawed samples, G″ () and Tanδ () showed obvious uptrends with increasing frequency and their dependencies on the frequency were more complex (appearing some clear shifts) compared with raw samples. These different behaviors emphasized the changes of internal structure and mechanistic properties after treatments.
FIGURE 1 Scanning electron microscope images at 100 × magnification of raw and treated carrot tissues. a: Raw tissues; b, c, and d: tissues frozen at –20, –70, –196, respectively, and thawed at 18oC. P: pore; H: hole; C: crack.
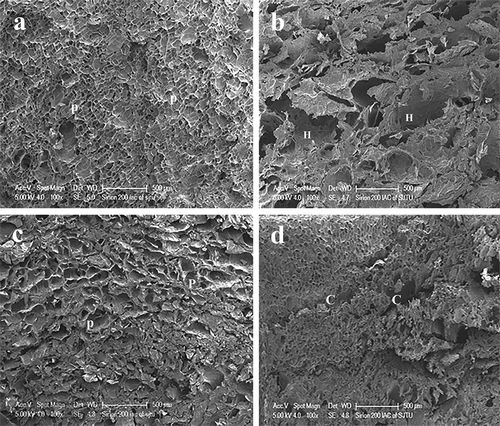
FIGURE 2 Frequency spectra for storage modulus (G′) of raw and treated carrots. Raw: raw carrot; 4–20, 70, and 196: carrots frozen at –20, –70, and –196oC, respectively, and thawed at 4oC; 18–20, 70, and 196: carrots frozen at –20, –70, and –196oC, respectively, and thawed at 18oC.
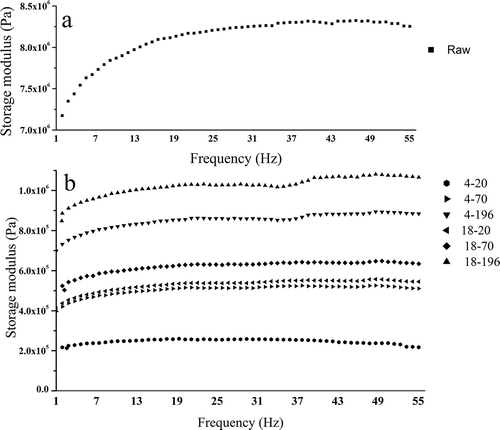
FIGURE 3 Frequency spectra for loss modulus (G″) of raw and carrots. Raw: raw carrot; 4–20, 70, and 196: carrots frozen at –20, –70, and –196oC, respectively, and thawed at 4oC; 18–20, 70, and 196: carrots frozen at –20, –70, and –196oC, respectively, and thawed at 18oC.
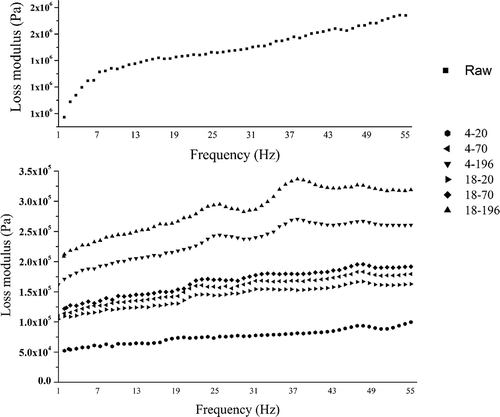
FIGURE 4 Frequency spectra for loss tangent (Tanδ) of raw and treated carrots. Raw: raw carrot; 4–20, 70, and 196: carrots frozen at –20, –70, and –196oC, respectively, and thawed at 4oC; 18–20, 70, and 196: carrots frozen at –20, –70, and –196oC, respectively, and thawed at 18oC.
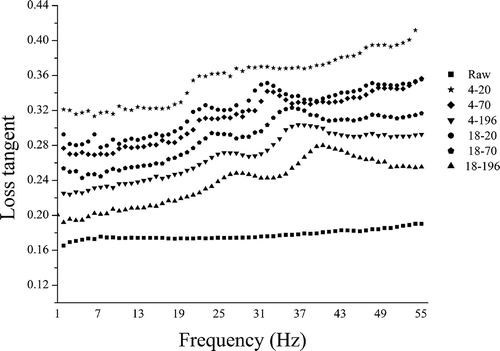
The values of G′ and G″ of frozen/thawed samples significantly decreased, whereas the Tanδ value significantly increased as compared with raw samples (p < 0.05; ). These results showed that after treatments the viscosity of samples increased and the elasticity strength decreased. In treated samples, the values of G′ and G″ of samples frozen at –196°C and thawed at 18°C were largest, and those frozen at –20°C and thawed at 4°C were lowest (p < 0.05). Regardless of 4 or 18°C thawing, the values of Tanδ of samples frozen at –196°C were significantly lower than those at –20°C (p < 0.05). It can be seen that rapid freezing (–196°C) and thawing (18°C) had an optimally protective effect on the elasticity strength.
TABLE 2 The average elastic modulus of all points on the frequency sweep curve of raw and carrots stored at –20oC for 30 d
These results could be attributed to the changes of cell turgidity and cell walls.[Citation19Citation23] Wu and Guo[Citation24] have reported that the loss of cell turgidity could directly lower the elastic response of Korla pears. In our case, cell damages from ice crystals aggravated the loss of cell turgidity, causing the reduced elasticity strength of samples. Besides, after treatments, the degradation of cell wall components and the breakage of the connection of pectin and hemicellulose may induce the generation of more viscous components in cell structures.[Citation25] These increased viscous components can reduce the intermolecular forces and enlarge the free volume of molecular movement, facilitating the local segmental peristalsis, and/or relaxation motions in material polymers. These motions could then resulted in the increases of internal losses of samples when dynamic forces were exerted on them, finally reflected in the increases of Tanδ. Moreover, cell wall components themselves (especially cellulose) possess the function of maintaining the rigidity and resistance of tissues. The degradation of cell walls could directly decrease the elastic modulus.[Citation19] Similar findings showed that the loss of cell turgidity and cell wall fluidity (from exosmosis, solubilization, and degradation) can decrease the elastic response of blanched and/or osmotic dehydrated apples.[Citation25] Based on the analysis of cell structures, rapid freezing/thawing could better maintain the cell integrity of samples. Hence, these samples obtained greater elastic strength.
Carotenoid
Carrot is regarded as an important dietary source of carotenoids. Due to the contributions to carrot qualities, such as provitamin A activity, antioxidant capacity, aroma property, and color,[Citation26] the levels of carotenoids could be used as a crucial indicator to evaluate the carrot quality during processing and storage. Raw carrots contained predominantly β-carotene, a substantial amount of α-carotene, and a low amount of lycopene and lutein (). The contents of β-carotene and α-carotene at –196°C were largest (p < 0.05), which were close to the control group (p > 0.05). Freezing at –20 and –70°C, thawing at 4°C caused them more loss than at 18°C (p < 0.05).The content of Lycopene at –70 and –196oC was larger than –20oC (p < 0.05). The content of lutein of samples frozen at –196°C and thawed at 18oC were largest, which were even larger than those from raw samples (p < 0.05). The increases could be owing to the accumulation of lutein during freezing storage.[Citation27] The content of lutein of samples frozen at –20°C and thawed at 4oC were lowest (p < 0.05). The lower contents of carotenoids at slow freezing could be the consequence of more damages of cell structures. Additionally, because of the presence of an oxygen atmosphere, it is likely that the degradation of carotenoids occurred via oxidative processes. Long-time thawing (4°C) allowed more carotenoids (released from severe cell ruptures) exposed to enzyme oxidation degradation. It is well documented that carotenoids (e.g., β-, α-carotene, and lycopene) can be oxidatively cleaved to form aldehydes, ketones, epoxides, and endoperoxides.[Citation28] Collectively, rapid freezing (–196°C) and thawing (18°C) had an effective protection for the carotenoids of carrots.
TABLE 3 Carotenoid contents of raw and carrots stored at –20oC for 30 d (expressed as mg kg–1 d.b.)
Soluble Sugar
The content of sucrose was higher than those of fructose and glucose in fresh carrots (). The contents of glucose and sucrose dramatically decreased after treatments (p < 0.05). One main reason was that soluble sugar flowed away together with the water release from the ruptured cells. Volden et al.[Citation29] have showed that soluble sugars can be lost to the blanching water due to the cell destruction. In treated samples, the content of sucrose at –196oC was largest. Its content of samples frozen at –20oC and thawed at 4oC was the lowest (p < 0.05). The content of fructose increased with decreasing freezing temperature, and the content of glucose at –70 and –196oC were larger than those at –20oC (p < 0.05). It can be seen that rapid freezing had an effective protection on the contents of soluble sugars, whereas thawing temperature had no obvious effect on them.
TABLE 4 Soluble sugar contents of raw and carrots stored at –20oC for 30 d (expressed as mg g–1 d.b.)
Correlation Between Viscoelastic and Nutritional Parameter
In the PLS analysis, the influence of x-variable on y-variable is transferred through PLS factors. If the contribution rate of a PLS factor to y-variable is large and along this factor the value of model effect weight (MEW) of x-variable is high, the influence of x-variable on y-variable is large. For carotenoids, the contribution rates of PLS factor 1 (F1) and factor 2 (F2) were 63.15 and 23.90%, respectively. Their cumulative percent was 87.05%. These results showed that viscoelastic parameters could well explained the variability of the levels of carotenoids. Hardness 1, hardness 2, fracturability, G′, G″, and Tanδ (with high MEW values of 0.387, 0.371, 0.380, 0.325, 0.340, and –0.398 along F1, respectively) were strongly related to the changes of carotenoids. As shown in , β-carotene, α-carotene, and lycopene were positively correlated to hardness 1, hardness 2, fracturability, G′, and G″, and negatively with Tanδ. Lutein showed a positive relation to hardness 1, hardness 2, and fracturability, and a negative one to G′, G″, and Tanδ. All viscoelastic parameters could well predict the levels of β-carotene, α-carotene, lycopene, and Lutein using respective standard partial regression equations with R2 = 0.911, 0.956, 0.998, and 0.923 (p < 0.01; ). For soluble sugars, the contribution rates of PLS F1 and F2 were 63.06 and 20.48%, respectively. Their cumulative percent was 83.54%. Viscoelastic parameters could also well explained the variability of the levels of soluble sugars. Hardness 1, hardness 2, fracturability, G′, G″, and Tanδ (with large MEW values of 0.382, 0.379, 0.391, 0.304, 0.323, and –0.430 along F1, respectively) were main factors that linked to the changes of soluble sugars. As shown in , soluble sugars were positively correlated to hardness 1, hardness 2, fracturability, G′, and G″, and negatively with Tanδ. All viscoelastic parameters could well predict the levels of fructose, glucose, and sucrose using respective standard partial regression equations with R2 = 0.980, 0.949, 0.933 (p < 0.01, ).
TABLE 5 Standard partial regression coefficients between mechanical and nutritional attributes
Increasing reports have suggested that the content of carotenoids was in relation to the texture attribute in fruits and vegetables. For instance, the changes of carotenoids can influencRe the sensorial properties in a wide range, involving texture, appearance, taste, and odor in sweet potatoes.[Citation13] Carrots with high content of carotene were more solid and less tender.[Citation30] The content of carotenoids (400–500 nm) vary, probably accompanied with the variation of firmness in apples.[Citation14] The changes of carotenoids can be used as an indicator of fruit maturity and firmness.[Citation31] In our case, we established the quantitative relationship between the viscoelastic and nutritional parameters in frozen/thawed carrots. The changes at nutritional levels could be well predicted by the variation of viscoelastic behaviors.
CONCLUSIONS
Freezing/thawing temperatures dramatically influenced the viscoelastic and nutritional attributes. Rapid freezing (–196oC) and thawing (18oC) contributed to maintaining the elasticity strength and nutritional qualities of carrots. Intermediate temperature (–70oC) well maintained the cohesiveness and chewiness of carrots. The PLS analysis showed that the loss of carotenoids and soluble sugars could be related to the reduction of hardness, fracturability, G′, and G″, and the increases of Tanδ in frozen/thawed carrots. The variation of viscoelastic behaviors could well predict the changes at nutritional levels. The changes occurring at the cell structure level (i.e., the loss of cell turgidity and the disruption of cell walls) could explain these changes.
FUNDING
This work was supported by a grant from national science and technology program: Research and Industrialization of Prepared Foods in City Nutrition Catering (grant number 2014BAD04B08).
ACKNOWLEDGMENTS
The authors would like to thank IAC of Shanghai Jiao Tong University for its technical assistance.
Additional information
Funding
REFERENCES
- Han, C.; Zhao, Y.; Leonard, S.W.; Traber, M.G. Edible Coatings to Improve Storability and Enhance Nutritional Value of Fresh and Frozen Strawberries (Fragaria× Ananassa) and Raspberries (Rubus Ideaus). Postharvest Biology and Technology 2004, 33, 67–78.
- Haiying, W.; Shaozhi, Z.; Guangming, C. Experimental Study on the Freezing Characteristics of Four Kinds of Vegetables. LWT–Food Science and Technology 2007, 40, 1112–1116.
- Sirijariyawat, A.; Charoenrein, S.; Barrett, D.M. Texture Improvement of Fresh and Frozen Mangoes with Pectin Methylesterase and Calcium Infusion. Journal of the Science of Food and Agriculture 2012, 92, 2581–2586.
- Shayanfar, S.; Chauhan, O.P.; Toepfl, S.; Heinz, V. Pulsed Electric Field Treatment Prior to Freezing Carrot Discs Significantly Maintains Their Initial Quality Parameters After Thawing. International Journal of Food Science and Technology 2014, 49, 1224–1230.
- Sousa, M.B.; Canet, W.; Alvarez, M.D.; Fernández, C. Effect of Processing on the Texture and Sensory Attributes of Raspberry (cv. Heritage) and Blackberry (cv. Thornfree). Journal of Food Engineering 2007, 78, 9–21.
- Holzwarth, M.; Korhummel, S.; Carle, R.; Kammerer, D.R. Evaluation of the Effects of Different Freezing and Thawing Methods on Color, Polyphenol, and Ascorbic Acid Retention in Strawberries (Fragaria×Ananassa Duch.). Food Research International 2012, 48, 241–248.
- Phothiset, S.; Charoenrein, S. Effects of Freezing and Thawing on Texture, Microstructure, and Cell Wall Composition Changes in Papaya Tissues. Journal of the Science of Food and Agriculture 2014, 94, 189–196.
- Ohnishi, S.; Fujii, T.; Miyawaki, O. Freezing Injury and Rheological Properties of Agricultural Products. Food Science and Technology Research 2003, 9, 367–371.
- Torres, M.; Canet, W. Rheological Properties of Frozen Vegetable Purees. Effect of Freeze-Thaw Cycles and Thawing Conditions. European Food Research and Technology 2001, 213, 30–37.
- Álvarez, M.D.; Fernández, C.; Canet, W. Effect of Freezing/Thawing Conditions and Long-Term Frozen Storage on the Quality of Mashed Potatoes. Journal of the Science of Food and Agriculture 2005, 85, 2327–2340.
- Álvarez, M.D.; Canet, W.; Fernández, C. The Effect of Temperature, Geometry, Gap, and Surface Friction on Oscillatory Rheological Properties of Mashed Potatoes. Journal of Food Process Engineering 2007, 30, 267–292.
- Ohnishi, S.; Shimiya, Y.; Kumagai, H.; Miyawaki, O. Effect of Freezing on Electrical and Rheological Properties of Food Materials. Food Science and Technology Research 2004, 10, 453–459.
- Tomlins, K.; Owori, C.; Bechoff, A.; Menya, G.; Westby, A. Relationship Among the Carotenoid Content, Dry Matter Content, and Sensory Attributes of Sweet Potato. Food Chemistry 2012, 131, 14–21.
- Rowe, P.I.; Künnemeyer, R.; McGlone, A.; Talele, S.; Martinsen, P.; Seelye, R. Relationship Between Tissue Firmness and Optical Properties of “Royal Gala” Apples from 400 to 1050 nm. Postharvest Biology and Technology 2014, 94, 89–96.
- Lemmens, L.; Van Buggenhout, S.; Oey, I.; Van Loey, A.; Hendrickx, M. Towards a Better Understanding of the Relationship Between the B-Carotene in Vitro Bio-Accessibility and Pectin Structural Changes: A Case Study on Carrots. Food Research International 2009, 42, 1323–1330.
- Góral, D.; Kluza, F. Cutting Test Application to General Assessment of Vegetable Texture Changes Caused by Freezing. Journal of Food Engineering 2009, 95, 346–351.
- Park, S.J.; Al Mijan, M.; Song, K.B. Quality Changes in Pteridium Aquilinum and the Root of Platycodon Grandiflorum Frozen Under Different Conditions. International Journal of Refrigeration 2014, 43, 90–96.
- Bourne, M.C. Texture Profile Analysis. Food Technology 1978, 32, 62–66.
- Xu, C.; Li, Y. Correlation of Viscoelastic Behavior with Water State and Ultrastructure In Hot Air-Dried Carrots. Food Control 2015, 50, 23–30.
- Chassagne-Berces, S.; Poirier, C.; Devaux, M.F.; Fonseca, F.; Lahaye, M.; Pigorini, G.; Guillon, F. Changes in Texture, Cellular Structure, and Cell Wall Composition in Apple Tissue As a Result of Freezing. Food Research International 2009, 42, 788–797.
- Rimkeeree, K.; Charoenrein, S. Effect of Cultivar and Ripening Stage on Quality and Microstructure of Frozen Mangoes (Mangifera Indica Linn.). International Journal of Food Properties 2014, 17, 1093–1108.
- Gómez, F.; Sjöholm, I. Applying Biochemical and Physiological Principles in the Industrial Freezing of Vegetables: A Case Study on Carrots. Trends in Food Science and Technology 2004, 15, 39–43.
- Varela, P.; Salvador, A.; Fiszman, S. Changes in Apple Tissue with Storage Time: Rheological, Textural, and Microstructural Analyses. Journal of Food Engineering 2007, 78, 622–629.
- Wu, J.; Guo, K.Q. Dynamic Viscoelastic Behaviour and Microstructural Changes of Korla Pear (Pyrus Bretschneideri rehd) Under Varying Turgor Levels. Biosystems Engineering 2010, 106, 485–492.
- Garcia Loredo, A.B.; Guerrero, S.N.; Gomez, P.L.; Alzamora, S.M. Relationships Between Rheological Properties, Texture, and Structure of Apple (Granny Smith var.) Affected by Blanching and/or Osmotic Dehydration. Food and Bioprocess Technology 2013, 6, 475–488.
- Ningrum, A.; Minh, N.N.; Schreiner, M. Carotenoids and Norisoprenoids as Carotenoid Degradation Products in Pandan Leaves (Pandanus Amaryllifolius Roxb.). International Journal of Food Properties 2015, 18, 1905–1914.
- Dalla Nora, C.; Müller, C.D.R.; de Bona, G.S.; de Oliveira Rios, A.; Hertz, P.F.; Jablonski, A.; de Jong, E.V.; Flôres, S.H. Effect of Processing on the Stability of Bioactive Compounds from Red Guava (Psidium Cattleyanum Sabine) and Guabiju (Myrcianthes Pungens). Journal of Food Composition and Analysis 2014, 34, 18–25.
- Fish, W.W.; Davis, A.R. The Effects of Frozen Storage Conditions on Lycopene Stability in Watermelon Tissue. Journal of Agricultural and Food Chemistry 2003, 51, 3582–3585.
- Volden, J.; Borge, G.I.A.; Bengtsson, G.B.; Hansen, M.; Thygesen, I.E.; Wicklund, T. Effect of Thermal Treatment on Glucosinolates and Antioxidant-Related Parameters in Red Cabbage (Brassica Oleracea L. ssp. Capitata f. Rubra). Food Chemistry 2008, 109, 595–605.
- Berger, M.; Küchler, T.; Maaßen, A.; Busch-Stockfisch, M.; Steinhart, H. Correlations of Carotene with Sensory Attributes in Carrots Under Different Storage Conditions. Food Chemistry 2008, 106, 235–240.
- Lu, R.; Peng, Y. Hyperspectral Scattering for Assessing Peach Fruit Firmness. Biosystems Engineering 2006, 93, 161–171.