Abstract
We used glycine betaine (5–20% w/v) for blanching green peas (100°C, 60 s), and their subsequent freezing and storage (–20°C, 90 days). Blanching after the addition of glycine betaine at ≥10% (w/v) followed by a 90 day storage period which resulted in the most desirable outcome: higher vitamin C levels, a superior green color, enhanced organoleptic quality and texture, and improved retention of peroxidase and lipoxygenase activity relative to control peas (no glycine betaine added). Microscopic characterizations of control and treated peas revealed that glycine betaine acts as a cryoprotectant which maintains cellular integrity. Glycine betaine (10% w/v) could be used commercially for production of frozen peas with better quality attributes.
INTRODUCTION
Glycine betaine (GB), a zwitterionic quaternary ammonium compound (molecular weight 117.2 g/mol), was discovered in beetroot (Beeta vulgaris) during the 18th century,[Citation1] but is biologically ubiquitous as it is found in almost all organisms. This amino acid derivative is known to act as a compatible solute that is capable of protecting cellular systems and biological tissues via its various roles in osmotic adjustment and protection of biomacromolecular systems. While GB is produced in plants as a response to abiotic stresses viz. drought, salinity, hyper-osmotic stress, and freezing,[Citation2] it is also produced in microbes.[Citation3] GB acts as an effective cryoprotectant in tomatoes and other plants as well as in bacterial cells[Citation4,Citation5] and as a thermoprotectant in Bacillus subtilis as well as other bacteria.[Citation6] Whereas the physicochemical properties and biological roles of individual compatible solutes differ,[Citation7–Citation10] GB can protect cellular systems against diverse and indeed multiple simultaneous stresses. In addition to osmotic adjustment and cryo- and thermoprotection, GB also shows kosmotropicity-induced protection against solvents, chaotropic solutes, and hydrophobic stressors.[Citation7–Citation9] Like other compatible solutes, GB has a unique and complex profile of physicochemical and biophysical activities. GB is utilized by the food, agricultural, pharmaceutical, and other industries for a variety of applications. [Citation11] Biogenic GB is commercially produced from beet molasses and marine alga Ascophyllum nodosum.[Citation12,Citation13]Acid whey, a waste material of the dairy industry, can also be fermented to produce GB.[Citation14]
Green peas are staple vegetables which are produced and consumed throughout the world.[Citation15] Seasonal variation in production has made it imperative to freeze-store peas in order to meet the gap between supply and demand at various times of year.[Citation16] Prior to long-term storage at –20°C, peas must first be blanched at a high temperature and then cooled rapidly. Failure to execute an optimized blanching methodology can lead to loss of heat-labile nutrients and a reduction in quality.[Citation17] Similarly, improper freezing protocols and some improper transportation and storage conditions during supply-chain management can promote nutrient loss and degrade quality further.[Citation18–Citation21]
GB is found in almost all food products of animal or plant origin and hence, has a generally recognized as safe (GRAS) status.[Citation22] It is present naturally at a low concentration (5 µg/g) in green peas.[Citation22] The nutraceutical benefits of GB include its use for treatment and monitoring of diseases such as homocystinuria, a genetic disorder of autosomal recessive nature;[Citation23] mitigation of hepatoxicity and neurotoxicity caused by ethanol consumption;[Citation24,Citation25] promoting healthy liver function due to its involvement in methylation reactions of metabolism which helps in the regeneration of hepatic cells;[Citation26,Citation27] and arterial degeneration; muscle wasting and variety of skin problems can also be treated or prevented using GB.[Citation28–Citation30] Supplementation of foods using GB, therefore, is not only permitted but can be desirable.
We hypothesized that GB can be used as a supplement which is able to protect against deterioration of pea quality during blanching and freezing. This was evaluated by monitoring vitamin C content, percentage alcohol-insoluble solids (AIS), green coloration, peroxidase (POD) activity, lipoxygenase (LOX) activity, texture of pea skin and cotyledons, sensory analyses, and assessment of cellular damage caused by freeze-thawing.
MATERIALS AND METHODS
Materials
Food-grade GB (≤98% [w/w]) of RUICHEM, Zhejiang, China, was used. Maltitol and trehalose used were of food grade. Freshly harvested green peas (Pisum sativum L.), still in their pods, were purchased from a street vegetable market in Mumbai, India. All the reagents and chemicals used in the study were purchased from S.D. Fine Chemicals Limited (Mumbai, India) and HiMedia Laboratories (Mumbai, India).
Pea Blanching, Preparation, and Frozen-Storage
Freshly harvested green peas were blanched in tap water (control), 5% w/v GB aqueous solution (5% GB), 10% w/v GB aqueous solution (10% GB), 15% w/v GB aqueous solution (15% GB), and 20% w/v GB aqueous solution (20% GB) were prepared using tap water and all were blanched for 60 s at 100°C.[Citation21] The ratio of peas to blanching solution was 1:2, and peas were cooled immediately after blanching by transferring to their respective blanching solutions which were pre-cooled to 4°C. A temperature of 0 to 4°C was maintained throughout the cooling period (i.e., 30 min). The cooled peas were packed into zip-lock bags which were sealed and then frozen at –20°C for 90 days. Sampling and assessment were carried out at 30-day intervals. Additional samples of fresh peas were osmotically dehydrated prior to freezing; this treatment produced “dehydrofrozen” peas.[Citation19] These dehydrofrozen peas were prepared in 56.5% (w/v) malitol + trehalose solution (with maltitol and trehalose at a ratio of 2:1 by weight),[Citation19] frozen in zip lock bags for 90 days, and were sampled after every 30 days. Raw peas which were used as a control were not exposed to any blanching treatment.
Analysis of Vitamin C and AIS
Vitamin C was determined titrimetrically by an iodometric method. Thawed pea samples (10 g) were crushed to a pulp by mortar and pestle; the pulp was dispersed in distilled water and the volume was then made up to 100 mL. This was then centrifuged at 3857 × g and supernatant was strained through a cheese cloth. Distilled water was added to 10 mL of this clear extract, making the total volume 99 mL. Starch indicator (0.5% w/v, 1 mL) was then added to make up the final volume to 100 mL. This was titrated against 0.005 M of iodine solution.[Citation31] Percent AIS was determined by a gravimetric method as reported by Nleya et al.,[Citation32] where the weight difference between the initial pea sample and the ethanol treated pea sample was determined.
LOX and POD Activity, Texture, and Color Analysis
Thawed pea samples (10 g) were pulped using a mortar and pestle, to which distilled water was added slowly but continuously to make the final volume 50 mL; this extract was strained through a cheese cloth and then centrifuged at 3857 × g. Protein content of pea samples was determined by Bradford’s method.[Citation33] LOX (234 nm) and POD (420 nm) activity was determined in the supernatant spectrophotometrically[Citation34] on a Hitachi U-2001 ultraviolet (UV)-spectrophotometer (Hitachi, Tokyo, Japan).
The force required to rupture the thawed peas was calculated using a texture analyzer (TA-XT2i, Surrey, UK) fitted with a P/2 probe (1 mm/s) which penetrated the peas up to a depth of 3 mm. The probe first ruptured the skin and then the cotyledons. Two forces were obtained from which the texture of the skin and cotyledon were evaluated (Supplementary file). The intensity of green coloration was monitored in terms of a* using Hunter Lab Colorimeter (LabScan® XE, Virginia, USA).
Sensory Evaluation
Sensory evaluation of thawed peas at room temperature (30 ± 3°C) was performed after every 30 days during the 90-day frozen-storage period, for 15 parameters essential to the perceived quality of green peas ().[Citation32,Citation35] A panel of 14 members that comprised of nine females and five males (students and employees of ICT, Mumbai) in the age group of 22–29 years, who did not smoke, performed the evaluation.
TABLE 1 Fifteen parameters essential to the perceived quality of green peas
Microscopic Analysis of Frozen Peas After a 90-Day Storage
Treated and control peas were assessed for signs of mechanical damage upon thawing using a light microscopy at × 100 magnification. The thawed peas were immediately deskinned and the cotyledons were separated. A thin transverse cotyledon section was prepared using a scalpel, placed on a grease-free glass slide.
Statistical Analyses
All the experiments were performed using a minimum of triplicates. Means and standard deviations were calculated using Microsoft Excel 2010. One-way analysis of variance (ANOVA) was performed using IBM SPSS Statistics 20.
RESULTS AND DISCUSSION
GB Enhances Pea Quality Upon Blanching
Many blanching protocols have been designed to minimize the loss of vitamin C[Citation17,Citation21,Citation34] which is one of the most heat-labile of the vitamins. Inclusion of 10 to 20% w/v GB during blanching provided considerable thermoprotection; only 21.32% of vitamin C (at 10% w/v GB) was lost compared with a 46.90% loss in peas blanched in tap water (). There was no significant effect of GB concentration in the range of 10 to 20% w/v. Blanching reduced the quantity of AIS in the peas indicating some loss of solids in the blanching solution, although inclusion of GB in blanch water did not have any effect on the AIS.
TABLE 2 Evaluation of green peas after blanching and rapid cooling in 5 to 20% (w/v) GB solution, in comparison to raw, tap water blanched, and osmo-dehydrated peas#
TABLE 3 Effect of blanching treatment and rapid cooling in GB solution on the sensory evaluation of frozen peas during 90-days storage#
POD is a heat-stable enzyme,[Citation21] whereas LOX is highly heat labile and so can be inactivated during blanching.[Citation21,Citation34,Citation36] LOX catalyzes oxygenation reactions of fatty acids and other fat-related compounds. Although high levels of LOX are undesirable due to this activity,[Citation36] lower levels of LOX can have an adverse impact on the characteristic flavor of blanched peas; LOX activity was maximum in raw peas. GB clearly provided thermoprotection to LOX (). Use of GB in the blanching water resulted in the retention of 3.34% of the initial LOX activity as compared to only 0.63% in peas blanched in water. A POD concentration in the range of 2 to 7% is recommended in blanched peas; values below 2% indicate over-blanching and values above 7% indicate under-blanching. Hence, blanching protocols for peas are designed on the basis of retention of POD activity after blanching.[Citation21] GB in the range of 10 to 20% w/v retained 7.09% POD activity as opposed to only 1.53% in peas blanched in water and 3.26% in osmo-dehydrated peas (). Peas blanched in 5% w/v GB retained similar levels of POD activity as those observed in osmo-dehydrated peas.
Blanching results in the softening of peas, but the use of GB reduced softening of the cotyledon to some extent when compared to control (). Osmotic dehydration led to hardening of peas.[Citation19] Blanching increased the intensity of green coloration as has also been previously reported.[Citation17,Citation34] There was no apparent influence of GB on the retention of green coloration during blanching.
GB Mediated Protection Against Freezing
Steady reduction of vitamin C content occurred in peas blanched in water in the 5% GB treatment, suggesting that neither water nor GB at 5% (w/v) are effective at maintaining pea quality (). However, the deterioration was found to be slowed down in peas that were blanched in 10 to 20% GB (w/v) up to 90 days of storage. Although trehalose is a well-known osmoprotectant and cryoproptectant,[Citation3,Citation4,Citation19] it provided cryoprotection against loss of vitamin C until 60 days, after which rapid deterioration in vitamin C was seen in osmo-dehydrated peas ().
FIGURE 1 Effect of different blanching treatments on the deterioration of a: vitamin C; b: green coloration; c: pea skin texture; and d: pea cotyledon texture during 90 days of storage at –20°C.
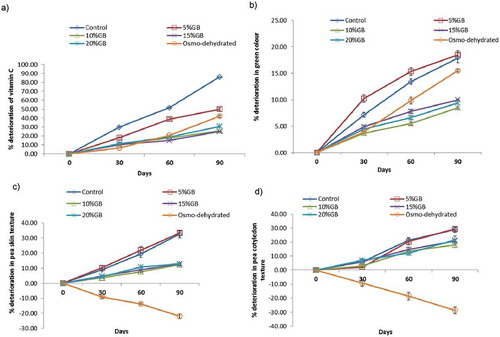
Peas in the 5% GB treatment showed rapid deterioration of green color immediately after blanching when compared to blanching in water (), an observation that was not supported by sensory observations (). All other test samples showed more-or-less consistent levels of color deterioration until 30 days (). After 30 days, the quality of osmo-dehydrated peas deteriorated rapidly, while those blanched in 10 to 20% GB (w/v) deteriorated at a considerably slower rate. These data also indicate that 10% GB (w/v) leads to better retention of green color than the osmo-dehydration treatment which utilizes the well-known cryoprotectant, trehalose (). Regardless of treatment regime, reduction of green coloration in frozen peas during storage is commonly reported as problematic.[Citation21,Citation32]
The texture of both the pea skin and cotyledons deteriorated at a faster rate in peas blanched in water or at 5% GB (w/v) than observed in peas blanched in 10 to 20% GB (w/v) during 90 days of frozen storage ( and ). For the osmo-dehydrated peas, a reverse trend was seen where the peas were found to harden rather than soften. Whereas this phenomenon was also reported by Giannakourou and Taoukis,[Citation19] the underlying mechanism has yet to be elucidated.
In raw peas, AIS are an indicator of maturity. The AIS value is indicative of the degree to which sugars have been converted in to starch during maturation of peas.[Citation32,Citation37] In the current study, the amounts of AIS were found to be comparable in all samples (). The LOX activity was reduced in all the samples during the 90-day storage period, although the extent of this reduction was influenced by the nature of blanching. The peas that had been blanched in water and in 5% GB (w/v), and the osmo-dehydrated peas, were characterized by very low LOX activity values while the peas blanched in 10 to 20% (w/v) GB showed much higher and similar values of LOX activity (). A similar trend was observed with POD activity (). These data clearly indicate that GB affords cryoprotection when used at or above 10% (w/v).
Microscopic Analysis of Peas After 90 Day Storage
There was a clear difference in the structural integrity of cells from pea samples that had been stored at –20ºC and then thawed when compared to those from fresh peas (). Cells of fresh peas appeared round, indicating a healthy turgor (), whereas peas that had been frozen and then thawed exhibited ruptured cells and were characterized by structural destruction of the tissues; this is consistent with earlier studies of freeze-thawing.[Citation4,Citation21] In the current study, peas blanched in either water (i.e., control) or in 5% GB (w/v) were characterized by a loss of cellular integrity, indicating that 5% GB (w/v) was insufficient to provide effective cryoprotection. By contrast, peas in the osmo-dehydration, 10% GB, and 20% GB treatments peas exhibited low levels of cellular damage, indicating that both GB and maltitol+trehalose can provide cryoprotection. The cryoprotectant activities of trehalose and GB is consistent with their known roles in the microbial cells.[Citation4,Citation11]
FIGURE 3 Microscopic images (x100 magnification) of fresh raw pea and test pea samples after 90 day frozen storage at –20°C.
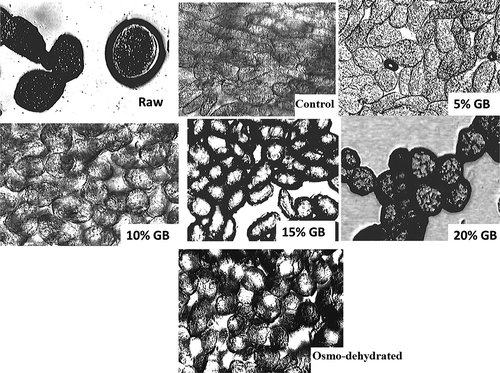
The mechanism by which GB provides cryoprotection is not yet understood. In tomatoes, exogenous application of GB is reported to improve its chilling tolerance which may be due to higher catalase activity in GB treated tomato plants[Citation5] that over express the catalase gene (CAT1). Similarly, in peas, POD activity was lower when water was used for blanching (). Hence, it appears that genes involved in aspects of the cryoprotectant activity may be activated upon treatment of the peas with GB. Further research at the molecular level is necessary to confirm these findings. Trehalose might have provided cryoprotection to peas by transforming the plant cell into glassy state when frozen as reported in microbial cells,[Citation4] a notion which is consistent with the hardening reported during texture analysis ( and ).
Sensory Analysis
Whereas GB is a tasteless, colorless, and odorless compound, the 15 parameters listed in were used to produce a holistic assessment of the overall quality of the peas after blanching and freeze-storage/thawing.[Citation32,Citation35] Osmo-dehydrated peas were perceived to be sweeter and showed more shriveling and hardness, than other test samples () as the maltitol and trehalose used during the osmotic dehydration process contributed to pea flavor. All of the other parameters assessed during the sensory analysis, including flavor, were similar .
During the 90-day storage period, shriveling and yellowing were observed in frozen peas that were blanched in either tap water or 5% GB (w/v), whereas there were no visible changes in osmo-dehydrated peas or those blanched in 10 to 20% GB (w/v). All pea samples were attributed positive scores for aroma and strength of flavor, except for osmo-dehydrated peas which were perceived to be less aromatic (). Retention of LOX activity in peas that were blanched in GB did not lead to the production of off-flavors according to organoleptic (). Hexane extracts were prepared from aqueous pea extracts to study the effect of retention of LOX activity on lipids. Three layers were formed: an upper, clear hexane layer; a middle lipoprotein-carbohydrate layer; and a lower aqueous layer. Gas chromatography–mass spectrometry (GC–MS) analysis of the upper hexane layer, however, did not yield reproducible results (data not shown); and this was presumably because much of the lipid in peas is bound either to proteins or sugars.[Citation38,Citation39] The textures of both the skin and cotyledons in osmo-dehydrated peas were altered during the 90-day storage period, a finding in accordance with that observed from texture analysis. The perceived juiciness of GB treated peas was greater than that for peas blanched in water, but was similar to that recorded for osmo-dehydrated peas at day 90. Hence, peas blanched in GB retained their quality during freeze-storage and thawing better than those blanched in water; furthermore, the former did not show hardening as seen in osmo-dehydrated peas. Osmo-dehydrated peas were perceived to be sweeter, and therefore, were preferred by the panel.
Multiplication of spoilage microorganisms has been observed down to –18ºC in frozen peas, the lowest recorded temperature at which microbial multiplication has been recorded.[Citation18,Citation40] Chaotropic substances, including the compatible solutes glycerol and fructose, can enhance flexibility of macromolecular systems at low temperature[Citation9,Citation41] and can thereby extend microbial growth windows by reducing temperature minima for multiplication.[Citation42,Citation43] Given the kosmotropicity of substances such as GB and trehalose, however, it is likely that they will have the opposite effect and thereby reduce the chance of spoilage during freeze-storage. In nature, some microbes inhabit compatible solute-rich milieu such as entomopathogenic fungi in the trehalose-rich insect haemolymph,[Citation44] and in relation to microbial biotechnology compatible solutes can be used to optimize biofuel fermentations and biological control of agricultural pests and pathogens,[Citation9,Citation45] and to produce food preservatives, body products, and cosmetics. Whereas the multifarious roles of compatible solutes in microbial cells have been relatively well-characterized, the mechanisms of action are not always clear. Osmotic adjustment is key to the ability of microbial cells coping with changes in or extremes of water activity.[Citation46–Citation48] Further work is needed to determine whether GB acts, in part at least, by preventing osmotic shock to peas during the blanching process.
CONCLUSION
The findings of the current study indicate that incorporation of 10% GB (w/v) in blanch water provided post blanching thermo- and cryo-protection to peas during frozen storage. The protocol described above can be modified for use at an industrial scale in order to improve the quality of commercially processed peas. Further studies on the protective capabilities of GB in blanch water for other fruits and vegetables may lead to additional applications of this compatible solute. The biophysical mechanism(s) underlying the protective activities of GB warrant further study.
Supplementary_file.doc
Download MS Word (73.5 KB)FUNDING
First author Jayaranjan R Kar, is grateful to University Grants Commission, India for the financial support provided for this research.
Additional information
Funding
REFERENCES
- Scheibler, C. Ueber das Betain, Eine im Safte der Zuckerru¨Ben (Beta Vulgaris) Vorkommende Pflanzenbase [About the Betaine, One in Said the Zuckerrübe¨Ben (Beta vulgaris) Occurring plant base]. Berichte der Deutschen Chemischen Gesellschaft [Reports of the German Chemical Society] 1869, 2, 292–295.
- Giri, J. Glycinebetaine and Abiotic Stress Tolerance in Plants. Plant Signaling & Behavior 2011, 6, 1746–1751.
- Severin, J.; Wohlfarth, A.; Galinski, E.A. The Predominant Role of Recently Discovered Tetrahydropyrimidines for the Osmoadaptation of Halophilic Eubacteria. Journal of General Microbiology 1992, 138, 1629–1638.
- Cleland, D.; Krader, P.; McCree, C.; Tang, J.; Emerson, D. Glycine Betaine As a Cryoprotectant for Prokaryotes. Journal of Microbiological Methods 2004, 58, 31–38.
- Park, E.J.; Jeknic, Z.; Chen, T.H.H. Exogenous Application of Glycinebetaine Increases Chilling Tolerance in Tomato Plants. Plant and Cell Physiology 2006, 47, 706–714.
- Holtmann, G.; Bremer, E. Thermoprotection of Bacillus Subtilis by Exogenously Provided Glycine Betaine and Structurally Related Compatible Solutes: Involvement of OPU Transporters. Journal of Bacteriology 2004, 186, 1683–1693.
- Bhaganna, P.; Volkers, R.J.M.; Bell, A.N.W.; Kluge, K.; Timson, D.J.; McGrath, J.W.; Ruijssenaars, H.J.; Hallsworth, J.E. Hydrophobic Substances Induce Water Stress in Microbial Cells. Microbial Biotechnology 2010, 3, 701–716.
- Cray, J.A.; Russell, J.T.; Timson, D.J.; Singhal, R.S.; Hallsworth, J.E. A Universal Measure of Chaotropicity and Kosmotropicity. Environmental Microbiology 2013, 15, 287–296.
- Cray, J.A.; Stevenson, A.; Ball, P.; Bankar, S.B.; Eleutherio, E.C.; Ezeji, T.C.; Singhal, R.S.; Thevelein, J.M.; Timson, D.J.; Hallsworth, J.E. Chaotropicity: A Key Factor in Product Tolerance of Biofuel-Producing Microorganisms. Current Opinion in Biotechnology 2015, 33, 228–259.
- Hallsworth, J.E.; Magan, N. Manipulation of Intracellular Glycerol and Erythritol Enhances Germination of Conidia at Low Water Availability. Microbiology 1995, 141, 1109–1115.
- Kar, J.R.; Singhal, R.S. Investigations on Ideal Mode of Cell Disruption in Extremely Halophilic Actinopolyspora halophila (MTCC 263) for Efficient Release of Glycine Betaine and Trehalose. Biotechnology Reports 2015, 5, 89–97.
- Heikkila, H.O.; Melaja, J.A.; Millner, D.E.D.; Virtanen, J.J. Betaine Recovery Process. US Patent 4359430, 1982.
- Whapham, C.A.; Blunden, G.; Jenkins, T.; Hankins, S.D. Significance of Betaines in the Increased Chlorophyll Content of Plants Treated with Seaweed Extract. Journal of Applied Phycology 1993, 5, 231–234.
- Kar, J.R.; Hallsworth, J.E.; Singhal, R.S. Fermentative Production of Glycine Betaine and Trehalose from Acid Whey Using Actinopolyspora halophila (MTCC 263). Environmental Technology & Innovation 2015, 3, 68–76.
- C azzato, E.; Laudadio, V.; Ceci, E.; Tufarelli, V. Effect of Sulphur Fertilization on Fatty Acid Composition of Faba Bean (Vicia faba L.), White Lupin (Lupinus albus L.), and Pea (Pisum sativum L.) Grains. Journal of Food, Agriculture, and Environment 2014, 12, 136–138.
- Mahajan, R.; Garg, S.; Sharma, P.B. Indian Frozen Peas Market: A Case Study on FPIL. International Journal of Globalisation and Small Business 2011, 4, 154–169.
- Lin, S.; Brewer, M.S. Effects of Blanching Method on the Quality Characteristics of Frozen Peas. Journal of Food Quality 2005, 28, 350–360.
- Collins, M.A.; Buick, R.K. Effect of Temperature on the Spoilage of Stored Peas by Rhodotorula glutinis. Food Microbiology 1989, 6, 135–141.
- Giannakourou, M.C.; Taoukis, P.S. Stability of Dehydrofrozen Green Peas Pretreated with Nonconventional Osmotic Agents. Journal of Food Science 2003, 68, 2002–2010.
- Green, K.; Foster, C. Transformations in Food Consumption and Production Systems: The Case of the Frozen Pea. In Industrial Ecology and Spaces of Innovation; Green, K.; Randles, S.; Eds.; Edward Elgar Publishing: Northhampton, MA, 2006; pp. 131–152.
- Sun, D.W. Handbook of Frozen Food Processing and Packaging, Contemporary Food Engineering; Taylor & Francis: Boca Raton, FL, 2012.
- De Zwart, F.J.; Slow, S.; Payne, R.J.; Lever, M.; George, P.M.; Gerrard, J.A.; Chambers, S.T. Glycine Betaine and Glycine Betaine Analogues in Common Foods. Food Chemistry 2003, 83, 197–204.
- Schwab, U.; Törrönen, A.; Toppinen, L.; Alfthan, G.; Saarinen, M.; Aro, A.; Uusitupa, M. Betaine Supplementation Decreases Plasma Homocysteine Concentrations But Does Not Affect Body Weight, Body Composition, Or Resting Energy Expenditure in Human Subjects. The American Journal of Clinical Nutrition 2002, 76, 961–967.
- Barak, A.J.; Beckenhauer, H.C.; Tuma, D.J. Betaine, Ethanol, and the Liver: A Review. Alcohol 1996, 13, 395–398.
- Kanbak, G.; Arslan, O.C.; Dokumacioglu, A.; Kartkaya, K.; Inal, M.E. Effects of Chronic Ethanol Consumption on Brain Synaptosomes and Protective Role of Betaine. Neurochemical Research 2008, 33, 539–544.
- Kim, Y.C. Liver Regeneration Accelerator Comprising Betaine. US Patent 20120172436, 2012.
- Lever, M.; Slow, S. The Clinical Significance of Betaine, An Osmolyte with a Key Role in Methyl Group Metabolism. Clinical Biochemistry 2010, 43, 732–744.
- Jutila, K. Use of Betaine. US Patent 20110196036, 2011.
- Messadek, J. Therapeutic Treatment. US Patent 20100210608, 2010.
- Peters, J.A.C.; van Norren, K.; Gorselink, M.; Hageman, R.J.J. Nutrition Comprising Betaine Against Muscle Wasting. US Patent WO2007043857, 2008.
- Silva, C.R.; Simoni, J.A.; Collins, C.H.; Volpe, P.L.O. Ascorbic Acid As a Standard for Iodometric Titrations. An Analytical Experiment for General Chemistry. Journal of Chemical Education 1999, 76, 1421.
- Nleya, K.M.; Minnaar, A.; de Kock, H.L. Relating Physico-Chemical Properties of Frozen Green Peas (Pisum sativum L.) to Sensory Quality: Sensory and Physico-Chemical Properties of Frozen Green Peas. Journal of the Science of Food and Agriculture 2014, 94, 857–865.
- Bradford, M.M. A Rapid and Sensitive Method for the Quantitation of Microgram Quantities of Protein Utilizing the Principle of Protein-Dye Binding. Analytical Biochemistry 1976, 72, 248–254.
- Gökmen, V.; Savaş Bahçeci, K.; Serpen, A.; Acar, J. Study of Lipoxygenase and Peroxidase As Blanching Indicator Enzymes in Peas: Change of Enzyme Activity, Ascorbic Acid, and Chlorophylls During Frozen Storage. LWT–Food Science and Technology 2005, 38, 903–908.
- Sanford, K.A.; Gullett, E.A.; Roth, V.J. Optimization of the Sensory Properties of Frozen Peas by Principal Components Analysis and Multiple Regression. Canadian Institute of Food Science and Technology Journal 1988, 21, 174–181.
- Gökmen, V.; Savaş Bahçeci, K.; Acar, J. Characterization of Crude Lipoxygenase Extract from Green Pea Using a Modified Spectrophotometric Method. European Food Research and Technology 2002, 215, 42–45.
- Martinez, C.; Ros, G.; Periago, M.J.; Lopez, G.; Ortuno, J.; Rincon, F. Physico-Chemical and Sensory Quality Criteria of Green Beans (Phaseolus vulgaris, L.). Lebensmittel-Wissenschaft und-Technologie 1995, 28, 515–520.
- Pont Lezica, R.; Romero, P.A.; Hopp, H.E. Glucosylation of Membrane-Bound Proteins by Lipid-Linked Glucose. Planta 1978, 140, 177–183.
- Staneloni, R.J.; Tolmasky, M.E.; Petriella, C.; Leloir, L.F. Transfer of Oligosaccharide to Protein from a Lipid Intermediate in Plants. Plant Physiology 1981, 68, 1175–1179.
- Rummel, J.D.; Beaty, D.W.; Jones, M.A.; Bakermans, C.; Barlow, N.G.; Boston, P.J.; Chevrier, V.F.; Clark, B.C.; de Vera, J.P.P.; Gough, R.V.; Hallsworth, J.E.; Head, J.W.; Hipkin, V.J.; Kieft, T.L.; McEwen, A.S.; Mellon, M.T.; Mikucki, J.A.; Nicholson, W.L.; Omelon, C.R.; Peterson, R.; Roden, E.E.; Sherwood, L.B.; Tanaka, K.L.; Viola, D.; Wray, J.J. A New Analysis of Mars “Special Regions:” Findings of the Second MEPAG Special Regions Science Analysis Group (SR-SAG2). Astrobiology 2014, 14, 887–968.
- Ball, P.; Hallsworth, J.E. Water Structure and Chaotropicity: Their Uses, Abuses, and Biological Implications. Physical Chemistry Chemical Physics 2015, 17, 8297–8305.
- Chin, J.P.; Megaw, J.; Magill, C.L.; Nowotarski, K.; Williams, J.P.; Bhaganna, P.; Linton, M.; Patterson, M.F.; Underwood, G.J.C.; Mswaka, A.Y.; Hallsworth, J.E. Solutes Determine the Temperature Windows for Microbial Survival and Growth. Proceedings of the National Academy of Sciences 2010, 107, 7835–7840.
- Williams, J.P.; Hallsworth, J.E. Limits of Life in Hostile Environments: No Barriers to Biosphere Function? Environmental Microbiology 2009, 11, 3292–3308.
- Lievens, B.; Hallsworth, J.E.; Pozo, M.I.; Belgacem, Z.B.; Stevenson, A.; Willems, K.A.; Jacquemyn, H. Microbiology of Sugar-Rich Environments: Diversity, Ecology, and System Constraints: Microbiology of Sugar-Rich Environments. Environmental Microbiology 2015, 17, 278–298.
- Hallsworth, J.E.; Magan, N. Improved Biological Control by Changing Polyols/Trehalose in Conidia of Entomopathogens. Brighton Crop Protection Conference—Pests and Diseases 1994, 3, 1091–1096.
- Alves, F.L.; Stevenson, A.; Baxter, E.; Gillion, J.L.M.; Hejazi, F.; Morrison, I.E.; Prior, B.A.; McGenity, T.J.; Rangel, D.E.N.; Magan, N.; Timmis, K.N.; Hallsworth, J.E. Concomitant Osmotic and Chaotropicity-Induced Stresses in Aspergillus wentii: Compatible Solutes Determine the Biotic Window. Current Genetics 2015, 61, 457–477.
- Hohmann, S. Osmotic Stress Signaling and Osmoadaptation in Yeasts. Microbiology and Molecular Biology Reviews 2002, 66, 300–372.
- Stevenson, A.; Cray, J.A.; Williams, J.P.; Santos, R.; Sahay, R.; Neuenkirchen, N.; McClure, C.D.; Grant, I.R.; Houghton, J.D.; Quinn, J.P.; Timson, D.J.; Patil, S.V.; Singhal, R.S.; Antón, J.; Dijksterhuis, J.; Hocking, A.D.; Lievens, B.; Rangel, D.E.N.; Voytek, M.A.; Gunde-Cimerman, N.; Oren, A.; Timmis, K.N.; McGenity, T.J.; Hallsworth, J.E. Is There a Common Water-Activity Limit for the Three Domains of Life? The International Society for Microbial Ecology Journal 2015, 9, 1333–1351.