Abstract
The aim was to develop an enzyme immobilization method for Rhizopus oryzae lipase to improve its acidolysis activity and stability. Lipase was adsorbed into NKA-9 resin and then crosslinked with glutaraldehyde as a crosslinker. The optimum conditions obtained using the response surface methodology were as follows: 34.6 mg lipase/100 mg support, 4.1 h of adsorption time, 45°C, pH 6.8, 0.5% glutaraldehyde concentration, and 2.5 h of crosslinking time. The acidolysis activity of the immobilized lipase was 31.78%, the free lipase activity was only 11.02%. The immobilized lipase showed better performance, such as higher acidolysis activity, better pH tolerance and temperature stability, enhanced storage stability, and improved reusability.
INTRODUCTION
Lipases (triacylglycerol acyl hydrolases EC 3.1.1.3) are hydrolases that act on glyceric ester bonds.[Citation1] Lipases are one of the most prevalent industrial enzymes and have been widely used in various industries, especially the food industry. Lipase-catalyzed reactions include hydrolysis, alcholysis, acidolysis, esterification, inter-esterification, and aminolysis.[Citation2] In particular, lipases with 1,3-positional selectivity play an important role in synthesizing 1,3-diacylglycerol and structured lipids.[Citation3]
However, free lipase is expensive, exhibits poor stability and lower organic solvent tolerance, is difficult to recover, and cannot be reused. To solve these problems, lipase has been immobilized using all types of methods, including adsorption,[Citation4] entrapment,[Citation5] covalent binding,[Citation6] and crosslinking.[Citation7] Among these methods, adsorption is most widely used for immobilization because lipase activity is high and the cost is low. However, the connecting force between the carrier and lipase is weak; therefore, it is easy for the lipase to fall off of the carrier during the catalytic progress, and lipase immobilized by adsorption is relatively difficult to reuse.[Citation8] Therefore, it is important to improve the stability of the immobilized lipase. Treating with a crosslinker can solve these problems. In this study, glutaraldehyde was used as a crosslinker because it can improve the interactions between the lipase and carrier.[Citation9]
Immobilization is used to improve hydrolytic and esterification activity in general. However, few studies have considered means for improving lipase acidolysis activity. An acidolysis reaction involves an acyl transfer between a triglyceride and fatty acid with lipase as the catalyst, and the triglyceride is transformed.[Citation10] The acidolysis reaction is important in oil modification and preparing functional lipids. Canola oil and caprylic acid were used as the reaction substrate and lipase was used to catalyze acidolysis of the substrate and produce structured lipids.[Citation11] It is meaningful to improve lipase acidolysis activity.
Immobilized lipases are widely used in the food industry. Chenodeoxycholic esters preparation by immobilized Rhizopus oryzae lipase and synthesis of monoacylglycerol rich in polyunsaturated fatty acids from tuna oil using immobilized lipase AK.[Citation12,Citation13] In this article, lipase was first adsorbed into NKA-9 resin and then crosslinked with glutaraldehyde as a crosslinker. The single method and response surface methodology (RSM) were used to optimize the lipase immobilization conditions. Simultaneously, the free and immobilized lipases’ thermal stability, pH tolerance and storage stability were studied. Further, the reusability of the immobilized lipase was also investigated. In addition, the 1,3-positional selectivities of the immobilized and free lipase were also studied.
MATERIALS AND METHODS
Materials
Rhizopus oryzae lipase (powder, ≥30 U/mg) and 1,3-dioleoyl 2-palmitoyl triglyceride (OPO) were purchased from Sigma-Aldrich (Santa Clara, CA, USA). NKA-9 resin was obtained from the Bo-hong Resin Company (Tianjin, China). Lard oil was purchased from the Zheng-yi Food Company (Harbin, China). Caprylic acid and other organic reagents were obtained from the Ke-mio Chemical Reagent Company (Tianjin, China). The chemicals were analytical and high-performance liquid chromatography (HPLC) grade (GR) and were acquired from the Tian-li Chemical Reagent Company (Tianjin, China).
Preparation of Supports
The pretreatment for NKA-9 resin was prepared. The resins were first submerged into ethanol (95%, v/v) for 24 h, rinsed with deionized water, treated with HCl (5%, w/v) for 4 h and NaOH (2%, w/v) for 4 h,[Citation9] rinsed with deionized water until neutral, and then dried at 37°C for 15 h. The final carriers were stored at 4°C. The resins were added to an appropriate quantity of phosphate buffer solution (PBS, pH 6.8) for 20 min prior to use.
Lipase Immobilization
Lipase solution (20–40 mg/mL PBS, 1 mL) and NKA-9 resin solution (50 mg/mL, 2 mL) were mixed in a test tube with a plug (10 mL); the pH of the PBS was 4.0–8.0, which was subsequently reacted at different temperatures (39–47°C) for a specific time period (3–5 h). Simultaneously, the mixture was shaken on an oscillator every 10 min. Next, 3 mL of glutaraldehyde solution (0.25–1.0%, w/v) was added to the system and reacted for a specific contact time (1.5–3.5 h). The immobilized lipase was then separated using a vacuum filter, during which deionized water was used to rinse the lipase several times, which was finally stored at 4°C until use. The RSM was used to optimize the immobilization conditions.[Citation14]
Lipase Activity Determination
Protein content was estimated by the method of Bradford.[Citation15] In this experiment, lipase activity refers as to acidolysis activity. The lard oil and caprylic acid were used as substrates and were used with the lipase-catalyzed acidolysis reaction. Lard oil (150 μL) was mixed with caprylic acid (50 μL), the mixture was shaken on an oscillator for 1 min, and 10 μg of free or immobilized lipase was added to the mixture for an enzymatic reaction at 45°C for 2 h.[Citation11,Citation16] The lipase was removed from the reaction mixture after centrifugation at 8000 × g for 3 min. One hundred microliters of the final reaction mixture was dissolved in n-hexane (1 mL, AR). Taking out 100 μL of the above the mixture for silica gel plate chromatography separation using a mixture composed of petroleum ether, ether and acetic acid (40:10:1) as developing solvents. We scraped the diacylglycerol and triacylglycerol stripe under 560 nm ultraviolet radiation after separation.
The scraped sample was methylated using the boron trifluoride-methanol method.[Citation11] A potassium hydroxide (KOH)-methanol solution (2 mL, 0.5 mol/L) was added to the sample, mixed, saponified in a 70°C water bath for 10 min, and then cooled to room temperature. We then added 3 mL of boron trifluoride diethyl etherate and reacted the sample for another 5 min under the same temperature conditions, and the sample continued to cool. Thereafter, n-hexane (2 mL, AR) and then 1 mL saturated sodium chloride were added and absorbed the upper organic phase, which was stored at –18°C for gas chromatography (GC) analysis. We used a GC system (HP 7890A) equipped with a flame ionization detector (FID) detector and a fused silica capillary column (100 m × 0.25 mm × 0.20 μm) to analyze the fatty acid composition. The initial column temperature was 140°C, which was maintained for 5 min, after which the temperature increased to 240°C at 4°C/min and was maintained for 15 min. The temperature of the injection port was 240°C, and the detector temperature was 10°C higher. The remaining conditions were as follows: He was the carrier gas with a 20 mL/min flow-rate; a mixture of H2 and air was the fuel gas with a 40 mL/min flow-rate; the split ratio was 50:1, and the quantity of sample injected was 1 μL. The quantity of caprylic acid among the fatty acid products was calculated using the area normalization method.
Acidolysis activity (A) was calculated using Eq. (1):
where Ci is the initial amount of caprylic acid minus the amount of this acid in the reaction mixture, and C0 is the initial quantity of caprylic acid added.
Effect of Temperature and pH on Free and Immobilized Lipase Stability
The free and immobilized lipases were first stored for 1 h at 35, 40, 45, 50, 55, 60, 65, and 70°C, the pH of PBS was 6.8; the lipase activity was measured at each temperature, and thermal stability results for the lipase were acquired. The lipase activity was evaluated using the method described above. The activity at the optimum temperature was considered 100%. The lipase was first incubated in PBS at different pH values (pH of 4.0, 5.0, 6.0, 7.0, 8.0, and 9.0) for 1 h at 45°C, and the pH tolerance was analyzed based on the lipase activity under different conditions. The lipase activity was measured using the above method. The activity at the optimum pH was considered 100%.
Storage Stability of the Free and Immobilized Lipase
The storage stability of the free and immobilized lipase was studied. These two types of lipases were first stored for a period of days under the following storage conditions: 4°C and PBS at pH 6.8. During this time, the lipase activity was measured every 5 days until the lipase activity was 50% of the initial activity.[Citation17]
The Reusability of the Immobilized Lipase
The reusability of the immobilized lipase was evaluated over several successive cycles until the activity was 50% of the initial activity. The residual activity was determined after each cycle. The immobilized lipase was removed using centrifugation (8000 × g, 3 min) at the end of each catalytic cycle.
The 1,3-positional Selectivities of the Free and Immobilized Lipases
The 1,3-positional selectivities of the free and immobilized lipases were determined by catalyzing OPO hydrolysis. First, 100 μL of OPO was added to 1 mL PBS (pH 6.8), and 10 mg immobilized lipase was added for the enzymatic reaction at 45°C for 30 min. Next, centrifugation (8000 × g, 3 min) was used for silica gel plate chromatography separation, and scraping was used for the free fatty acids stripe under 560 nm ultraviolet radiation. Methyl-esterification was used to prepare for the GC analysis as previously described. The percentage of the 1,3-positional oleic acid among the 1, 2, 3-positional fatty acids was calculated using the area normalization method. We calculated 1,3-positional selectivity (S) using Eq. (2):
where Bi is the quantity of oleic acid obtained using the hydrolysis reaction, and B0 is the total quantity of hydrolyzed oleic acid and palmitic acid.
RESULTS AND DISCUSSION
Obvious Impact Factors on Lipase Immobilization
The Plackett-Burman design was used to reduce the number of experiments and decrease the experimental time to optimize the immobilization conditions. The variables used in this study were immobilization pH, quantity of lipase added, adsorption time, crosslinking time, concentration of glutaraldehyde, and temperature maintained at 45°C during the immobilization process. The optimum value of each factor was previously confirmed after a preliminary experiments. Using the five factors’ optimum valve and considering it the central point, each factor was evaluated at a high (+1), a low (–1), and a central (0) level. All five factors were assigned two levels for eight experiments to validate the clear impact factors on lipase immobilization.[Citation18] Lipase activity was used as the response valve. The experiment design and lipase activity results are shown in .
Table 1 Plackett-Burman design and its responses
shows the variance analysis (ANOVA) of the obvious impact factor experimental results. The factors lipase addition, adsorption time and pH exhibit significant (p < 0.05); however, the crosslinking time and glutaraldehyde concentration were not significant (p > 0.05). These data indicate that the lipase added, the adsorption time, and the pH are associated with lipase activity, and any minor change in these variables from the zero level value may influence the lipase activity considerably. As crosslinking time and glutaraldehyde concentration did not significantly influence the lipase activity, these factors should be neglected in follow-up experiments. Therefore, the lipase added, the adsorption time and the pH were used as the main factors for further optimization to obtain the best conditions for lipase immobilization.
Table 2 Variance analysis for Plackett-Burman design
Optimization of Conditions for Lipase Immobilization Using RMS
Based on the Plackett-Burman experiment results, the lipase added, the adsorption time, and the pH were used as independent variables for the RSM, and each factor was evaluated at a high (+1), a low (–1), and a central (0) level. Based on the Box-Benhnken central composite design, all three factors were used in 15 experiments with 12 experimental runs and three center point runs. The specific experiment design and response value results are detailed in . The experimental data were analyzed using Design-Expert 7.0, which provide results from a polynomial regression equation and ANOVA. The fitted quadratic equation obtained is as follows:
Table 3 Box-Behnken design and its responses
where Y means the lipase activity, A is the quantity of lipase added, B is adsorption time, and C is the pH of the lipase solution. Using the ANOVA to evaluate the adequacy of the fitted model, the p-value was used as the index (). According to the ANOVA results, a lack of fit is not significant (p > 0.05), but the model is significant (p < 0.0001), and the RCitation2 value obtained approached 1.00 (RCitation2 = 0.9933). These data demonstrate the adequacy and credibility of the model, that the response can be predicted and that the model can explain the relationship between the lipase activity and variables. The p-values of the factors showed that the model terms B, C, ACitation2, BCitation2, and CCitation2 were extremely significant (p < 0.01), that the interacting terms AB and BC were significant (p < 0.05), and that the other terms were not significant. For the variables, the adsorption time exhibited the greatest impact on the lipase activity, the pH exhibited the second greatest influence, and the lipase added exhibited the least importance.
Table 4 Test of significance for regression coefficient
The three-dimensional response curve is shown in , which illustrates the interactive effect of the factors on lipase activity. shows that the interaction between the lipase added and the adsorption time on lipase activity is significant. When the pH is maintained, the lipase activity first increased with an increase in adsorption time, and the maximum value was reached near 4.0 h; thereafter, with an increase in adsorption time, the lipase activity was lower. This phenomena is due to greater lipase accumulation with an increase in adsorption time, which may inhibit the substrate contact with the lipase active site and, consequently, influence lipase activity. Compared with the remaining two factors, the lipase added exhibited the lowest impact on lipase activity (p > 0.05). Lipase added at 34–35 mg/100 mg support can provide suitable catalytic power but not steric hindrance of the lipase active site due to a high concentration of lipase.[Citation19]
FIGURE 1 Response curve for the immobilized lipase activity A: as an interaction between lipase added and adsorption time, B: as an interaction between lipase added and pH, and C: as an interaction between adsorption time and pH. Immobilized temperature 45°C, pH 4–8, adsorption time 3–5 h, lipase added at 20–40 mg/100 mg carrier, glutaraldehyde concentration 0.5%, and crosslinking time 2.5 h.
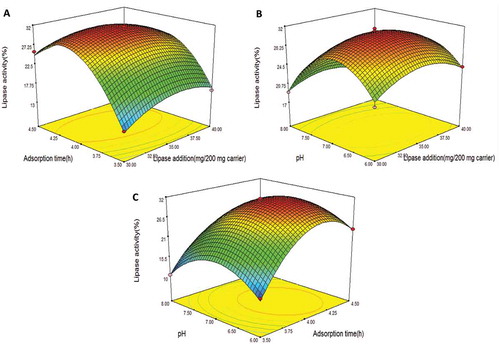
The interaction between the lipase added and the pH is shown in . The interaction between AC is not significant to the response (p > 0.05). When the adsorption time was constant, the lipase activity first increased when the pH of the lipase solution increased, but then it then decreased. The highest activity was obtained at approximately a pH of 7.0. A similar result was observed with the Aspergillus niger lipase immobilized on resin.[Citation20]
The interaction between the adsorption time and pH significantly influenced lipase activity, which is illustrated in . The lipase activity increased with an increase in adsorption time and pH until reaching an optimum value; thereafter, an additional increase in the two factors may decrease the lipase activity. These observations indicate that an extreme pH and adsorption time negatively influence lipase activity. Some researchers investigated the effects of the reaction temperature and time on the immobilization of lipase on the silica gel matrix, and found similar change with our result.[Citation21]
After the optimization experiment for lipase immobilized on NKA-9 resin, the best conditions were as follows: temperature 45°C, pH 6.8, lipase added at 34.6 mg/100 mg support, adsorption time 4.1 h, glutaraldehyde concentration 0.5%, and crosslinking time 2.5 h. The optimum lipase acidolysis activity was 31.80%. The average of acidolysis activity for the validation experiment under the optimum conditions was 31.78%, which only differs from the regression analysis-predicted value by 0.02%. These data indicate that the experimental value and predicted value match, and the conditions determined by the RSM are accurate and reliable.[Citation22]
Thermal Stability of Free and Immobilized Lipases
The results show that the optimum temperature of the lipase shifted from 40 to 45°C after immobilization in . Further, the immobilized lipase exhibited better activity at a higher temperature than the free lipase. When the temperature reaches up to 70°C, 31.81% of the activity was retained by the immobilized lipase, and the free lipase was deactivated. These data indicate that immobilization positively effects the lipase thermal stability. This phenomenon is due to the more rigid external backbone for lipase molecules generated through immobilization and crosslinking;[Citation23] the high temperature has less influence on the lipase activity, which increases thermal stability.
FIGURE 2 Physicochemical properties of the immobilized lipase. Thermal stability of free and immobilized Rhizopus oryzae lipase. The lipase was incubated for 1 h at a temperature range from 35 to 70°C in PBS (pH 6.8). The thermal stability was determined by measuring the lipase activity under each condition (A). The pH stability of the free and immobilized Rhizopus oryzae lipase. The lipase was incubated for 1 h at 45°C in PBS at the pH range from 4.0 to 9.0. The activity under each condition was measured (B). The storage stability of the free and immobilized Rhizopus oryzae lipase. The lipases were stored at 4°C in PBS (pH 6.8). The storage stability was tested by measuring the activity every 5 days until the activity was 50% of the initial activity (C). Reusability tests for the immobilized Rhizopus oryzae lipase. The reusability was evaluated by catalyzing several successive cycles. The residual activity was determined until the activity was 50% of the initial activity (D).
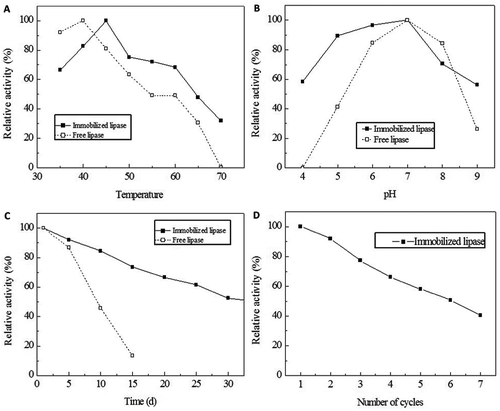
pH Stability of the Free and Immobilized Lipases
The results show that the optimum pH of the immobilized lipase was 7.0, which is the same as the free lipase in . However, the immobilized lipase exhibited higher acidolysis activity, and the pH tolerance range is wider. The relative activities of immobilized lipase at the pH range 4.0–9.0 were greater than 55%. The free lipase presented steady at pH range of 6.0–8.0; when the pH reached 4.0, the free lipase was deactivated. Some researchers found similar result that the free enzyme remained stable in the pH range from 3 to 6, while, the immobilized lipases were found to be stable up to a pH of 7.0.[Citation24]
Storage Stability of Free and Immobilized Lipases
The change in activity of the free and immobilized lipases during storage is shown in . The immobilized lipase exhibits better storage stability than the free lipase; a previous study produced similar results.[Citation25,Citation26] At a storage time of 15 days, the relative activity of the immobilized lipase was 73.5% compared with 13.5% for free lipase. After immobilization, the interaction between the carrier and lipase is stronger, which can inhibit detachment of the lipase molecule, thus resulting in better storage stability.[Citation27]
The Reusability of the Immobilized Lipase
The reusability of the immobilized lipase is detailed in . At the end of each cycle, the residual activity was measured. The immobilized lipase showed a relatively high catalytic activity; the relative activity was 50.83% after six consecutive cycles, and decreased below 50% after the seventh cycle. The decrease in activity may be attributed to lipase leaching.
1,3-positional Selectivity of Free and Immobilized Lipase
The 1,3-positional selectivities of the free and immobilized lipase are, respectively, 71.47 and 70.28%. The relative selectively of the immobilized lipase is as high as 98.3%. For the lipase, we observed almost no loss in 1,3-positional selectivity after immobilization, which indicates that the immobilized method applied in this study has little influence on the lipase’s 1,3-positional selectivity, and the lipase exhibits a high value for future applications.
CONCLUSIONS
The Rhizopus oryzae lipase was immobilized by an adsorption-crosslinking method to improve its acidolysis activity. The NKA-9 resin was used as a support, and glutaraldehyde was used as a crosslinker. The optimum acidolysis activity of the immobilized lipase obtained under optimum conditions was 31.78%, which was 11.02% better than the free lipase. After immobilization, the lipase exhibited better thermal stability, pH tolerance, and storage stability; even the reusability was enhanced. In addition, the 1,3-positional selectively of the immobilized lipase was as high as 98.3% compared with the free lipase. These data demonstrate that this method may be applied in oil modification and functional lipid preparation as well as in oil and fatty acid reaction catalysis fields.
FUNDING
This work was supported by “The Twelfth Five-Year-Plan” in National Science and Technology for The Rural Development in China (2011BAD09B0304), the National Natural Science Foundation of China (Grant No. 31201397, 31301519), the Natural Science Foundation of Heilongjiang Province of China (C201134), the Open Research Fund for Key Laboratory of Dairy Science, Ministry of Education in China (2012KLDSOF-06), and the postdoctoral science-research developmental foundation of Heilongjiang province (LBH-Q13029).
Additional information
Funding
REFERENCES
- Fan, Y.X.; Qian, J.Q. Lipase Catalysis in Ionic Liquids/Supercritical Carbon Dioxide and Its Applications. Journal of Molecular Catalysis B-Enzymatic 2010, 66, 1–7.
- Liu, J.; Zhang, Y. Optimisation of Lipase Production by a Mutant of Candida antarctica DSM-3855 Using Response Surface Methodology. International Journal of Food Science and Technology 2011, 46, 695–701.
- Lee, K.T.; Akoh, C.C. Immobilized Lipase-Catalyzed Production of Structured Lipids with Eicosapentaenoic Acid at Specific Positions. Journal of the American Oil Chemists Society 1996, 73, 611–615.
- Fernandez-Lafuente, R.; Armisén, P.; Sabuquillo, P.; Fernández-Lorente, G.M.; Guisán, J. Immobilization of Lipases by Selective Adsorption on Hydrophobic Supports. Chemistry and Physics of Lipids 1998, 93, 185–197.
- Sagiroglu, A.; Telefoncu, A. Immobilization of Lipases on Different Carriers and Their Use in Synthesis of Pentyl Isovalerates. Preparative Biochemistry and Biotechnology 2004, 34, 169–178.
- Chang, S.W.; Shaw, J.F.; Yang, K.H.; Chang, S.F.; Shieh, C.J. Studies of Optimum Conditions for Covalent Immobilization of Candida rugosa Lipase on Poly(Glutamic Acid) by RSM. Bioresource Technology 2008, 99, 2800–2805.
- Erdemir, S.; Sahin, O.; Uyanik, A.; Yilmaz, M. Effect of the Glutaraldehyde Derivatives of Calix Arene As Cross-Linker Reagents on Lipase Immobilization. Journal of Inclusion Phenomena and Macrocyclic Chemistry 2009, 64, 273–282.
- Zhang, B.; Weng, Y.; Xu, H.; Mao, Z. Enzyme Immobilization for Biodiesel Production. Applied Microbiology and Biotechnology 2012, 93, 61–70.
- Wang, W.; Jiang, Y.; Zhou, L.; Gao, J. Comparison of the Properties of Lipase Immobilized onto Mesoporous Resins by Different Methods. Applied Biochemistry and Biotechnology 2011, 164, 561–572.
- Friedman, H.I.; Nylund, B. Intestinal Fat Digestion, Absorption, and Transport. A Review. The American Journal of Clinical Nutrition 1980, 33, 1108–1139.
- Wang, Y.; Xia, L.; Xu, X.; Xie, L.; Duan, Z. Lipase-Catalyzed Acidolysis of Canola Oil with Caprylic Acid to Produce Medium-, Long- and Medium-Chain-Type Structured Lipids. Food and Bioproducts Processing 2012, 90, 707–712.
- Quintana, P.G.; Canet, A.; Marciello, M.; Valero, F.; Palomo, J.M.; Baldessari, A. Enzyme-Catalyzed Preparation of Chenodeoxycholic Esters by An Immobilized Heterologous Rhizopus oryzae Lipase. Journal of Molecular Catalysis B: Enzymatic 2015, 118, 36–42.
- Pawongrat, R.; Xu, X.; H-Kittikun,A Synthesis of Monoacylglycerol Rich in Polyunsaturated Fatty Acids from Tuna Oil With Immobilized Lipase AK. Food Chemistry 2007, 104, 251–258.
- Kumar, P.; Mishra, H.N. Optimization of Mango Soy Fortified Yogurt Formulation Using Response Surface Methodology. International Journal of Food Properties 2003, 3, 499–517.
- Jafary, F.; Kashanian, S.; Sharieat, S.Z.S. Purification, Immobilization, and Characterization of Bovine Lactoperoxidase. International Journal of Food Properties 2013, 4, 905–916.
- Hari, K.S.; Sattur, A.P.; Karanth, N.G. Lipase-Catalyzed Synthesis of Isoamyl Isobutyrate-Optimization Using a Central Composite Rotatable Design. Process Biochemistry 2001, 37, 9–16.
- Foresti, M.L.; Ferreira, M.L. Analysis of the Interaction of Lipases with Polypropylene of Different Structure and Polypropylene-Modified Glass Surface. Colloids and Surfaces A-Physicochemical and Engineering Aspects 2007, 294, 147–155.
- Rastogi, N.K.; Rashmi, K.R. Optimization of Enzymatic Liquefaction of Mango Pulp by Response Surface Methodology. European Food Research and Technology 1999, 20, 57–62.
- Bussamara, R.; Dall’Agnol, L.; Schrank, A.; Fernandes, K.F.; Vainstein, M.H. Optimal Conditions for Continuous Immobilization of Pseudozyma hubeiensis (Strain HB85A) Lipase by Adsorption in a Packed-Bed Reactor by Response Surface Methodology. Enzyme Research 2012, 2012, Article ID 329178. Retrieved from http://dx.doi.org/10.1155/2012/329178
- Gonzalez-Saiz, J.M.; Pizarro, C. Polyacrylamide Gels As Support for Enzyme Immobilization by Entrapment. Effect of Polyelectrolyte Carrier, pH, and Temperature on Enzyme Action and Kinetics Parameters. European Polymer Journal 2001, 37, 435–444.
- Lee, D.H.; Park, C.H.; Yeo, J.M.; Kim, S.K. Lipase Immobilization on Silica Gel Using a Cross-Linking Method. Journal of Industrial and Engineering Chemistry 2006, 12, 777–782.
- MuÈller, R.H.; MaÈder, K.; Gohla, S. Solid Lipid Nano-Particles(SLN) for Controlled Drug Delivery—A Review of the State of the Art. European Journal of Pharmaceutics and Biopharmaceutics 2000, 50, 161–177.
- Nawani, N.; Singh, R.; Kaur, J. Immobilization and Stability Studies of a Lipase from Thermophilic bacillus sp: The Effect of Process Parameters on Immobilization of Enzyme. Electronic Journal of Biotechnology 2006, 9, Retrieved from http://dx.doi.org/10.2225/vol9-issue5-fulltext-9
- Chiou, S.H.; Wu, W.T. Immobilization of Candida Rugosa Lipase on Chitosan with Activation of the Hydroxyl Groups. Biomaterials 2004, 25,197–204.
- Dong, H.; Li, J.; Li, Y.; Hu, L.; Luo, D. Improvement of Catalytic Activity and Stability of Lipase by Immobilization on Organobentonite. Chemical Engineering Journal 2012, 181, 590–596.
- Pandya, P.H.; Jasra, R.V.; Newalkar, B.L.; Bhatt, P.N. Studies on the Activity and Stability of Immobilized α-Amylase in Ordered Mesoporous Silicas. Microporous and Mesoporous Materials 2005, 77, 67–77.
- Mosafa, L.; Moghadam, M. Shahedi M., Papain Enzyme Supported on Magnetic Nanoparticles: Preparation, Characterization, and Application in the Fruit Juice Clarification. Chinese Journal of Catalysis Journal 2013, 34, 1897–1904.