Abstract
Dietary phenolics have remarkable attention as potential anti-inflammatory agents. This study investigated cyclooxygenase inhibitory activity of nutraceuticals isolated from hydroalcohol extract of Corchorus olitorius L. (Co) and Vitis vinifera L. (Vv). Molecular docking calculations using AutoDock Vina was used to evaluate the binding mode of these nutraceuticals and its major derivatives in the active site of both cyclooxygenase enzymes. In vitro studies showed that quercetin derivatives exhibit a recognizable selective inhibition of cyclooxygenase-II (60%) compared to cyclooxygenase-I (37%). Results showed quercetin ability to explore extra hydrophobic pocket present in the structure of cyclooxygenase-II enzyme, thus explaining its potential selectivity toward cyclooxygenase-II.
INTRODUCTION
Cyclooxygenase (COX) enzyme; also known as prostaglandin-endoperoxide synthase (PTGS); catalyzes the first committed step in arachidonic-acid metabolism. Two isoforms of the membrane protein COX are known: COX-I and COX-II. COX-I enzymes are constitutively expressed in most tissues and are responsible for the biosynthesis of cytoprotective prostaglandins in the gastric mucosa and the kidney.[Citation1] COX-II enzymes are induced by cytokines, mitogens, and endotoxins in inflammatory cells[Citation1] and are responsible for the elevated production of prostaglandins in inflammatory cells and the central nervous system.[Citation2,Citation3] The structure of the COX enzyme consists of three distinct domains: an N-terminal epidermal growth factor (EGF) domain, followed by a membrane-binding motif, and the C-terminal catalytic domain which contains the COX and peroxidase active sites[Citation4] Inhibition of both COX-I and COX-II with little specificity, leads to serious side effects, such as gastric lesions and renal toxicity.[Citation5,Citation6] The differential tissue distribution of the COX enzymes was the basis for the development of COX-II selective inhibitors. COX-II selective inhibitors have been identified to have potent anti-inflammatory activity in vivo with minimal gastric side effects.[Citation7] Recognition of new avenues for selective COX-II inhibitors in cancer chemotherapy and neurological diseases such as Parkinson and Alzheimer’s diseases still continues to attract investigations on the development of COX-II inhibitors.[Citation8] Phenolic compounds in plants have attracted increasing attention as potential agents for preventing and treating many inflammation-associated human diseases.[Citation9] Among the enriched phenolics are the Egyptian edible plants Corchorus olitorius L., Malvaceae (Co) and Vitis vinifera L., Vitaceae (Vv) that were investigated for their anti-inflammatory activity. We have previously reported that quercetin and its derivatives are the major components responsible for this anti-inflammatory activity.[Citation10] In this study we evaluate the COX inhibitory activity of these flavonoids using in silico docking studies illustrated by in vitro studies. Molecular docking analyses will help in understanding the binding mode of these derivatives and thus lead to further development of more potent and selective COX-II derivatives.
Materials and methods
In vitro COX-I and COX-II inhibition
The aerial parts of C. olitorius (1.5 kg) and the leaves (with stalks) of V. vinifera (1.5 kg) were collected from the Zoological Garden, Giza, Egypt, Spring, 2012. A voucher specimen (395) is deposited in the herbarium of German University in Cairo (GUC), Egypt. The authenticity of species was confirmed by Professor Dr. Abdel Salam Mohamed Al-Nowiahi, Professor of Taxonomy, Faculty of Science, Ain-Shams University, Egypt. Each plant was individually extracted with double distilled water (8 L). The aqueous extracts were evaporated in vacuo at low temperature until dryness, yielding 160 g of C. olitorius and 190 g of V. vinifera. The dried aqueous extract of both plants were dissolved in ethanol to extract the highest percentage of phenolics followed by evaporation of the filtrate to obtain crude phenolic content of 90 and 110 g from (Co) and (Vv), respectively.
The hydroalcholic extracts of C. olitorius and V. vinifera were evaluated for their COX inhibitory activity in vitro by using human peripheral blood mononuclear cells (PBMC), as they were added to the wells in the presence of 10 µL of heme. Specific wells were dedicated to incubate cell lysate with the tested agent in the presence of either COX-I inhibitor SC-560 or COX-II inhibitor DuP-697(synthesized at Searle). The reactions were initiated by adding 20 µL of arachidonic acid solution. The inhibitory activity of the tested compounds was assessed using Cayman’s COX assay kit (Ann Arbor, MI, USA). The absorbance was measured at 590 nm using a plate reader (ChroMate-4300, FL, USA).
Target Selection
The coordinates of each crystal structure were retrieved from the protein data bank (PDB). The PDB was screened to select crystal structures based upon their resolution and presence of a co-crystallized ligand. In case of COX-II, priority was given for structures co-crystallized with a selective COX-II inhibitor. The two crystal structures chosen for COX-I and COX-II enzymes were 1EQG and 3LN1, respectively. 1EQG is an x-ray crystal structure for COX-I enzyme complexed with ibuprofen with 2.61 Ǻ resolution and 0.223 R-value.[Citation11] 3LN1 is an x-ray crystal structure for COX-II enzyme complexed with a selective inhibitor celecoxib with 2.40 Ǻ resolution and 0.235 R-value.[Citation12] The original PDB files were prepared by adding hydrogens, assigning gastiger charges and assigning bond order. Identical and redundant protein chains together with nonessential cofactors, ions, and water molecules were discarded. The Protein Data Bank files were converted to protein data bank (PDB), partial charge (Q), and atom type (T) files by Molecular Graphics Laboratory Tools (MGLTOOLs) package (version 1.5.6) for AutoDock Vina docking experiments (version 1.1.2).[Citation13]
Preparation of Metabolites
It was found that quercetin glycosides are not present in plasma as they undergo metabolism to yield mainly: quercetin, quercetin-3’sulphate, 3’methyl quercetin, quercetin-7-glucuornide, and quercetin-4’-glucuornide[Citation14] Molecular mechanics force field (MMFF) conformational search was carried out in vacuum for all these studied derivatives using Spartan’06 (version 1.1.1) default settings. Following this conformational search, a HF 6-31G* energy minimization algorithm was conducted on the global minima conformation using Spartan’06 default convergence criterion.
Docking Experiment
All docking experiments were conducted using Vina default settings. The docking grid was centered on the co-crystallized ligand with a size of 20 Å × 20 Å × 20 Å and a grid spacing of 1 Å. By default, the docking was terminated when the maximum energy difference between the best scored pose and the worst one was 3 kcal/mol. We assessed the posed prediction success by docking the co-crystallized ligand into its corresponding binding site of the crystal structure. We calculated root-mean-square deviation (RMSD) between the docked poses and the original co-crystallized ligand using Pymol.
Results and Discussion
In vitro studies
The hydroalcohol extracts of Co and Vv were studied for their inhibitory activity on both COX-I and COX-II enzymes. The inhibitory activities of these extracts were compared to indomethacin, the reference drug in this study. In vitro assay for both extracts at a concentration of 100 µg/mL showed a relatively higher selective inhibition of COX-II over COX-I enzyme. C. olitorius and V. vinifera extracts caused a COX-I enzyme inhibition of 41.75 and 24% compared to a COX-II enzyme inhibition of 62.4 and 49.2%, respectively (). Attempts were made to identify the compounds responsible for this inhibitory effect within each extract. Quercetin 3-O-galactoside and quercetin-3-O-glucuronide were isolated as they represent the major compounds (21.2) and (27.7%) in C. olitorouis and V. vinifera, respectively, using (Waters 600 E multisolvent delivery system, Waters 600 E pump and Waters 2998 PDA) which was employed using a Lichrospher_ 100 RP-18 (250 10mm i.d.;10 lm; Merck KGaA, Darmstadt, Germany), the mobile phase used: MeOH–H2O, 2:8 at 4mL/min for purification the major compounds. The COX inhibitory activities of these compounds were studied at a concentration of 25 µg/mL. Quercetin 3-O-galactoside showed inhibition of COX-I and COX-II enzymes of 39.2 and 60.7%, respectively. Similarly, quercetin-3-O-glucuronide showed inhibition of COX-I and COX-II enzymes of 37.6 and 60%, respectively. The inhibitory activity of the hydroalcohol extracts were found similar to that of the major isolated compounds with a relatively higher COX-II inhibitory activity compared to that of COX-I. Our results are in agreement with previous literature where quercetin 3-O-galactoside (hypersoide) was found responsible for COX-I[Citation15] and COX-II inhibition via regulating the protein and messenger ribonucleic acid (mRNA) expression levels of COX-II[Citation16] and inhibiting the production of E2 production. Quercetin-7-O-glucuronide was also reported to inhibit COX-I[Citation17] as well as COX-2 via reduction of COX-II mRNA expression in interleukin 1-β.[Citation13]
TABLE 1 Effect of Quercetin metabolites as major components of C. olitorius and V. vinifera on cyclooxygenase (COX-I) and cyclooxygenase (COX-II) inhibitory activity
Docking Analysis
Bauer et al.[Citation18] have evaluated the docking performance of Glide, Gold, and AutoDock Vina with different datasets in an attempt to find an effcient virtual screening workflow for a specific target. According to their results; for both COX-I and COX-II enzymes; Autodock Vina showed good screening performance.[Citation19] For further validation, docking of ibuprofen and celecoxib was carried out to assess the performance of the docking protocol to reproduce their experimental binding mode. Comparison of the best scoring pose of both ibuprofen and celecoxib to those found in their crystal structures showed no significant structural differences with a calculated RMSD of 0.103 and 0.158 Å, respectively ().
Exploring COX-II Extra Pocket by Quercetin Metabolites
The in silico studies were in agreement to the in vitro results where docking of quercetin derivatives showed a relative selectivity of these derivatives to COX-II active site (). COX-II has an extra binding pocket which is reported to be a pre-requisite for COX-II drug selectivity.[Citation20] It was previously found that three amino acid differences resulted in a larger (about 20%) and more accessible channel, in COX-II. The exchange of a valine at position 523 in COX-II (Val335) for a relatively bulky isoleucine (Ile) residue in COX-I allowed access to an additional hydrophobic pocket. In addition, exchange of Ile-434 for a valine (Val403) in COX-II allows a neighboring residue phenylalanine-518 (Phe-518) to swing out of the way, resulting in further access to the side cavity
FIGURE 2 (Left): Superimposition of the active sites of both COX-I (cyano) and COX-II (magenta) showing the major structural differences resulting in a larger pocket in COX-II enzyme. (Right): COX-II active site consists of 3 main pockets: Pocket A formed of Phe367, Tyr371, Ser516, Trp373, and Leu370. Pocket B formed of Leu345, Arg106, Val102, Met99, Leu517, and Tyr341. Pocket C formed of Tyr341, Ser399, Phe504, Val509, and Leu338.
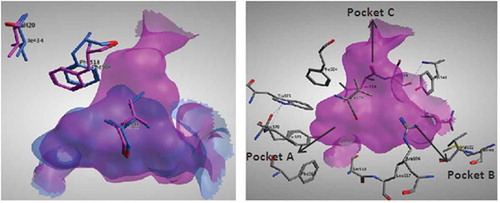
TABLE 2 Calculated docking scores for Quercetin metabolites into the active site of COX-I and COX-II enzymes
Celecoxib’s selective COX-II inhibitory activity is attributed to the presence of a sulfonamide group that fits into the side pocket C of COX-II forming hydrogen bonds with the hydrophilic side chains (His75 and Gln178). Van der Waals interactions dominate the remainder of the celecoxib’s interactions with the protein.[Citation12] The docking poses for quercetin and its derivatives were examined to check their ability to bind to this extra pocket. The two best scoring derivatives, quercetin and methyl quercetin, had their benzopyran ring exploring this extra pocket ( left). Overlay of quercetin on the co-crystallized structure of celecoxib was done to compare their poses. The bezopyran ring found in quercetin showed similar configuration to the 4-benzene sulphonamide moiety found in celecoxib and accounting for its selectivity
FIGURE 3 (Left): Quercetin and its metabolites docked inside COX-II active site. Quercetin (yellow), methyl quercetin (grey), quercetin sulphate (pink), and quercetin-7-glucuornide (green) all were able to bind to COX-II extra binding domain with the exception of quercetin-4-glucuornide (blue) which appears to be out of the pocket. (Right): Overlay of Celecoxib (grey), Quercetin (magenta), and Methyl quercetin (cyano) showing the benzopyran ring superimposed on the benzene sulphonamide moiety.
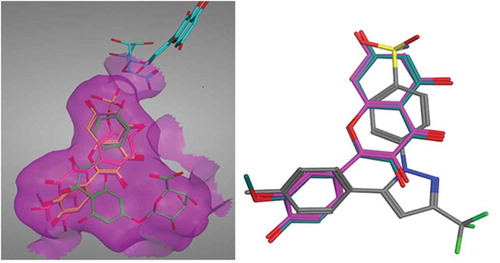
Benzopyrans were first identified as a novel class of COX-II inhibitors in 2003.[Citation23] These compounds showed useful in selective inhibition of COX-II over COX-I. Chateurvidi et al.[Citation24] have reported a pharmacophore model for 2,3 diaryl benzopyran analogues.[Citation24] They reported two aromatic probes, an acceptor probe and a sulfonyl probe as key pharmacophoric features for optimal COX-II inhibitory activity for this class of compounds. Quercetin showed the same reported pharmacophoric features but lacked an aromatic feature due to absence of a 3-aryl substituent on the benzopyran ring.
Although the binding mode of quercetin had never been reported, yet several benzopyran analogues have been crystallized with COX-II aiming at understanding their selectivity. Three X-ray crystal structures have been reported having a benzopyran analogue co-crystallized with COX-II enzyme. The orientation of both compounds SC-75416 and SD8381 found in the two crystal structures 3MQE and 3LN0, respectively, placed the benzopyran ring in pocket A of COX-II binding site. On the other hand, the binding mode of the non-selective compound 23(d) in 3NTG crystal structure was rotated 180º placing the benzopyran ring in pocket B of the active site.[Citation12] None of these three benzopyran analogues was shown to explore the extra pocket C in COX-II active site. This suggests that a slight variation in the position and nature of the substituents present in the structure of these analogues can alter their binding mode and consequently their potency and selectivity. Quercetin differs from the other crystallized analogues in having a catechol ring at the 2-position on the benzopyran ring and absence of bulky substituents at other positions on the ring. Our proposed quercetin binding mode placed its benzopyran ring in the extra pocket C of the COX-II enzyme
(Fig. 4).Conclusion
In the present study, in vitro studies showed quercetin to have potential activity on both COX-I and COX-II with preferential selectivity toward the COX-II active site. Docking studies of quercetin and its derivatives further supported these results showing the docking poses of these derivatives to able to explore the extra pocket found in COX-II active site which is a prerequisite for selective COX-II inhibitors. These results prove valuable in proposing quercetin as a potential selective COX-II inhibitor. Our proposed quercetin binding mode placed the benzopyran ring present in its structure in this extra pocket pointing out that this ring could be responsible for the selectivity of these derivatives. This binding mode explores some interesting findings for future design of more benzopyran derivatives with potential COX-II inhibitory activity.
REFERENCES
- Herschman, H.R. Prostaglandin Synthase 2. Biochimica et Biophysica Acta 1996, 1299, 125–140.
- Seibert, K.; Zhang, Y.; Leahy, K.; Hauser, S.; Masferrer, J.; Perkins, W.; Lee, L.; Isakon, P. Pharmacological and Biochemical Demonstration of the Role Of Cyclooxygenase 2 in Inflammation and Pain. Proceedings of the National Academy of Sciences of the United States of America, 1994, 91, 12013–12017.
- Masferrer, J.L.; Zweifel, B.S.; Manning, P.T.; Hauser, S.D.; Leahy, K.M.; Smith, W.G.; Isakson, P.C.; Seiber, K. Selective Inhibition of Inducible Cyclooxygenase 2 in Vivo Is Anti-inflammatory And Nonulcerogenic. Proceedings of the National Academy of Sciences of the United States of America, 1994, 91, 3228–3232.
- Kurumbail, R.G.; Stevens, A.M.; Gierse, J.K.; McDonald, J.J.; Steqeman, R.A.; Pak, J.Y.; Gildehaus, D.; Miyashiro, J.M.; Penning, T.D.; Seibert, K.; Isakson, P.C.; Stallings, W.C. Structural Basis for Selective Inhibition of Cyclooxygenase-2 by Anti-Inflammatory Agents. Nature 1996, 384, 644–648.
- Laneuville, O.; Breuer, D.K.; Dewitt, D.L.; Hla, T.; Funk, C.D.; Smith, W.L. Differential Inhibition of Human Prostaglandin Endoperoxide H Synthases-1 and -2 By Nonsteroidal Anti-Inflammatory Drugs. Journal of Pharmacology and Experimental Therapeutics 1994, 271, 927–934.
- Mitchell, J.A.; Akarasereenont, P.; Thiemermann, C.; Flower, R.J.; Vane, J.R. Selectivity of Nonsteroidal Antiinflammatory Drugs As Inhibitors of Constitutive and Inducible Cyclooxygenase. Proceedings of the National Academy of Sciences of the United States of America 1993, 90, 11693–11697.
- Lin, C.; Li, C.; Shih, Y.; Chuang, L. Antioxidant and Anti-Inflammatory Properties of Lower-Polymerized Polyphenols in Oolong Tea. International Journal of Food Properties 2014, 16, 752–764.
- Asanuma, M.; Miyazaki, I. Nonsteroidal Anti-Inflammatory Drugs in Experimental Parkinsonian Models and Parkinson’s Disease. Current Pharmaceutical Design 2008, 14, 1428–1434.
- Katalinic, V.; Mozina, S.; Generalic, I.; Skroza, D.; Ljubenkov, I.; Klancnik, A. Phenolic Profile, Antioxidant Capacity, and Antimicrobial Activity of Leaf Extracts from Six Vitis Vinifera L. Varieties. International Journal of Food Properties 2013, 16, 45–60.
- Handoussa, H.; Hanafi, R.; Eddiasty, I.; El-Gendy, M.; El Khatib, A.; Linscheid, M.; Mahran, M.; Ayoub, N. Anti-Inflammatory and Cytotoxic Activities of Dietary Phenolics Isolated from Corchorus Olitorius and Vitis Vinifera. Journal of Functional Foods 2013, 5, 1204–1216.
- Selinsky, B.S.; Gupta, K.; Sharkey, C.T.; Loll, P.J. Structural Analysis of NSAID Binding by Prostaglandin H2 Synthase: Time-Dependent and Time-Independent Inhibitors Elicit Identical Enzyme Conformations. Biochemistry 2001, 40, 5172–5180.
- Wang, J.L.; Limburg, D.; Graneto, M.J.; Springer, J.; Hamoer, J.R.; Liao, S.; Pawlitz, J.L.; Kurumbail, R.G.; Maziasz, T.; Talley, J.J.; Kiefer, J.R.; Carter, J. The Novel Benzopyran Class of Selective Cyclooxygenase-2 Inhibitors. Part 2: The Second Clinical Candidate Having a Shorter and Favorable Human Half-Life. Bioorganic & Medicinal Chemistry Letters 2010, 20, 7159–7163.
- Trott, O.; Olson, A.J. AutoDock Vina: Improving the Speed and Accuracy of Docking with a New Scoring Function, Efficient Optimization, and Multithreading. Journal of Computational Chemistry 2010, 31, 455–461.
- O’Leary, K.A.; Day, A.J.; Needs, P.W.; Mellon, F.A.; O’Brien, N.M.; Williamson, G. Metabolism of Quercetin-7- and Quercetin-3-Glucuronides by An in Vitro Hepatic Model: The Role of Human Beta-Glucuronidase, Sulfotransferase, Catechol-O-Methyltransferase, and Multi-Resistant Protein 2 (MRP2) in Flavonoid Metabolism. Biochemical Pharmacology 2003, 65, 479–491.
- Hillwig, M.L.; Hammer, K.D.; Birt, D.F.; Wurtele, E.S. Characterizing the Metabolic Fingerprint and Anti-Inflammatory Activity of Hypericum Gentianoides. Journal of Agricultural and Food Chemistry 2008, 56, 4359–4366.
- Choi, J.H.; Kim, D.W.; Yun, N.; Choi, J.S.; Islam, M.N.; Kim, Y.S.; Lee, S.M. Protective Effects of Hyperoside Against Carbon Tetrachloride-Induced Liver Damage in Mice. Journal of Natural Products 2011, 74, 1055–1060.
- Hiermann, A.; Schramm, H.W.; Laufer, S. Anti-Inflammatory Activity of Myricetin-3-O-Beta-D-Glucuronide and Related Compounds. Inflammation Research 1998, 47, 421–427.
- Boeckler, F.; Ibrahim, T.; Vogel, S.; Bauer, M. Evaluation and Optimization of Virtual Screening Workflows with DEKOIS 2.0—A Public Library of Challenging Docking Benchmark Sets. Journal of Chemical Information and Modeling 2013, 53, 1447–1462.
- Boeckler, F.M.; Ibrahim, T.M.; Vogel, S.M.; Bauer, M.R. Evaluation and Optimization of Virtual Screening Workflows with DEKOIS 2.0—A Public Library of Challenging Docking Benchmark Sets 2013, 53, 1447–1462.
- Zarghi, A.; Arfaei, S. Selective COX-2 Inhibitors: A Review of Their Structure-Activity Relationships. Iranian Journal of Pharmaceutical Research 2011, 10, 655–683.
- Dannhardt, G.; Kiefer, W. Cyclooxygenase Inhibitors—Current Status and Future Prospects. European Journal of Medicinal Chemistry 2001, 36, 109–126.
- Charlier, C.; Michaux, C. Dual Inhibition of Cyclooxygenase-2 (COX-2) and 5-Lipoxygenase (5-LOX) As a New Strategy to Provide Safer Non-Steroidal Anti-Inflammatory Drugs. European Journal of Medicinal Chemistry 2003, 38, 645–659.
- Joo, Y.; Kim, J.; Kang, S.; Noh, M.; Ha, J.; Choi, J.; Lim, K.; Chung, S. 2,3-Diarylpyranon-4-Ones: A New Series of Selective Cycloxygenase-2-Inhibitors. Bioorganic & Medicinal Chemistry Letters 2004, 14, 2195–2198.
- Chaturvedi, S.; Manivannan, M.; Prasanna, S. Quantitative Structure—Activity Relationship Analysis of a Series of 2,3-Diaryl Benzopyran Analogues As Novel Selective Cyclooxygenase-2 Inhibitors. Bioorganic & Medicinal Chemistry Letters 2004, 14, 4005–4011.