Abstract
Bovine casein peptide had a strong angiotensin-I-converting enzyme inhibitory activity. The objective of this study was to evaluate the effects of the galactose concentration on angiotensin-I-converting enzyme inhibitory activity, antioxidant activity, and physicochemical properties of Maillard reaction products derived from bovine casein peptide and galactose in aqueous model system. The browning intensities of galactose-bovine casein peptide Maillard reaction products increased remarkably with the galactose concentration increased during heat treatment, while its pH and amino groups decreased significantly. The fluorescence compounds of galactose-bovine casein peptide Maillard reaction products reached the maximum when the galactose concentration was 45 g/L. Compounds with molecular weight between 2941 and 8864 Da were dominant while compounds smaller than 250 Da were also produced during the reactions, as characterized by size exclusion chromatography. Galactose-bovine casein peptide Maillard reaction products lost 14.6% of the original angiotensin-I-converting enzyme inhibitory activity of bovine casein peptide after the addition of galactose concentration ranged from 0 to 12%, but its strong antioxidant activities were obtained. A high galactose concentration could decrease angiotensin-I-converting enzyme inhibitory activity of bovine casein peptide and enhance its antioxidant activities.
Introduction
The Maillard reaction is classified as non-enzymatic browning reaction through condensation of carbonyl groups of reducing sugar and amino groups from amino acids, peptides, or proteins.[Citation1] Maillard reaction products (MRPs) are produced during processing and storage of various foods and are largely responsible for desirable food attributes, such as aromas, tastes, and colors. MRPs also lead to the loss of nutritional values and production of potentially toxic compounds. Maillard reaction is strongly influenced by some factors, such as type and concentration of reactants, water activity, temperature, heating time, or pH values.[Citation2,Citation3] Among these parameters, the selection of concentration of reducing sugars is crucial, due to the fact that different concentration of reducing sugars follow various pathways of the Maillard reaction. Regulation of these parameters can control the Maillard reaction progress and physicochemical characteristics of the resulting MRPs.
Recently, several studies are available discussed on MRPs from peptide–sugar model systems and most of these works were focused on the kinetics, functional properties, sensory properties, and antioxidant activities. For example, Pentoses over hexose reacted with a shrimp hydrolysate during Maillard reaction.[Citation4] The effect of temperature on peptide degradation and peptide crosslinking occurred during the Maillard reaction.[Citation5] MRPs that were prepared with rice protein hydrolysates and dextran T20 at 100°C for 20 min, showed the greatest improvement in solubility, emulsification activity, and emulsification stability.[Citation6] Functional properties of soy protein hydrolysate were also enhanced by conjugation with curdlan.[Citation7] Ogasawara et al.,[Citation8] studied flavor-enhancing properties of the MRPs from 1000 to 5000 Da peptides. Xylose, cysteine, and sunflower protein hydrolysate model system could potentially be used to produce flavor enhancers with high antioxidant activity.[Citation9] The peanut peptides with smaller molecular weight showed a higher reaction degree to increase antioxidant activity and to produce more volatile compounds through Maillard reaction with glucose.[Citation10] The effects of Maillard reaction conditions on antiradical activity of tuna stomach hydrolysate–sugar MRPs were reported.[Citation11] However, little work has been conducted on the angiotensin-I-converting enzyme (ACE) inhibitory activity of MRPs derived from peptide and sugar.
Many ACE inhibitory peptides has been isolated in enzymatic hydrolysate from food proteins[Citation12] and especially from milk proteins,[Citation13] which are widely applied as functional ingredients in many processed foods. Our previous research[Citation14] has validated that bovine casein peptides (BCPs) had strong ACE inhibitory activity. Nevertheless, BCPs are regarded as important precursors of the Maillard reaction in food production, processing, storage, and distribution. It is not evident whether BCPs would keep biological activity in the presence of different initial concentrations of reducing sugars during the Maillard reaction. Therefore, the objective of this study was to evaluate effects of initial galactose concentration on ACE inhibitory activity, antioxidant activity, molecular weight distribution, fluorescence formation, browning intensity, free amino group, and pH of BCPs and their possible MRPs.
Materials and methods
Materials
Bovine casein was prepared from bovine milk using isoelectric precipitation by the Key Laboratory of Dairy Science (Northeast Agricultural University), Ministry of Education (Harbin, Heilongjiang Province, China). AS1.398 neutral protease (from Bacillus subtilis; activity 50,000 U/g) was purchased from Wuxi Enzyme Preparation Company (Wuxi, China). D-galactose, o-phthaldialdehyde (OPA), L-leucine, Potassium ferricyanide(III) powder, 2,2-Diphenyl-1-picryl-hydrazyl (DPPH), Hippuryl-Lhisstidyl-L-leucine (Hip-His-Leu), ACE (EC 3.4.15.1), hippuric acid (HA), bovine serum albumin, β-lactoglobulin, ribonuclease, bacitracin, MSH tetrapeptide, Leu-Try-Met-Arg, Bradykimin, Tyr-Glu, Leu-Phe, Asp-Glu, and trifluoroacetic acid (TFA) were purchased from Sigma-Aldrich Co. (St. Louis, MO, USA). Sodium hydroxide (NaOH), sodium chloride (NaCl), Hydrochloride (HCl), sodium borate, and sodium phosphate were of analytical grade.
Preparation of BCPs
Bovine casein was dissolved in pH 7.0, 0.04 mol/L phosphate buffer to give a starting protein concentration of 75 g/L. The enzyme to substrate ratio was 1:20 (w/w protein). During hydrolysis, temperature was controlled at 45°C in a stirred water bath and pH value was maintained at 7.0 by continuous addition of a 1 mol/L NaOH. After 6 h of hydrolysis reaction, enzyme inactivation was carried out at 95°C for 20 min. Casein hydrolysate was centrifuged in a LG10-2.4 refrigerated centrifuge (Beijing Medical Instrument Co., Ltd., Beijing, China) at 4000 × g for 20 min at 4°C. The supernatant was ultrafiltrated through a 10 kDa molecular weight cut-off membrane (Millipore Co., Billerica, MA) at 25°C under a pressure of 20 bar. The obtained permeates were collected and lyophilized to obtain BCPs.
Preparation of MRPs
BCPs and galactose were dissolved in Milli-Q water. In this mixture, 30 g/L of BCPs reacted with 15, 30, 45, 60, 90, and 120 g/L galactose at 95°C with the initial pH value of 9.0 in sealed screw-top glass tubes. After the 3 h of heat treatment, all mixture solutions were cooled immediately in ice water. Control experiments with only casein peptides (30 g/L) were heated without galactose were also conducted. The pH was measured at 25°C using a calibrated pH meter (Mettler Toledo Co., Ltd., Shanghai, China).
Determination of Browning and Absorbance at 294 nm
The advanced stage of the Maillard reaction was monitored by the increase in absorbance at 420 nm.[Citation15] Asorbance at 294 nm was used to determine the precursor of brown pigment formation in the Maillard reaction and caramelization reactions.[Citation16] Browning intensities and ultraviolet (UV)-absorbance of galactose-BCP MRPs were analyzed by absorbance readings at 420 and 294 nm, using a UV-Visible spectrophotometer (UV-2401PC, Shimadzu Co., Ltd., Japan). Samples were diluted to 10-fold and 100-fold with Milli-Q water for browning intensities and UV-absorbance analysis, respectively.
Fluorescence Spectroscopy
Fluorescence spectra of galactose-BCP MRPs were determined using a F-4500 Fluorescence Spectrometer (Hitachi. Co. Ltd., Japan). Samples were diluted to 100-fold with Milli-Q water. The spectrum of excitation and emission was scanned from 300 to 400 nm and 370 to 600 nm, corresponding to the emission and excitation wavelength set at 425 and 347 nm, respectively. Both of excitation and emission slits were set to 10 nm. The scan rate was 120 nm/min. Fluorescence intensity of samples was determined at excitation wavelength of 347 nm and emission wavelength of 425 nm.
Molecular Weight Measurement of MRPs
The molecular weight distribution profiles of galactose-BCP MRPs were estimated by size exclusion chromatography using a series connection column with TSKgel G 2000 SW (600 × 7.5 mm I.D., 10 μm particle size, Tosoh Corporation, Tokyo, Japan) fitted to a TSK guard column (75 × 7.5mm I.D.). The samples of 20 μL aliquot were injected at the concentration of 2.5 g/L. Thirty percent acetonitrile (ACN) in 0.1% TFA Milli-Q water was used as the eluent at a flow rate of 1.0 mL/min. Absorbances were monitored at 214 nm. The 10 standards used were bovine serum albumin (67,000 Da), β-lactoglobulin (36,000 Da), ribonuclease (13,700 Da), bacitraci (1423 Da), MSH tetrapeptide (764.84 Da), Leu-Try-Met-Arg (604.77 Da), bradykimin (368.44 Da), Tyr-Glu (310.31 Da), Leu-Phe (278.35 Da), and Asp-Glu (262.22 Da) as molecular weight (MW) calibration standards (log MW = –0.1346x + 7.9722, with retention time, expressed in minutes, R2 = 0.9852).
Determination of Free Amino Group Content
Free amino groups were quantified by the OPA method.[Citation17] Briefly, the 30 μL sample was diluted to 8-fold and mixed with 1 mL of OPA reagent. After vortexing and a minimal 5 min delay in the dark at 20°C, the absorbance was recorded at 340 nm against the OPA reagent. The blank was determined in the same manner, except that Milli-Q water was used instead of the sample. Absorbance readings were converted into free amino contents using a calibration curve obtained with L-leucine (0 to 0.8 g/L). The change in free amino group was expressed as relative concentrations (%) in comparison with the content of heated samples without galactose.
Determination of ACE Inhibitory Activity
ACE inhibitory activity analysis of galactose-BCP MRPs was measured by high-performance liquid chromatography (HPLC), with a modification of the method of Cushman and Cheung.[Citation18] Hip-His-Leu (5.0 mmol/L) was dissolved in 50 mmol/L sodium borate buffer (pH 8.3) containing 0.5 mol/L NaCl. A mixture containing 120 μL hippuryl-L-histidyl-L-leucine (HHL) solution and 20 μL sample was preincubated at 37°C for 5 min and 10 μL of ACE solution (100 Units/L) was then added. And the mixture was incubated at 37°C for 60 min. The reaction was stopped by the addition of 150 μL of 1 mol/L HCl. HA liberated by ACE was determined by reverse phase–high-performance liquid chromatography (RP–HPLC) onto a Yilite SinoChrom ODS-BP C18 column (4.6 mm i.d. × 250 mm, 5 μm, Dalian, China) at 30°C. Samples were filtered through 0.45 μm filters and 50 μL were injected. Samples were eluted using a stepwise gradient of ACN and Milli-Q water containing 0.1% TFA as follows: 0–12 min, 15–75% ACN and 13–15 min, 75% ACN. The detection wavelength was set at 228 nm and the flow rate was held at 1 mL/min. The percentage of ACE inhibitory activity was calculated as follows: ACE inhibitory activity (%) = (A0 – A1) /A0 × 100%, where A0 represents the HA without sample and A1 represents the HA with sample.
Determination of Ferrous Reducing Power
The ferrous reducing power of galactose-BCP MRPs was determined according to the modified method of Chawla et al.[Citation19] Briefly, 0.1 mL of MRPs sample was diluted 12-fold and mixed with 0.5 mL of 0.2 mol/L sodium phosphate buffer (pH 6.6) and 0.5 mL of 1% potassium ferricyanide. The reaction mixtures were incubated in a water bath at 50°C for 20 min, followed by addition of 0.5 mL of 10% trichloroacetic acid. The mixtures were then centrifuged at 5000 × g using an Eppendorf 5417R centrifuge (Eppendorf AG, Hamburg, Germany) for 10 min, and 0.5 mL of supernatant was blended with 0.5 mL of Milli-Q water and 0.1 mL of 0.1% FeCl3. The reaction mixture was left for 10 min and its absorbance was measured at 700 nm. The results were expressed as absorbance units. Higher absorbance of the reaction mixture indicated greater reducing power.
Determination of DPPH Radical Scavenging Activity
DPPH radical scavenging activity of galactose-BCP MRPs was estimated according to a modified method of Yen and Hsieh.[Citation20] The sample (250 μL) was added to 1 mL of a solution of DPPH, freshly prepared daily, at a concentration of 0.1 mmol/L in ethanol. The reaction solution was then shaken vigorously and allowed to stand in the dark at room temperature for 30 min. The mixture was centrifuged at 4000 × g for 10 min. The absorbance of the supernatant of mixtures was recorded at 517 nm. The percentage of DPPH radical scavenging activity was calculated as follows:
where Asample is the absorbance of samples, Acontrol is the absorbance of ethanol added instead of 0.1 mM DPPH and Ablank is the absorbance of ethanol added instead of samples.
Statistical Analysis
Three independent trails were carried out with preparation of galactose-BCP MRP under the condition of different initial concentrations of galactoses in the Maillard reaction. Analyses including the determinations of browning, UV-absorbance, fluorescence spectra, pH, free amino groups, ACE inhibitory activity, ferrous reducing power, and DPPH radical scavenging activity were run in triplicate. Size exclusion chromatography (SEC) measurements were carried out in duplicate. One-way analysis of variance (ANOVA) was conducted to determine the significance of the main effects by using the SPSS system (SPSS 13.0 for windows, SPSS Inc., Chicago, IL). Experimental results were expressed as means with standard deviation. Significant differences (p < 0.05) among means were identified using the Duncan’s multiple range tests.
Results and discussion
Changes in Browning Intensity and Absorbance at 294 nm
Effects of galactose concentration on browning intensity and absorbance at 294 nm of galactose-BCP MRPs were presented in , during heat treatment at pH 9.0 and 95°C for up to 3 h. A sharp incensement in A420 and A294 of galactose-BCP MRPs was observed at galactose concentration ranging from 0 to 120 g/L (p < 0.05). A correlation between galactose concentration and browning or UV-absorbance of galactose-BCP MRPs was observed (R2 = 0.8603 and 0.9170, respectively). The browning intensity and UV-absorbance of galactose-BCP MRPs were increased by 47-fold and 13-fold, respectively, when galactose concentration rose from 0 to 120 g/L. These results indicated that absorbance at 294 nm and browning intensity were influenced by galactose concentration involved in the Maillard reaction. Galactose-BCP MRPs with high galactose concentration had the stronger browning intensity and absorbance at 294 nm. Apart from Maillard reaction, caramelization of galactose probably might also occur, leading to browning of the galactose-BCP mixtures. This result was in agreement with Benjakul et al.,[Citation21] who found that MRPs derived from 2% sugar had a greater absorbance at 294 nm than did those with 1% sugar. Also, Matmaroh et al.,[Citation22] also reported that the intermediate products and browning intensity increased with increasing reactant concentration.
FIGURE 1 A: Effects of galactose concentration on browning intensity and UV-absorbance at 294 nm of galactose-BCP MRPs during heat treatment at pH 9.0 and 95°C for up to 3 h; B: Effects of galactose concentration on free amino group and pH of galactose-BCP MRPs during heat treatment at pH 9.0 and 95°C for up to 3 h. Error bars represent the standard deviation of the mean of triplicate experiments. Values with different letters are significantly different (p < 0.05).
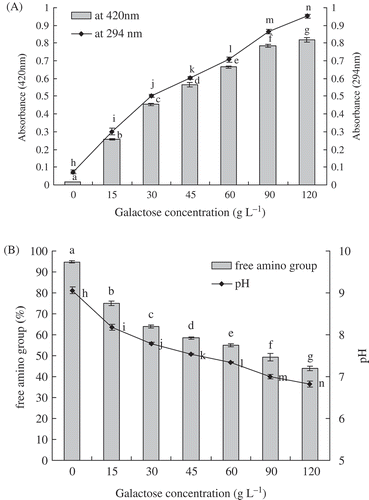
Changes in Free Amino Group and pH
At an early stage of the Maillard reaction, terminal α-amino groups of peptides or proteins and ε-amino groups of lysine residues react with the carbonyl groups of reducing sugars.[Citation23,Citation24] The loss of free amino groups in the galactose-BCP system was determined by the OPA method. Effects of galactose concentration on free amino groups of galactose-BCP MRPs were shown in during heat treatment at pH 9.0 and 95°C for up to 3 h. When the galactose concentration increased, free amino groups of galactose-BCP MRPs significantly decreased (p < 0.05). Free amino groups (5.31%) of was lost after BCP was heated at 95°C for 3 h. However, free amino groups of galactose-BCP MRPs decreased from 94.69 to 43.94%, when galactose concentration ranged from 0 to 12% (p < 0.05). This indicated that initial galactose concentration was generally effective for promoting the Maillard reaction. The initial galactose concentration played an important role on the ultimate content of free amino groups, which determined the formation rate of galactose-BCP MRPs. This observation was in accordance with Ajandouz et al.,[Citation25] and Lertittikul et al.,[Citation26] who reported that disappearance of free amino groups also occurred in protein-reducing sugar model system during the Maillard reaction. Furthermore, a significant decrease in free amino group content, 32.5 and 28.3%, respectively, was observed in the α-LA-ribose and β-LG-ribose MRPs after heat treatment at 95°C for 1 h.[Citation27] Therefore, the loss of available amino groups can be used as an indicator to evaluate the reactivity of Maillard reaction between protein, peptide, or amino acid and sugar.
During the Maillard reaction, the formation of organic acids, such as formic and acetic acid, led to the pH reduction in the MRPs.[Citation28] The consumptions of the N-terminal amino group would notably decrease the pH of galactose-BCP MRPs. Changes in pH of galactose-BCP MRPs as a function of galactose concentration were shown in . According to the results of , there were significant differences of pH among various concentrations of galactose-BCP MRPs systems (p < 0.05). The pH of galactose-BCP MRPs decreased markedly when the galactose concentration ranged from 0 to 12% (p < 0.05). It was also observed that the decreasing rates of pH were dependent on initial sugar concentration of galactose-glycine model systems at 60°C.[Citation29] The pH of MRPs derived from aqueous glucose-diglycine and glucose-triglycine model systems, decreased greatly within the first 10 min and thereafter decreased slightly until it reached 240 min of heating time.[Citation30] However, there is no regularity among the final pH values of silver carp protein hydrolysate-glucose MRPs made of different ratios.[Citation31] These results might be due to the different systems and the different preparations of Maillard reaction.
Effect of Galactose Concentration on Fluorescence Spectrum and Intensity
Fluorogens may be precursors of brown pigments.[Citation32] Fluorescent spectra analysis is used for characterization of the MRPs.[Citation33,Citation34] Effects of galactose concentration on fluorescence excitation and emission spectra, fluorescence intensity of galactose-BCP MRPs and BCP are shown in . Fluorescence intensity of galactose-BCP MRPs was the strongest at excitation wavelength of 347 nm and emission wavelength of 425 nm. When the galactose concentration was 45 g/L, the fluorescence intensity of the galactose-BCP MRPs reached a maximum fluorescence and thereafter slowed to a slow decline, shown in and . For instance, fluorescence intensity of MRPs at initial galactose concentration of 45 g/L was about 20 times more than that of BCP. When the initial galactose concentration increased from 45 to 120 g/L, the fluorescence intensity of MRPs decreased from 553.21 to 545.99. A similar fluorescence spectrum pattern was observed for the ribose-casein model, which reached maximum fluorescence rapidly before slowly decreasing.[Citation35] In contrast, the fluorescence intensity was found to increase gradually with increasing heating time for both glucose-casein[Citation35] and -rice protein[Citation36] model MRPs. This showed that different kinds of MRPs had the different fluorescent development patterns. In the present study, these results indicated that the high initial galactose concentration might force the fluorescence compounds of galactose-BCP MRPs to accumulate quickly and reach a maximum before being converted into brown-colored pigments. This also confirmed that the initial galactose concentration influenced the fluorescent development patterns and determined the Maillard reaction rates of the galactose-BCP model system.
FIGURE 2 A: Effects of galactose concentration on fluorescence excitation (left) and fluorescence emission of galactose-BCP MRPs during heat treatment at pH 9.0 and 95°C for up to 3 h. Excitation wavelength for emission spectra was set to 347 nm and scanning wavelength range from 370 to 600 nm. Emission wavelength for excitation spectra was 425 nm and scanning wavelength range from 300 to 400 nm; B: Effects of galactose concentration on fluorescence intensity of galactose-BCP MRPs (excitation at 347 nm and emission at 425 nm).
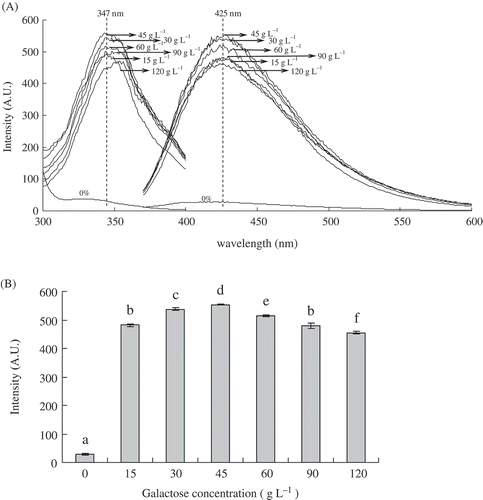
Size Exclusion Chromatography
Galactose-BCP MRPs, formed under a different initial galactose concentration of the Maillard reaction, were monitored at 214 nm by size exclusion chromatography, shown in . Changes in absorbance at 214 nm for BCP and galactose-BCP MRPs presented a similar shape pattern. However, with the initial galactose concentration increased, the chromatographic profiles of galactose-BCP MRPs showed increasing areas under the curve, which reflected the neoformation of a wide range of MRPs. Decourcelle et al.,[Citation37] reported that the area under the curve for shrimp hydrolysate–xylose model system was 1.37 fold greater than for shrimp hydrolysate at 220 nm. Gu et al.,[Citation38] also verified that casein-glucose MRPs comprised high, low, and medium molecular weight compounds.
FIGURE 3. Effects of galactose concentration on size exclusion chromatograms of galactose-BCP MRPs during heat treatment at pH 9.0 and 95°C for up to 3 h.
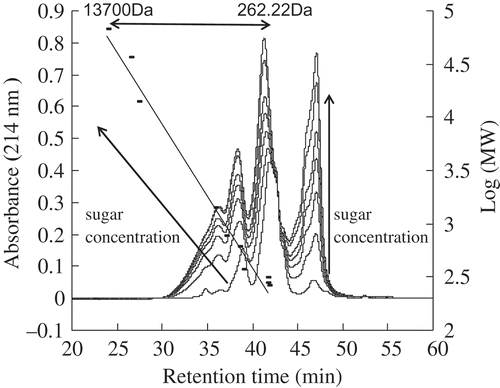
New compounds detected at 214 nm can also be produced in the progress of the Maillard reaction with increasing initial galactose concentration, due to molecular rearrangements in the peptide bonds. In galactose-BCP MRPs of difference galactose concentrations, crosslinking and polymerization occurred for large fractions between 2941 and 8864 Da estimated by molecular weight calibration. BCPs were covalently attached to galactose and galactose degradation products, which resulted in high molecular weight MRPs. Meanwhile, those rearrangements of peptide bonds also affected compounds smaller than 262 Da in all galactose-MRPs. Peptide degradation and caramelization of galactose also induced the formation of low and medium molecular weight compounds. Furthermore, molecular rearrangements and production of new, smaller molecules in galactose-BCP MRPs were as a function of the initial galactose concentrations.
Effect of Galactose Concentration on ACE Inhibitory Activity of Galactose-BCP MRPs
A modified HPLC method was utilized, which based on the liberation of HA from hippuryl-L-histidyl-L-leucine, catalyzed by ACE. Effects of initial galactose concentration on ACE inhibitory activity of galactose-BCP system were analyzed. showed that, in the initial galactose concentration ranging from 0 to 120 g/L, there were significant differences of ACE inhibitory activities in the galactose-BCP system (p < 0.05). With the initial galactose concentration increased, ACE inhibitory activity of galactose-BCP MRPs gradually decreased. For instance, ACE inhibitory activity of MRPs decreased from 77.11 to 62.50%, corresponding to the galactose concentration from 0 to 120 g/L. It was validated that bioactive tripeptide IPP (Ile-Pro-Pro) also lost its known ACE inhibitory activity due to the Maillard reaction with ribose.[Citation39] However, Rufian-Henares et al.[Citation40] demonstrated that food melanoidins from coffee, beer, and sweet wine showed ACE inhibitory activity. The fructose–amino acid model MRPs, except fructose–leucine, fructose–proline, fructose–alanine, and fructose–valine MRPs, showed higher activities than the glucose–amino acid model MRPs.[Citation41] In this study, BCPs showed strong ACE inhibitory activities. Initial galactose concentration could substantially decrease ACE inhibitory activities of BCPs glycated with galactose by Maillard reaction. This indicated that consumption of amino groups of BCP mainly led to its loss of ACE inhibitory activity in the high galactose concentration. Furthermore, the loss of the ACE inhibitory activity was a positive correlation with the consumption of amino groups in the galactose-BCP system.
FIGURE 4. A: Effects of galactose concentration on ACE inhibitory activity of galactose-BCP MRPs during heat treatment at pH 9.0 and 95°C for up to 3 h; B: Effects of galactose concentration on DPPH radical scavenging activity and ferrous reducing power of galactose-BCP MRPs during heat treatment at pH 9.0 and 95°C for up to 3 h. Error bars represent the standard deviation of the mean of triplicate experiments. Values with different letters are significantly different (p < 0.05).
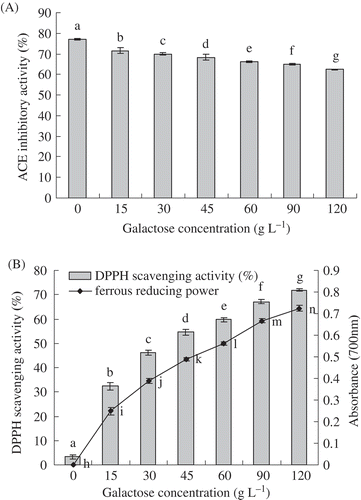
Effect of Galactose Concentration on Antioxidant Activities of Galactose-BCP MRPs
DPPH radicals is scavenged by antioxidants through the donation of hydrogen to form a stable DPPH-H molecule.[Citation42] Stable radical DPPH has been widely used for the determination of antioxidant activity of various food materials.[Citation43] In this study, the DPPH radical-scavenging activity of galactose-BCP MRPs increased significantly as the galactose concentration increased (p < 0.05), shown in . In contrast to this, the heated BCP lacked the DPPH scavenging activity of its corresponding MRPs. Furthermore, the DPPH scavenging activity of MRP from 120 g/L galactose was 22-fold more than that BCP. slightly greater activity was also found with porcine plasma protein–sugar MRP derived from 2% sugar than that from 1% sugar within the 5 h of the heating time.[Citation21] The DPPH radical scavenging activity was increased with increasing xylose concentration and reached the plateau at 30 mg/mL xylose concentration in the xylose-porcine haemoglobin hydrolysate model system.[Citation44]
Besides radical-scavenging activity, reducing power is also an important indictor of antioxidant activity. Hydroxyl groups and pyrrole groups of MRPs played an important role in the reducing activity.[Citation45,Citation46] Zeng et al.[Citation47] showed that the reducing power of the MRPs from psicose was also stronger than that from fructose. Ferrous reducing power of IPP-ribose MRPs increased significantly with the heating time.[Citation39] In this test, ferrous reducing power of galactose-BCP MRPs increased significantly with the galactose concentration increased (p < 0.05), shown in the . Heated BCP did not have ferrous reducing power of its corresponding MRPs. Ferrous reducing power of MRP from 120 g/L galactose was the biggest among all the galactose-BCP MRPs. The previously mentioned results indicated that the high initial sugar concentration of the Maillard reaction could produce MPRs with strong DPPH radical scavenging activity and ferrous reducing power. DPPH radical scavenging activity and ferrous reducing power of galactose-BCP MRPs were also positively related with their browning intensity.
Conclusions
Galactose-BCP MRPs were prepared at the various galactose concentrations. During the Maillard reaction, the browning rate of galactose-BCP MRPs increased significantly with an increased galactose concentration, while the fluorescence intensity of galactose-BCP MRPs reached a maximum value at the initial galactose concentration of 45 g/L, and thereafter gradually decreased. Free amino groups and pH of galactose-BCP MRPs decreased remarkably with increased galactose concentration. Size exclusion chromatography of galactose-BCP MRPs indicated large molecular rearrangements and production of new smaller molecules as initial galactose concentration increased. In comparison with BCP, high galactose concentration led to a considerable loss of ACE inhibitory activity of galactose-BCP MRPs as well as an increase in ferrous reducing power and radical-scavenging activity. Therefore, ACE inhibitory activity, antioxidant activity, free amino group, pH, browning intensity, fluorescence intensity, and the molecular weight distribution of BCPs were greatly affected by the initial galactose concentration involved in the Maillard reaction.
Acknowledgments
The authors would like to thank Teagasc Food Research Centre at Moorepark for the analysis of the size-exclusion chromatography.
FUNDING
This study was supported by the project for the National “Twelfth Five-Year” Plan for Science and Technology Support Program of China (No. 2013BAD18B06), the Open Research Fund for Key Laboratory of Dairy Science (Northeast Agricultural University), the Ministry of Education, Harbin China (201201), and the Innovative Research Team of Higher Education of Heilongjiang Province (2010td11).
Additional information
Funding
References
- Baltes, W. Chemical Changes in Food by the Maillard Reaction. Food Chemistry 1982, 9, 59–73.
- Ashoor, S.H.; Zent, J.B. Maillard Browning of Common Amino-Acids and Sugars. Journal of Food Science 1984, 49, 1206–1207.
- Stamp, J.A.; Labuza, T.P. Kinetics of the Maillard Reaction Between Aspartame and Glucose in Solution at High-Temperatures. Journal of Food Science 1983, 48, 543–544.
- Laroque, D.; Inisan, C.; Berger, C.; Vouland, É.; Dufossé, L.; Guérard, F. Kinetic Study on the Maillard Reaction. Consideration of Sugar Reactivity. Food Chemistry 2008, 111, 1032–1042.
- Lan, X.; Liu, P.; Xia, S.; Jia, C.; Mukunzi, D.; Zhang, X.; Xia, W.; Tian, H.; Xiao, Z. Temperature Effect on the Non-Volatile Compounds of Maillard Reaction Products Derived from Xylose-Soybean Peptide System: Further Insights into Thermal Degradation and Cross-Linking. Food Chemistry 2010, 120, 967–972.
- Li, Y.; Zhong, F.; Ji, W.; Yokoyama, W.; Shoemaker, C.F.; Zhu, S.; Xia, W. Functional Properties of Maillard Reaction Products of Rice Protein Hydrolysates with Mono-, Oligo-, and Polysaccharides. Food Hydrocolloids 2013, 30, 53–60.
- Fan, J.F.; Zhang, Y.Y.; Szesze, T.; Li, F.J.; Zhou, M.Y.; Saito, M.; Tatsumi, E.; Li, L.T. Improving Functional Properties of Soy Protein Hydrolysate by Conjugation with Curdlan. Journal of Food Science 2006, 71, C285–C291.
- Ogasawara, M.; Katsumata, T.; Egi, M. Taste Properties of Maillard-Reaction Products Prepared from 1000 to 5000 Da Peptide. Food Chemistry 2006, 99, 600–604.
- Eric, K.; Raymond, L.V.; Huang, M.; Cheserek, M.J.; Hayat, K.; Savio, N.D.; Amédée, M.; Zhang, X. Sensory Attributes and Antioxidant Capacity of Maillard Reaction Products Derived from Xylose, Cysteine, and Sunflower Protein Hydrolysate Model System. Food Research International 2013, 54, 1437–1447.
- Su, G.; Zheng, L.; Cui, C.; Yang, B.; Ren, J.; Zhao, M. Characterization of Antioxidant Activity and Volatile Compounds of Maillard Reaction Products Derived from Different Peptide Fractions of Peanut Hydrolysate. Food Research International 2011, 44, 3250–3258.
- Sumaya-Martinez, M.T.; Thomas, S.; Linard, B.; Binet, A.; Guerard, F. Effect of Maillard Reaction Conditions on Browning and Antiradical Activity of Sugar–Tuna Stomach Hydrolysate Model System. Food Research International 2005, 38, 1045–1050.
- Gu, X.; Hou, Y.K.; Li, D.; Wang, J.Z.; Wang, F.J. Separation, Purification, and Identification of Angiotensin I-Converting Enzyme Inhibitory Peptides from Walnut (Juglans Regia L.) Hydrolyzate. International Journal of Food Properties 2015, 18, 266–276.
- Phelan, M.; Aherne, A.; FitzGerald, R.J.; O’Brien, N.M. Casein-Derived Bioactive Peptides: Biological Effects, Industrial Uses, Safety Aspects, and Regulatory Status. International Dairy Journal 2009, 19, 643–654.
- Jiang, Z.; Tian, B.; Brodkorb, A.; Huo, G. Production, Analysis, and In Vivo Evaluation of Novel Angiotensin-I-Converting Enzyme Inhibitory Peptides from Bovine Casein. Food Chemistry 2010, 123, 779–786.
- Morales, F.J.; Jiménez-Pérez, S. Free Radical Scavenging Capacity of Maillard Reaction Products As Related to Colour and Fluorescence. Food Chemistry 2001, 72, 119–125.
- Ajandouz, E.H.; Tchiakpe, L.S.; Ore, F.D.; Benajiba, A.; Puigserver, A. Effects of pH on Caramelization and Maillard Reaction Kinetics in Fructose-Lysine Model Systems. Journal of Food Science 2001, 66, 926–931.
- Church, F.C.; Swaisgood, H.E.; Porter, D.H.; Catignani, G.L. Spectrophotometric Assay Using O-Phthaldialdehyde for Determination of Proteolysis in Milk and Isolated Milk Proteins. Journal of Dairy Science 1983, 66, 1219–1227.
- Cushman, D.W.; Cheung, H.S. Spectrophotometric Assay and Properties of the Angiotensin-Converting Enzyme of Rabbit Lung. Biochemical Pharmacology 1971, 20, 1637–1648.
- Chawla, S.P.; Chander, R.; Sharma, A. Antioxidant Properties of Maillard Reaction Products Obtained by Gamma-Irradiation of Whey Proteins. Food Chemistry 2009, 116, 122–128.
- Yen, G.C.; Hsieh, P.P. Antioxidative Activity and Scavenging Effects on Active Oxygen of Xylose-Lysine Maillard Reaction-Products. Journal of the Science of Food and Agriculture 1995, 67, 415–420.
- Benjakul, S.; Lertittikul, W.; Bauer, F. Antioxidant Activity of Maillard Reaction Products from a Porcine Plasma Protein-Sugar Model System. Food Chemistry 2005, 93, 189–196.
- Matmaroh, K.; Benjakul, S.; Tanaka, M. Effect of Reactant Concentrations on the Maillard Reaction in a Fructose-Glycine Model System and the Inhibition of Black Tiger Shrimp Polyphenoloxidase. Food Chemistry 2006, 98, 1–8.
- Ledl, F.; Beck, J.; Sengl, M.; Osiander, H.; Estendorfer, S.; Severin, T.; Huber, B. Chemical Pathways of the Maillard Reaction. Progress in Clinical and Biological Research 1989, 304, 23–42.
- Van Boekel, M.A.J.S. Effect of Heating on Maillard Reactions in Milk. Food Chemistry 1998, 62, 403–414.
- Ajandouz, E.H.; Desseaux, V.; Tazi, S.; Puigserver, A. Effects of Temperature and pH on the Kinetics of Caramelisation, Protein Cross-Linking, and Maillard Reactions in Aqueous Model Systems. Food Chemistry 2008, 107, 1244–1252.
- Lertittikul, W.; Benjakul, S.; Tanaka, M. Characteristics and Antioxidative Activity of Maillard Reaction Products from a Porcine Plasma Protein–Glucose Model System As Influenced by pH. Food Chemistry 2007, 100, 669–677.
- Jiang, Z.; Brodkorb, A. Structure and Antioxidant Activity of Maillard Reaction Products from α-Lactalbumin and β-Lactoglobulin with Ribose in An Aqueous Model System. Food Chemistry 2012, 133, 960–968.
- Brands, C.M.J.; van Boekel, M. Kinetic Modeling of Reactions in Heated Monosaccharide-Casein Systems. Journal of Agricultural and Food Chemistry 2002, 50, 6725–6739.
- Liu, S.C.; Yang, D.J.; Jin, S.Y.; Hsu, C.H.; Chen, S.L. Kinetics of Color Development, pH Decreasing, and Anti-Oxidative Activity Reduction of Maillard Reaction in Galactose/Glycine Model Systems. Food Chemistry 2008, 108, 533–541.
- Kim, J.S.; Lee, Y.S. Study of Maillard Reaction Products Derived from Aqueous Model Systems with Different Peptide Chain Lengths. Food Chemistry 2009, 116, 846–853.
- You, J.; Luo, Y.; Shen, H.; Song, Y. Effect of Substrate Ratios and Temperatures on Development of Maillard Reaction and Antioxidant Activity of Silver Carp (Hypophthalmichthys Molitrix) Protein Hydrolysate-Glucose System. International Journal of Food Science and Technology 2011, 46, 2467–2474.
- Patton, A.R. Paper Chromatography of Browning Reaction Fluorogens. Nature 1951, 167, 406.
- Morales, F. J.; van Boekel, M. A Study on Advanced Maillard Reaction in Heated Casein/Sugar Solutions: Fluorescence Accumulation. International Dairy Journal 1997, 7, 675–683.
- Matiacevich, S.B.; Santagapita, P.R.; Buera, M.P. Fluorescence from the Maillard Reaction and Its Potential Applications in Food Science. Critical Reviews in Food Science and Nutrition 2005, 45, 483–495.
- Jing, H.; Kitts, D.D. Chemical Characterization of Different Sugar-Casein Maillard Reaction Products and Protective Effects on Chemical-Induced Cytotoxicity of Caco-2 Cells. Food and Chemical Toxicology 2004, 42, 1833–1844.
- Li, Y.; Lu, F.; Luo, C.; Chen, Z.; Mao, J.; Shoemaker, C.; Zhong, F. Functional Properties of the Maillard Reaction Products of Rice Protein with Sugar. Food Chemistry 2009, 117, 69–74.
- Decourcelle, N.; Sabourin, C.; Dauer, G.; Guérard, F. Effect of the Maillard Reaction with Xylose on the Emulsifying Properties of a Shrimp Hydrolysate (Pandalus Borealis). Food Research International 2010, 43, 2155–2160.
- Gu, F.; Kim, J.M.; Hayat, K.; Xia, S.; Feng, B.; Zhang, X. Characteristics and Antioxidant Activity of Ultrafiltrated Maillard Reaction Products from a Casein–Glucose Model System. Food Chemistry 2009, 117, 48–54.
- Jiang, Z.; Rai, D.K.; O’Connor, P.M.; Brodkorb, A. Heat-Induced Maillard Reaction of the Tripeptide IPP and Ribose: Structural Characterization and Implication on Bioactivity. Food Research International 2013, 50, 266–274.
- Rufian-Henares, J.A.; Morales, F.J. Angiotensin-I Converting Enzyme Inhibitory Activity of Coffee Melanoidins. Journal of Agricultural and Food Chemistry 2007, 55, 1480–1485.
- Hwang, I.G.; Kim, H.Y.; Woo, K.S.; Lee, J.; Jeong, H.S. Biological Activities of Maillard Reaction Products (MRPS) in a Sugar-Amino Acid Model System. Food Chemistry 2011, 126, 221–227.
- Matthaus, B. Antioxidant Activity of Extracts Obtained from Residues of Different Oilseeds. Journal of Agricultural and Food Chemistry 2002, 50, 3444–3452.
- Zhang, Y.W.; Liu, J.; Lu, X.; Zhang, H.; Wang, L.; Guo, X.N.; Qi, X.G.; Qian, H.F. Isolation and Identification of An Antioxidant Peptide Prepared from Fermented Peanut Meal Using Bacillus Subtilis Fermentation. International Journal of Food Properties 2014, 17, 1237–1253.
- Sun, Q.; Luo, Y. Effect of Maillard Reaction Conditions on Radical Scavenging Activity of Porcine Haemoglobin Hydrolysate-Sugar Model System. International Journal of Food Science and Technology 2011, 46, 358–364.
- Yanagimoto, K.; Lee, K.-G.; Ochi, H.; Shibamoto, T. Antioxidative Activity of Heterocyclic Compounds Formed in Maillard Reaction Products. International Congress Series 2002, 1245, 335–340.
- Yoshimura, Y.; Iijima, T.; Watanabe, T.; Nakazawa, H. Antioxidative Effect of Maillard Reaction Products Using Glucose-Glycine Model System. Journal of Agricultural and Food Chemistry 1997, 45, 4106–4109.
- Zeng, Y.; Zhang, X.; Guan, Y.; Sun, Y. Characteristics and Antioxidant Activity of Maillard Reaction Products from Psicose-Lysine and Fructose-Lysine Model Systems. Journal of Food Science 2011, 76, C398–C403.