Abstract
The objective of this study was to identify and quantify the volatile flavor composition of selected Saskatchewan grown pulses including navy beans, red kidney beans, green lentils, and yellow peas, and to determine the flavor changes induced by thermal processing. Flavor profile of roasted flours, ground roasted seeds, pre-cooked seeds, pre-cooked slurries, pre-cooked–freeze-dried, and pre-cooked–spray-dried flours was studied using headspace solid-phase microextraction gas chromatography/mass spectrometry. The highest total area count (p < 0.05) was found in navy bean and the lowest in red kidney bean. 3-Hexanol was the most abundant volatile flavor compound. Pre-cooking significantly reduced (p < 0.05) volatile compounds total area count by 61.75%, except for the red kidney bean and yellow pea, whereas roasting significantly increased (p < 0.05) total area count for navy bean and red kidney bean. Major differences observed in relative peak area for the same chemical family showed that volatile flavor compounds of pulses were significantly affected by type and processing conditions. Basic knowledge of the volatile profiles of pulses and the flavor changes occurred following different types of thermal processing, could ensure better quality control of raw materials and help product developers meet flavor-delivery challenges. The relevant information may also be of interest to relevant industries targeting specific pulse-based food product development.
Introduction
Pulses are a good and inexpensive source of proteins, complex carbohydrates, fiber, and minerals and are gaining increasing recognition around the world as healthy foods that can provide an adequate supply of energy and protein to meet various dietary needs.[Citation1] The growing trend toward convenience and ready-to-eat foods has stimulated interest in processing pulse seeds to obtain flours and different fractions. Opportunities exist for using pulses and their fractions as supplements in the development of various food products, such as weaning food, meat products, bakery products, soups, purees, extruded snacks, pasta, yogurt, and salad dressing. However, the presence of anti-nutritional compounds (such as trypsin inhibitors) and some undesirable beany flavors are challenges that need to be addressed in food product development. Significant reductions in anti-nutritional activities, as affected by various thermal treatments, have been reported in a number of studies.[Citation2–Citation7] Thus, the potential of thermally treated pulse ingredients with enhanced nutritional properties for use in different food products could be increasingly realized. The flavor profile of these value-added ingredients, however, remains an issue of interest as flavor is considered an important quality trait in determining the acceptability of food products.
The fresh flavors of legumes are associated with naturally occurring compounds, normal metabolism of the plants and the flavors produced by enzymatic degradation during harvesting, storage, and processing. For example, volatile C6 and C9 aldehydes and alcohols are the principal chemicals produced upon physical disruption of the tissues of edible plants; this occurs as a result of aerobic oxidation of linoleic and linolenic acids in the presence of lipoxygenase and alcohol oxidoreductase[Citation8] or by autoxidative decomposition of these fatty acids. The flavor associated with cooked legumes (e.g., green, beany, and earthy odor) is due to enzymatic, non-enzymatic, and chemical reactions induced during thermal processing depending on the temperature applied. Chemical compounds identified so far in pulses, include aldehydes, alcohols, ketones, and heterocyclic compounds.[Citation9–Citation13] These play an important role in determining the flavor profiles of pulses and may be significantly altered during cooking. The appearance of novel chemical compounds and the changes in their concentrations during thermal processing could also have a significant impact on the taste and flavor of the finished food product.
Headspace solid-phase microextraction (HS-SPME) has been used in combination with gas chromatography (GC) and mass spectrometry (MS) for flavor analysis of different food matrices (e.g., vegetables, fruits, juices, soft drinks, and alcoholic beverages).[Citation14] HS-SPME combines sampling, extraction, concentration, and sample introduction in a single solvent-free step through adsorption/absorption of volatile flavors onto an adsorbent fiber coated with an appropriate stationary phase.[Citation15] Absorption/adsorption is based on equilibrium partitioning of the analytes between the solid phase of the SPME fiber and the liquid or solid sample matrix.[Citation16] The approach has proven efficient and effective as a method for the sensitive detection of volatile, semi-volatile, polar, and non-polar compounds. In the coupled system, the SPME fiber with the concentrated analytes is transferred to and desorbed into a hot GC injector port and eluted by the mobile phase for subsequent chromatographic analysis and MS detection.[Citation9,Citation15,Citation17–Citation20]
In our previous studies, the flavor profile of Saskatchewan grown field peas (Pisum sativum L.) as affected by cultivar, crop year, processing (i.e., dry milling, cooking, and dehulling), location, as well as storage conditions was examined.[Citation9,Citation21,Citation22] The perceived aroma and flavor properties of cooked field peas with different varieties were also evaluated by trained sensory panel.[Citation23] Until now, very few published studies have focused on the volatile components of different pulse varieties, and changes in flavor profiles associated with different types of thermal processing have not been investigated in a systematic manner. The aim of the present study, therefore, was to identify and compare the flavor profiles of selected Saskatchewan grown pulses (i.e., green lentils, yellow peas, red kidney beans, and navy beans) and to study the effects of thermal processing, such as cooking and drying (i.e., roasted flour, roasted seeds, pre-cooked seeds, pre-cooked slurry, pre-cooked–freeze-dried, and pre-cooked–spray-dried samples), on changes in the volatile flavor compounds of these pulses.
Materials and methods
Materials
The following pure commercial standards were purchased from Sigma-Aldrich (Oakville, ON, Canada): alcohols (1-butanol, 1-pentanol, 3-pentanol, 3-hexanol, 1-penten-3-ol, 2-methoxy-4-vinylphenol, 1-nonanol, 2-ethyl-2-hexanol, 2-methoxy-ethanol, 2-ethyl-1-butanol), aldehydes (benzaldehyde, hexanal, octanal, 3-methyl-butanal, 2-methyl-butanal, dodecanal, heptanal), ketones (acetone, 2-pentanone, 2-heptanone, 2-butanone), aromatic compounds (toluene, ben zothiazole, 2-methyl furan, stryrene, p-xylene, o-xylene), alkanes (decane, heptane, nonadecane, n-undecane, n-tetradecane, trichloromethane), terpenes (d-limonene, 3-carene, camphene), ester (ethyl acetate), sulphur compounds (dimethylsulfide), and nitrogen compounds (2-methyl-pyrazine, ethyl pyrazine, 1-pentanamine, 2-ethyl-3-methyl pyrazine, 2,6-demethylpyrazine, 2,5-dimethylpyrazine, 2,3-diethyl-5(6)-methylpyrazine). All standards used are chromatographic grade reagents.
Green lentil (Lens culinaris, Laird variety) and yellow field pea (Pisum sativum L., Eclipse variety) seeds were provided by pulse growers in Saskatchewan (Saskatoon, SK, Canada); common bean (Phaseolus vulgaris L., including dark red kidney and navy bean varieties) seeds were provided by the Crop Development Centre (Saskatoon, SK, Canada). These cultivars were grown in three replicates under uniform conditions using recommended agronomic practices on land managed by the Crop Development Centre, University of Saskatchewan, Canada near Saskatoon, Saskatchewan. The seeds collected were from the crop year of 2011. Dry seeds were stored at 4°C until analysis.
Preparation of Volatile Standards
Standard solutions were prepared as described by Azarnia et al.[Citation9] Briefly, individual stock solutions of each commercial standard listed in the previous section were prepared in 15-mL screw-top amber vials (Supelco, Oakville, ON, Canada) as follows: 10 mL of each commercial standard was individually diluted in 10 mL of methanol (solution 1). Subsequently 1 mL of solution 1 was diluted in 9 mL of methanol (solution 2). Ten microliters of solution 2 was then diluted in 1 mL of 6M saturated NaCl solution. The prepared standard was then analyzed by HS-SPME-GC/MS. Pure 2-pentanone standard was used as a daily reference standard; it was injected at the beginning and after each sixth injection of sample. The reproducibility of the method was evaluated by calculating the relative standard deviation (RSD) of the area count of the standard during each injection. RSD values between 2.4 and 4.0% were ensured.
Sample Preparation
The four different varieties of pulse seeds were processed using seven different thermal treatments as described below. (1) Raw flours were prepared by grinding dry seeds (60 g) in a domestic coffee grinder in a Black & Decker SmartGrind Model CBG100S coffee bean grinder (The Black & Decker Corporation, Towson, MD, USA) for 40 s and then passing them through a 106-µm sieve; the sieved material was collected for further analysis and is referred to as “R.” (2) The sieved material (R) was then spread thinly (25 g) in a sealed aluminium dish and roasted for 1 min in an electric double oven (model OD302, Fisher & Paykel Appliances Ltd., Huntington Beach, CA, USA) at 100°C; the roasted flour sample is referred to as “RF.” (3) Roasted seeds were prepared by roasting the whole seeds (80 g) in a sealed aluminium dish for 20 min in the same oven as above at 100°C, and then ground and sieved using the same method as for raw flours; the roasted seed is referred to as “RS.” (4) Cooked whole seeds were prepared by soaking dry seeds of pea, navy, and red kidney beans in cold deionized water overnight (16 h) at 4°C using a ratio of 1:3 (seeds:water). Soak water was drained before cooking. The lentil seed was cooked directly without prior soaking. Seeds were cooked in boiling double distilled (DD) water using a ratio of 1:3 (seeds:water) for 20, 20, 30, and 40 min for yellow pea, lentil, navy bean, and red kidney bean, respectively. The pre-cooked seeds were weighed and then mashed two to three times inside the SPME vial using a spatula; they are referred to as “PCSE.” (5) The slurry of pre-cooked seeds was prepared by continuously blending PCSE with DD water using a ratio of 1:2 (water:seeds) in a commercial blender (Warring, New Hartford, CT, USA) for 2 min to obtain a homogeneous dispersion. The pre-cooked slurry is referred to as “PCSL.” (6) The freeze-dried sample was prepared by evenly spreading PCSL in a pan, freezing overnight in a freezer at –40°C, and then freeze-dried in a VirTis model 50-SRC-5 freeze dryer (VirTis Co., Inc., Gardiner, NY, USA). The pre-cooked–freeze-dried sample is referred to as “PCFD.” (7) The spray-dried sample was prepared by using a BUCHI Mini Spray Dryer (Model 191, Buchi Laboratoriums-Technik, Flawil, Switzerland), the inlet and outlet temperatures were at 130 and 80°C, respectively. The PCSL was passed through a sieve prior to spray drying. The pre-cooked–spray-dried sample is referred to as “PCSD.” The seeds of each type of pulse were prepared according to the previously mentioned procedures. Two grams of each prepared sample was individually placed into 10-mL headspace amber vials (Supelco, Oakville, ON, Canada) and stored at 4°C prior to analysis by HS-SPME-GC/MS. All the samples were prepared on the day the HS-SPME-GC/MS analysis was performed.
HS-SPME-GC/MS Analysis
Samples were extracted at 50°C for 30 min using a carboxen-polydimethylsiloxane SPME fiber (CAR/PDMS, 85 µm; Supelco, Oakville, ON, Canada) according to the optimized conditions reported by Azarnia et al.Citation9 The fiber was placed into the split/splitless injector (glass insert SPME, 0.8 ID; Varian, Mississauga, ON, Canada) and the volatile compounds were desorbed at 300°C for 3 min. Analytes were eluted using pure helium carrier gas at a constant flow rate of 1 mL min–1 using a VF-5MS capillary column, 30 m × 0.25 mm × 0.25 µm (Varian Inc., Mississauga, ON, Canada). Volatile compounds were determined with a Varian CP-3800 gas chromatograph (Palo Alto, CA). The initial temperature of the GC oven was 35°C; it was held for 3 min and then increased to 80°C at a rate of 6°C min–1, and finally to 280°C at a rate of 20°C min–1, and held for 2 min. The total time of analysis was 22.5 min. A Saturn 2000 MS detector (Varian Inc., Palo Alto, CA, USA) was used for detection of compounds, and the mass range was 40–400 m/z. The total ion current was obtained using an electron ionization source at 70 eV at a scan rate of 1.0 s/scan. Each volatile compound was identified either by the National Institute of Standards and Technology (NIST) database (V.05) through mass spectra library search, or by comparing retention times (RT) and the mass spectra of the compounds with those of the pure commercial standards (as listed in the previous section).
The area count of volatiles for each type of pulse under each processing treatment was obtained from the average of triplicate measurements. The relative peak area (RPA) was calculated using the procedure described by Azarnia et al.[Citation9] as follows:
The TAC for each sample and the sum of RPA values for the same chemical family were calculated and compared for the different type of pulse subjected to the thermal treatments.
Statistical Analysis
Each variety of pulse was grown in three replicates under uniform conditions as described above. These three batches of samples were subjected to different thermal treatments as described previously. Values given in tables and figures are the means of three replicates. Error bars indicate the standard deviation. Statistical significance was evaluated by one-way analysis of variance (ANOVA) using GraphPad PRISM version 3.02 software (GraphPad Software, Inc., San Diego, CA, USA). Significant differences between means were determined by Tukey’s multiple comparison tests at the 5% significance level.
Results and Discussion
Effect of Processing on Total Volatile Compounds
The volatile compounds identified in navy bean, red kidney bean, green lentil, and yellow pea under different thermal treatments are presented in and , grouped by chemical families. Changes in the TAC values of the samples are shown in . Based on the ANOVA test, significant differences (p < 0.05) were observed between different pulse types and between different processing treatments, and interactions between types of pulses and treatments were observed. A comparison of the R samples (), showed that navy bean had the highest TAC (p < 0.05) and red kidney bean the lowest TAC among all the pulse types. TAC values of the volatile compounds from pre-cooked samples (including PCSE, PCSL, PCFD, and PCSD) were generally reduced with an average value of –61.75% compared with the R samples (). The reduction in TAC was significant (p < 0.05) for all the pulse types, except for red kidney bean PCSD and yellow pea PCFD. The observed results are consistent with previously reported findings that showed significantly decreased total volatile compounds counts in cooked peas and pea slurries,[Citation9] as well as in cooked French beans.[Citation10] Whitfield and Shipton[Citation24] also found that the concentrations of volatiles decreased markedly in blanched peas relative to raw pea samples but there was no change in qualitative composition. Together, these results indicate that during cooking and drying, volatile components are lost or reduced. Some workers have also found that the bonds between protein in pulses and the oxygenated products derived from lipid hydroperoxide decomposition are stronger following protein denaturation induced by wet heating, as more active sites in proteins (such as the α-amino group of lysine and the thiol group of cysteine) are available for binding.[Citation25] Flavor compounds may lose their olfactory effect and become less intense as a result of the formation of these lipoprotein complexes, which may also help to explain the general reduction in TAC for pre-cooked samples. For the roasted seeds, the TACs of volatile compounds were significantly increased (p < 0.05) compared with the R samples for the navy bean and red kidney bean ().
TABLE 1 Volatile Compounds Identified in Navy Bean and Red Kidney Beans Using HS-SPME-GC/MS
TABLE 2 Volatile Compounds Identified in Green Lentil and Yellow Pea Using HS-SPME-GC/MS
FIGURE 1 Effect of thermal processing on A: the total area counts (TAC) of volatile compounds; and B: the total relative peak area (RPA) of volatile alcohols present in navy bean, red kidney bean, yellow pea, and green lentil. (R: raw; RF: roasted flour; RS: roasted seed; PCSE: pre-cooked seeds; PCSL: pre-cooked slurry; PCFD: pre-cooked-freeze-dried; PCSD: pre-cooked-spray-dried). Results are the average value of three replications. For each type of pulse, mean values bearing different letters are significantly different (p < 0.05) as per Tukey’s multiple comparison test.
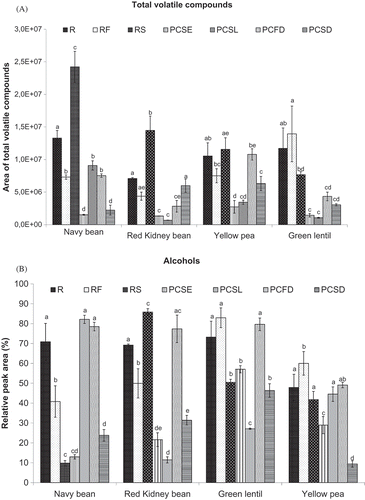
Effect of Processing on Alcohols
The formation of n-hexanol, such as 1-hexanol and 3-hexanol, as presented in and , is typically achieved by the transformation of n-hexanal in the presence of alcohol oxidoreductase.[Citation26] The alcohol oxidoreductase activity, ranging from 0.0031–0.0064 units/mg protein, was previously reported in green beans at pH of 8.8.[Citation27] The formation of 1-penten-3-ol involves a similar pathway: the oxidation of linolenic acid leads to the formation of 16-hydroperoxide; 1-penten-3-one is then formed by enzymatic isomerization of 16-hydroperoxide; and 1-penten-3-one is finally reduced to 1-penten-3-ol in the presence of alcohol oxidoreductase.[Citation27] In our study, 3-hexanol was the most abundant volatile compound, with the highest RPA being found in navy bean, red kidney bean, green lentil, and yellow pea ( and ). This suggests that 3-hexanol, which has a strong green leafy odor,[Citation28] makes an appreciable contribution to the flavor of these pulses; saturated and unsaturated alcohols were quantitatively dominant components and they have been reported to play an important role in pulse flavor.[Citation10–Citation12] The presence of 3-hexanol has been previously reported in green bean, soybean, and winged bean.[Citation2,Citation27,Citation29]
The total RPA values of alcohols for all the R samples were similar (), except that the total RPA of alcohols in the R samples of yellow peas was significantly lower (p < 0.05) than in the other raw pulse samples. The effect that thermal processing had on the total RPA of alcohols can be seen in . A comparison of the raw and pre-cooked pulses (PCSE, PCSL, PCFD, and PCSD) showed a general trend toward a reduction in the concentration of the total RPA of alcohols induced by wet heating. The RPA of alcohols was significantly decreased (p < 0.05) for all PCSE and PCSD samples, as well as for PCSL of red kidney and green lentil. This observation is supported by the finding reported by Del Rosario et al.[Citation2] of a decrease in the concentration of nearly all alcohols in both soybean and winged bean headspace samples upon heating. Roasting of the pulses (i.e., RF and RS) caused a significant increase or decrease (p < 0.05) in the RPA of alcohols relative to the R samples, depending on pulse type. The RF of navy bean and red kidney bean, and the RS of navy bean and green lentil, showed significantly decreased RPA values for alcohols. In contrast, the RS of red kidney bean showed significantly increased RPA values for total alcohol content detected. As reported by Kato et al.[Citation29] differences between the formation rate and the disappearance rate of the various volatile alcohols may explain the differences observed for different types of pulse during the course of roasting.
Effect of Processing on Aldehydes
As shown in and , hexanal was the principal aldehyde found in navy bean, red kidney bean, green lentil, and yellow pea. The presence of hexanal has previously been reported in beans, soybeans, and peas.[Citation2,Citation12,Citation22,Citation30,] In the raw seeds, linoleic acid is oxidized to hydroperoxides in the presence of oxygen; hexanal may be formed by the cleavage of 13-hydroperoxylinoleic acid by lyases.[Citation31] This process often occurs in pulse seeds that are disrupted during processing, and the hexanals formed contribute to the greeny and grassy flavor of legumes. Thermal processing either increased or decreased the RPA of hexanal ( and ).
Other aliphatic saturated aldehydes found in raw pulses ( and ) include 3-methyl butanal (powerful penetrating, acrid, and apple-like odor),[Citation28,Citation32] 2-methyl butanal (pungent, fresh, fruity with a peculiar cocoa and coffee-like flavor),[Citation33,Citation34] heptanal (a very strong, fatty, harsh, pungent odor),[Citation28] nonanal (a strong, fatty odor developing orange, and rose notes),[Citation28] octanal (strong, fruity odor)[Citation32] and dodecanal (a characteristic fatty odor reminiscent of violets on dilution).[Citation28] The amino acid phenylalanine is likely the precursor of most of the aromatic aldehydes of the pulses, including benzaldehyde,[Citation35] as shown in and , which has a characteristic odor and aromatic taste similar to bitter almond.[Citation28] The presence of 2-methyl butanal, 3-methyl butanal, heptanal, and benzaldehyde has been reported in a variety of bean cultivars.[Citation2,Citation10,Citation30]
The RPA of total aldehydes in the different type of pulse is presented in . The R samples of yellow pea had significantly lower RPA values (p < 0.05), whereas higher values were found for red kidney bean and green lentils. The differences observed for R samples may be due to differences in the levels of fatty acids and linoleate.[Citation2,Citation12] In addition, the pre-cooking process (i.e., PCSE, PESL, PCFD, and PCSD) generally led to either statistically equal or significantly increased (p < 0.05) RPA values for total aldehydes in relation to the raw samples. The PCSD samples all exhibited significantly increased (p < 0.05) RPA values for aldehydes compared to R samples. The roasted samples (including RF and RS) generally had statistically similar amounts of total aldehydes, except that a significantly decreased value was observed for the RS of red kidney bean compared with the R samples.
FIGURE 2 Effect of thermal processing on the total relative peak area (RPA) of A: volatile aldehydes; and B: volatile ketones present in navy bean, red kidney bean, yellow pea, and green lentil. (R: raw; RF: roasted flour; RS: roasted seed; PCSE: pre-cooked seeds; PCSL: pre-cooked slurry; PCFD: pre-cooked-freeze-dried; PCSD: pre-cooked-spray-dried). Results are the average value of three replications. For each type of pulse, mean values bearing different letters are significantly different (p < 0.05) as per Tukey’s multiple comparison test.
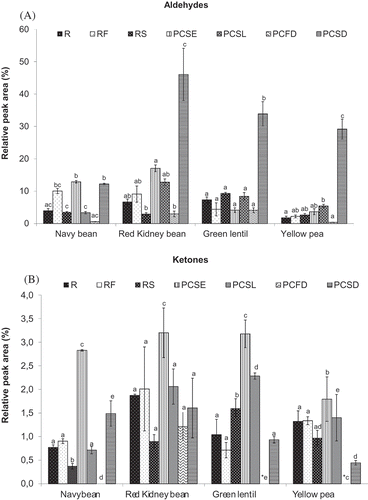
In general, the heat stabilities of lipoxygenase and alcohol oxidoreductase determine the levels of RPA of total aldehydes and alcohols. Lumen et al.[Citation27] reported that the lipoxygenase activity of green bean seeds was quite stable, persisting for up to 40 min during heating at 80°C. In contrast, alcohol oxidoreductase was very unstable; it remained stable for up to 8 min and then was completely inactivated. Thus, the changes observed in the RPA values after thermal processing may be due to differences in the heat stabilities of the two enzymes, which may have led to different impacts on the concentration of alcohols and aldehydes upon heating. Additionally, the formation of these compounds due to non-enzymatic reaction may also be responsible for the changes observed.
Effect of Processing on Ketones
Ketones are carbonyl compounds that are formed by lipoxygenase activity from the breakdown of unsaturated fatty acid hydroperoxides. As shown in and , 2-butanone was found in the R samples of navy bean, red kidney bean, lentils, and peas. 2-Pentanone, which has a mild green, fuel-oil odor, was found in the R samples of yellow pea and RS green lentil. Heating produced small amounts of other ketones in the pulses, including 2-heptanone, which has a fruity, spicy, cinnamon, banana, slightly spicy odor.[Citation28] These compounds have distinctive characteristics that can affect the flavor of pulses and their flavor potency varies with their carbon chain length. The presence of 2-pentanone (moderately sharp, fragrant, mint- or acetone-like odor)[Citation36] and 2-butanone (moderately sharp, sweet, pungent, and acetone-like odor)[Citation37] has been identified in peas, dry beans, and lentils.[Citation9,Citation12]
The PCSE of navy bean, red kidney bean, lentils, and yellow peas all had significantly increased (p < 0.05) total RPA values for ketones compared with the R samples (). The PCSL had either statistically equal (for navy bean and red kidney beans) or significantly increased (for green lentil and yellow peas) total RPA values for ketones. Ketones were not detected in the PCFD samples of navy bean, green lentil, or yellow pea. The PCSD samples exhibited significantly increased (p < 0.05) total RPA values for ketones in navy beans, whereas significantly decreased values were observed for yellow peas relative to the R samples. The RS of navy beans showed significantly decreased RPA values for ketones, whereas the RS of green lentils exhibited significantly increased values ().
Effect of Processing on Aromatic Compounds
2-Methyl-furan was detected in the R samples of navy bean, red kidney bean, green lentils, and yellow peas ( and ). Other substituted furans, e.g., 2-ethyl-furan, which has a powerful, sweet, burnt odor,[Citation28] appeared after heating ( and ). Aromatic compounds (cyclic compounds containing a certain number of double bonds) are present in a wide variety of foods in small quantities. In pulse seeds, they originate from the oxidation of unsaturated fatty acids.[Citation12] Maga and Katz[Citation38] reported the appearance of 2-ethyl furan upon heating, and indicated that a series of alkylated analogues, in particular 2-substituted alkylfurans such as 2-methyl- and 2-ethyl furans, often accompanied the parent furans associated with thermal treatment of complex Maillard reaction precursors or lipids. Azarnia et al.[Citation9] found furan-2-methyl and toluene to be the most abundant aromatic compounds in yellow peas. Other aromatics, including o-xylene and p-xylene, which are lipid-derived compounds, were also detected in this study ( and ); they have also been found in beans, split peas, and lentils.[Citation2,Citation12,Citation39] Additionally, styrene, which has a characteristic sweet, balsamic, almost floral odor, was detected; it has been reported in several field pea cultivars, split pea, beans, and lentils.[Citation9,Citation39]
PCSE and PCSL had generally increased RPA values for total aromatics; the values were significant (p < 0.05), except for the PCSL of navy bean and yellow pea (). In contrast, the PCSD of red kidney bean and yellow pea showed significantly decreased RPA values for aromatics. The RF and RS of navy beans showed significantly increased (p < 0.05) values, whereas the RS of red kidney beans showed significantly decreased RPA values for aromatic compounds (). The varying levels of oxidation of unsaturated fatty acid as a result of the different thermal treatments may explain the differences observed in the formation of aromatic compounds in the pulse samples studied.
FIGURE 3 Effect of thermal processing on the total relative peak area (RPA) of A: volatile aromatic compounds; and B: volatile alkane compounds present in navy bean, red kidney bean, yellow pea, and green lentil. (R: raw; RF: roasted flour; RS: roasted seed; PCSE: pre-cooked seeds; PCSL: pre-cooked slurry; PCFD: pre-cooked-freeze-dried; PCSD: pre-cooked-spray-dried). Results are the average value of three replications. For each type of pulse, mean values bearing different letters are significantly different (p < 0.05) as per Tukey’s multiple comparison test.
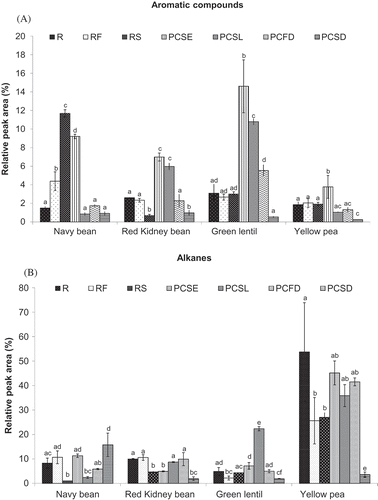
Effect of Processing on Alkane Compounds
The alkane compounds present in pulses originate mainly from the oxidative decomposition of lipids.[Citation40] They generally have weak odours and do not contribute much to flavors in foods; however, they may modify the volatility and flavor-imparting properties of other volatile compounds.[Citation41] Whereas 2-methyl decane was the most abundant alkane in navy bean and red kidney bean, 2,4-dimethyl hexane and 4-methyl decane gave the highest RPA values for alkanes in green lentils and yellow peas ( and ). Chlorinated constituents (i.e., trichloromethane) were found in navy bean, red kidney bean, green lentil, and yellow peas, as shown in and ; they may be produced following minimal exposure of pulse plants to chlorinated organic compound. Their presence can be eliminated by making changes to cultural practices.[Citation39]
The RS and RF of the different pulses generally exhibited significantly decreased or statistically similar RPA values for alkanes in comparison with R samples (). Spray drying (PCSD) significantly decreased (p < 0.05) the RPA of alkanes for red kidney bean, green lentil, and yellow pea, whereas navy bean showed the opposite trend. The PCSE of red kidney bean also exhibited significantly decreased RPA values for alkane compounds ().
Effect of Processing on Terpenoids
Terpenes, including 3-carene, camphene, and D-limonene, were found in raw and thermally treated navy bean, red kidney bean, green lentil, and yellow pea ( and ). The presence of monoterpenes in plants may result from endogenous isoprenoid biosynthesis or from carotenoid degradation by either lipoxygenase (LOX) or hydroperoxides.[Citation11] D-limonene, characterized as having a pleasant, lemon-like odor, is considered the most abundant and widespread terpenes; it appeared in the heated samples of navy bean, red kidney bean, and yellow pea (see and ). Limonene and 3-carene have also been detected in dry and cooked beans, as well as in blanched green peas.[Citation10–Citation12]
The comparison of the volatiles in raw and roasted pulse samples (RS and RF) showed a general increasing trend in the RPA of terpenes for all varieties subjected to roasting (). A significant increase (p < 0.05) in the RPA of terpenes was observed for PCSL of red kidney beans. The PCFD and PCSD of green lentils showed significantly decreased (p < 0.05) RPA for terpenes ().
FIGURE 4 Effect of thermal processing on the total relative peak area (RPA) of A: volatile terpenes present; and B: volatile nitrogen compounds in navy bean, red kidney bean, yellow pea, and green lentil. (R: raw; RF: roasted flour; RS: roasted seed; PCSE: pre-cooked seeds; PCSL: pre-cooked slurry; PCFD: pre-cooked-freeze-dried; PCSD: pre-cooked-spray-dried). Results are the average value of three replications. *Not detected. For each type of pulse, mean values bearing different letters are significantly different (p < 0.05) as per Tukey’s multiple comparison test.
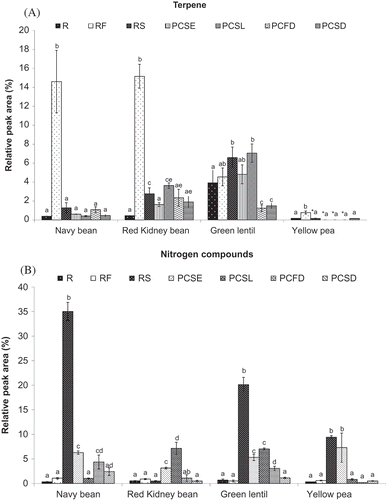
Effect of Processing on Sulphur Compounds
Volatile sulphur compounds occur naturally in foods and can form during heat processing and storage. Sulphur-containing compounds are generally very flavor-active due to their low flavor thresholds and characteristic odors. Dimethyl disulphide, which has a diffuse, intense onion odor, was the predominant sulphur compound found in the pulses ( and ); it is believed to result from the decomposition of methanethiol. The presence of dimethyl disulphide has been reported in raw green peas and in cooked French beans.[Citation9,Citation13] Methanethiol, which has an objectionable odor of decomposing cabbage or garlic, appeared in both the raw and thermally treated samples of navy bean, red kidney bean, green lentil, and yellow pea ( and ). The presence of small quantities of methanethiol was reported in the low boiling volatiles from cooked beans in a study using capillary column GC.[Citation13]
The RPA values for total sulphur compounds were either significantly increased or remained stable after the roasting process (RS and RF), except for the RF of green lentils (figure not shown). The PCSE of the navy bean, red kidney bean and yellow peas showed significantly increased RPA values for total sulphur compounds. The PCFD and PCSD yellow pea, on the other hand, exhibited significantly decreased (p < 0.05) total sulphur compounds compared with R samples.
Effect of Processing on Nitrogen Compounds
As shown in and , most of the nitrogenous flavor compounds (alkylated pyrazines, and pyrrole) were not detected in the raw samples; instead they were generally formed or increased after heating (cooking and roasting) most likely due to the Maillard reaction. Pyrazine compounds are produced by the reaction between amino acids and reducing sugars; they may also be observed upon dry-thermal degradation of proteins.[Citation29] However, in most cases, protein isolates do not produce pyrazines on wet heat treatment (i.e., under high moisture content treatment).[Citation29,Citation42] A diverse group of pyrazines were found at low concentrations in the headspace of the thermally treated samples ( and ). The highest levels of nitrogen compounds were found in the RS samples (). In particular, RS of navy bean, green lentil, and yellow pea exhibited significantly increased (p < 0.05) amounts of nitrogen compounds in comparison with the R samples (). The pre-cooking processes (PCSE, PCSL, PCFD, and PCSD) led to either significantly increased or statistically equal RPA values for nitrogenous compounds. These compounds generally have a chocolate, roasted nut flavor, and a sharp taste.[Citation22] This is consistent with the finding of Kato et al.[Citation29] that alkylated pyrazines were formed or increased markedly during roasting. Koehler et al.[Citation43] reported that, among the alkylated pyrazines, the mono-ethyl-mono-methyl-pyrazines had the lowest odor detection threshold level. The formation of 2-ethyl-5-methyl-pyrazine, 2-ethyl-6-methyl-pyrazine, 2-methyl-6-propyl-pyrazine, and 2-methyl-5-propyl-pyrazine during roasting is of interest as they are desirable food flavors[Citation44,Citation45] that could mask the beany flavor of pulses.
Conclusion
Basic knowledge of the volatile profiles of pulses such as red kidney bean, navy bean, lentils and peas, as well as the flavor changes that occur following different types of thermal processing, could ensure better quality control of raw materials and help product developers meet flavor-delivery challenges during product development. The results presented clearly show changes in the volatile flavor profiles of the treated pulse flours with some flavors disappearing and others appearing on heat treatment. Some of these changes are of interest in product development. For example, the novel volatile compounds such as pyrazines and alkylated pyrazines produced during the roasting and cooking processes may play an important role in masking the beany flavor associated with the presence of aldehydes, alcohols, and sulphur compounds in raw pulses. The significant reduction in the total volatile compounds of the pre-cooked pulses, as well as the changes in volatile contents of different chemical families upon thermal processing, may also be of interest to relevant industries targeting specific pulse-based food product development. Further research using GC/sniffing port analysis, as well as quantitative descriptive analysis (QDA) and hedonic sensory evaluation, will be useful to determine which volatiles are significant contributors to the aroma of different pulse foods.
Acknowledgments
The authors wish to thank Hassan Sabik and Denis Bélanger of the Food Research and Development Centre of Agriculture and Agri-Food Canada in St. Hyacinthe for their technical assistance.
FUNDING
This study was funded by Agriculture and Agri-Food Canada’s Agricultural Bio-product Innovation Program, and National Natural Science Foundation of China (31501405), Natural Science Basic Research Plan in Shaanxi Province of China (2015JQ3081, GK201601002), Fundamental Research Funds for the Central Universities of China (GK201503070), and Scientific Research Foundation for the Returned Overseas Chinese Scholars, State Education Ministry.
Additional information
Funding
REFERENCES
- Boye, J.I.; Ma, Z. Finger on the Pulse. Food Science and Technology (London) 2012, 26, 20–24.
- Del Rosario, R.; De Lumen, B.O.; Habu, T.; Flath, R.A.; Mon, T.R.; Teranishi, R. Comparison of Headspace of Volatiles from Winged Beans and Soybeans. Journal of Agricultural and Food Chemistry 1984, 32,1011–1015.
- Iwuoha, C.I.; Umunnakwe, K.E. Chemical, Physical, and Sensory Characteristics of Soymilk As Affected by Processing Method, Temperature, and Duration of Storage. Food Chemistry 1997, 59, 373–379.
- Jourdan, G.A.; Norena, C.P.Z.; Brandelli, A. Inactivation of Trypsin Inhibitor Activity from Brazilian Varieties of Beans (Phaseolus Vulgaris L.). Food Science and Technology International 2007, 13, 195–198.
- Ma, Z.; Boye, J.I.; Simpson, B.K.; Prasher, S.O. Monpetit D and Malcolmson L, Thermal Processing Effects on the Functional Properties and Microstructure of Lentil, Chickpea, and Pea Flours. Food Research International 2011, 44, 2534–2544.
- Vidal-Valverde, C.; Frias, J.; Estrella, I.; Gorospe, M.; Ruiz, R.; Bacon, J. Effect of Processing on Some Antinutritional Factors of Lentils. Journal of Agricultural and Food Chemistry 1994, 42, 2291–2295.
- Wang, N.; Daun, J.K.; Malcolmson, L.J. Relationship Between Physicochemical and Cooking Properties, and Effects of Cooking on Antinutrients, of Yellow Field Peas (Pisum Sativum). Journal of the Science of Food and Agriculture 2003, 83, 1228–1237.
- Jelen, H.; Wasowicz, E. Lipid-Derived Flavor. In Food Flavors: Chemical, Sensory, and Technological Properties; Jelen, H.; Ed.; CRC Press: Boca Raton, FL, 2011; 65–93.
- Azarnia, S.; Boye, J.I.; Warkentin, T.; Malcolmson, L.; Sabik, H.; Bellido, A.S. Volatile Flavour Profile Changes in Selected Field Pea Cultivars As Affected by Crop Year and Processing. Food Chemistry 2011, 124, 326–335.
- Barra, A.; Baldovini, N.; Loiseau, A.M.; Albino, L.; Lesecq, C.; Lizzani Cuvelier, L. Chemical Analysis of French Beans (Phaseolus Vulgaris L.) by Headspace Solid Phase Microextraction (HS-SPME) and Simultaneous Distillation/Extraction (SDE). Food Chemistry 2007, 101, 1279–1284.
- Jakobsen, H.B.; Hansen, M.; Christensen, M.R.; Brockhoff, P.B.; Olsen, C.E. Aroma Volatiles of Blanched Green Peas (Pisum Sativum L.). Journal of Agricultural and Food Chemistry 1998, 46, 3727–3734.
- Oomah, B.D.; Liang, L.S.Y.; Balasubramanian, P. Volatile Compounds of Dry Beans (Phaseolus Vulgaris L.). Qualitas Plantarum—Plant Foods for Human Nutrition 2007, 62, 177–183.
- Self, R.; Casey, J.C. Swain, T. The Low-Boiling Volatiles of Cooked Foods. Chemistry and Industry 1963, 27, 863–864.
- Kataoka, H.; Lord, H.L.; Pawliszyn, J. Applications of Solid-Phase Microextraction in Food Analysis. Journal of Chromatography A 2000, 880, 35–62.
- Vas, G.; Vékey, K. Solid-Phase Microextraction: A Powerful Sample Preparation Tool Prior to Mass Spectrometric Analysis. Journal of Mass Spectrometry 2004, 39, 233–254.
- Pawliszyn, J. New Directions in Sample Preparation for Analysis of Organic Compounds. TrAC Trends in Analytical Chemistry 1995, 14, 113–122.
- Kataoka, H. Recent Advances in Solid-Phase Microextraction and Related Techniques for Pharmaceutical and Biomedical Analysis. Current Pharmaceutical Analysis 2005, 1, 65–84.
- Xiao, Z.B.; Zhang, N.; Niu, Y.W.; Feng, T.; Tian, H.X.; Zhu, J.C.; Yu, H.Y. Multivariate Classification of Cherry Wines Based on Headspace Solid Phase Microextraction and Gas Chromatography-Mass Spectrometry of Volatile Compounds. International Journal of Food Properties 2015, 18, 1272–1287.
- Ertas, A.; Yilmaz, M.A.; Boga, M.; Hasimi, N.; Yesil, Y.; Goren, A.C.; Temel, H.; Topcu, G. Chemical Profile and Biological Activities of Two Edible Plants: Chemical Investigation and Quantitative Analysis Using Liquid Chromatography Tandem Mass Spectrometry and Gas Chromatography Mass Spectrometry. International Journal of Food Properties 2016, 19, 124–138.
- Lin, M.; Long, M.; Li, G.; Chen, X.; Zheng, J.; Li, C.; Kan, J. Analysis of Peanut Using Near-Infrared Spectroscopy and Gas Chromatography–Mass Spectrometry: Correlation of Chemical Components and Volatile Compounds. International Journal of Food Properties 2015, 19(3), 508–520. DOI:10.1080/10942912.2015.1016575
- Azarnia, S.; Boye, J.I.; Warkentin, T.; Malcolmson, L. Application of Gas Chromatography in the Analysis of Flavour Compounds in Field Peas. In Gas Chromatography in Plant Science, Wine Technology, Toxicology, and Some Specific Applications; Salih, B.; Çelikbıçak, Ö.; Ed.; Intech Publisher: Rijeka, Croatia, 2012; 15–30.
- Azarnia, S.; Boye, J.I.; Warkentin, T.; Malcolmson, L. Changes in Volatile Flavour Compounds in Field Pea Cultivars As Affected by Storage Conditions. International Journal of Food Science and Technology 2011, 46, 2408–2419.
- Malcolmson, L.; Frohlich, P.; Boux, G.; Bellido, A.S.; Boye, J.; Warkentin, T.D. Aroma and Flavour Properties of Saskatchewan Grown Field Peas (Pisum Sativum L.). Canadian Journal of Plant Science 2014, 94, 1419–1426.
- Whitfield, F.B.; Shipton, J. Volatile Carbonyls in Stored Unblanched Frozen Peas. Journal of Food Science 1966, 31, 328–331.
- Beyeler, M.; Solms, J. Interaction of Flavor Model Compounds with Soy Protein and Bovine Serum Albumin. Lebensmittel-Wissenschaft Technologie-Food Science and Technology 1974, 7, 217–219.
- Matoba, T.; Sakurai, A.; Taninoki, N.; Saitoh, T.; Kariya, F.; Kuwahata, M.; Yukawa, N.; Fujino, S.; Hasegawa, K. n-Hexanol Formation from n-Hexanal by Enzyme Action in Soybean Extracts. Journal of Food Science 1989, 54, 1607–1610.
- De Lumen, B.O.; Stone, E.J.; Kazeniac, S.J.; Forsythe, R.H. Formation of Volatile Flavor Compounds in Green Beans from Linoleic and Linolenic Acids. Journal of Food Science 1978, 43, 698–702.
- Burdock, G.A. Fenaroli’s Handbook of Flavour Ingredients, 6th ed., CRC Press: Boca Raton, FL, 2002.
- Kato, H.; Doi, Y.; Tsugita, T.; Kosai, K.; Kamiya, T.; Kurata, T. Changes in Volatile Flavour Components of Soybeans During Roasting. Food Chemistry 1981, 7, 87–94.
- Van Ruth, S.M.; Roozen, J.P.; Cozijnsen, J.L. Volatile Compounds of Rehydrated French Beans, Bell Peppers, and Leeks. Part 1. Flavour Release in the Mouth and in Three Mouth Model Systems. Food Chemistry 1995, 53, 15–22.
- Galliard, T.; Phillips, D.R.; Reynolds, J. The Formation of Cis-3-Nonenal, Trans-2-Nonenal, and Hexanal from Linoleic Acid Hydroperoxide Isomers by a Hydroperoxide Cleavage Enzyme System in Cucumber (Cucumis Sativus) Fruits. Biochimica et Biophysica Acta (BBA)-Lipids and Lipid Metabolism 1976, 441, 181–192.
- Lewis, R.J. Sr. Hawley’s Condensed Chemical Dictionary, 15th Ed.; John Wiley & Sons, Inc.: New York, NY, 2007.
- Boelens, M.H.; Boelens, H.; van Gemert, L.J. Sensory Properties of Optical Isomers. Perfumer & Flavorist, 1993, 18, 1–15.
- Bartschat, D.; Mosandl, A. Stereoisomeric Flavour Compounds LXXX: Alkyl-Branched Alkanals-Stereodifferentiation, Structure Elucidation, and Structure-Function Relationship. European Food Research and Technology 1998, 206, 165–168.
- Murray, K.E.; Shipton, J.; Whitfield, F.B.; Last, J.H. The Volatiles of Off-Flavoured Unblanched Green Peas (Pisum Sativum). Journal of the Science of Food and Agriculture 1976, 27, 1093–1107.
- NIOSH. NIOSH Pocket Guide to Chemical Hazards & Other Databases CD-ROM. Department of Health & Human Services, Centers for Disease Prevention & Control. National Institute for Occupational Safety & Health. DHHS (NIOSH) Publication No. 2005-151, 2005.
- O’Neil, M.J. The Merck Index—An Encyclopedia of Chemicals, Drugs, and Biologicals, 13th Ed.; Merck and Co., Inc.: Whitehouse Station, NJ, 2001; 1084–1085.
- Maga, J.A.; Katz, I. Furans in Foods. Critical Reviews in Food Science and Nutrition 1979, 11, 355–400.
- Lovegren, N.V.; Fisher, G.S.; Legendre, M.G.; Schuller, W.H. Volatile Constituents of Dried Legumes. Journal of Agricultural and Food Chemistry 1979, 27, 851–853.
- Perkins, E.G. Gas Chromatography and Gas Chromatography–Mass Spectrometry of Odor/Flavour Components in Lipid Foods. In Flavour Chemistry of Lipid Foods; Min, D.B.; Smouse, T.H.; Eds.; American Oil Chemists’ Society: Champaign, IL, 1988; 35–36.
- Stevenson, R.J.; Chen, X.D. A Study of Volatile “Trapping” in Spray Dried Whey Protein Concentrate by “Crushing” and/or Vacuuming, and Detection by Solid-Phase Microextraction/Gas Chromatography/Mass Spectrometry. Food Research International 1996, 29, 495–504.
- Qvist, I.H.; Von Sydow, E.C.F. Unconventional Proteins As Aroma Precursors. Chemical Analysis of the Volatile Compounds in Heated Soy, Casein, and Fish Protein Model Systems. Journal of Agricultural and Food Chemistry 1974, 22, 1077–1084.
- Koehler, P.E.; Mason, M.E.; Odell, G.V. Odor Threshold Levels of Pyrazine Compounds and Assessment of Their Role in the Flavor of Roasted Foods. Journal of Food Science 1971, 36, 816–818.
- Buttery, R.G.; Seifert, R.M.; Guadagni, D.G.; Ling, L.C. Characterization of Volatile Pyrazine and Pyridine Components of Potato Chips. Journal of Agricultural and Food Chemistry 1971, 19, 969–971.
- Wang, P.S.; Kato, H.; Fujimaki, M. Studies on Flavour Components of Roasted Barley. Part III. The Major Volatile Basic Compounds. Agricultural and Biological Chemistry 1969, 33, 1775–1781.