Abstract
The objective of this study was to evaluate the effect of Bunium persicum and Rosmarinus officinalis essential oils on the oxidation of virgin olive oil during accelerated storage. The synergistic effect between citric acid and antioxidants of natural and synthetic types was investigated. The B. persicum and R. officinalis essential oils significantly retarded the oxidation of virgin olive oil. Their antioxidant capacity was comparable to butylatedhydroxytoluene and better than β-carotene. Here we show that when natural and synthetic antioxidants are combined with citric acid, they produce an extra antioxidant effect, hence the manifestation of synergy. Generally, the essential oils of B. persicum and R. officinalis can be applied to increase the oxidative stability of virgin olive oil.
INTRODUCTION
Lipid oxidation is one of the major factors that cause deterioration during the storage and processing of edible fats, oils, and fat-containing products. It modifies major quality control parameters of fats and oils such as color, flavor, aroma, and nutritive value, affecting suitability for consumption.[Citation1] Virgin olive oils (VOOs) are known to be more resistant to oxidation than other edible oils because they contain more natural antioxidants and lower levels of unsaturated fats. However, even though VOO generally has a high resistance to oxidation, some of its minor compounds such as free fatty acids and photosensitizers prove to be pro-oxidants by nature. Therefore, different VOOs do not exhibit similar stabilities.[Citation2]
Synthetic antioxidants such as butylatedhydroxyanisole (BHA) and butylatedhydroxytoluene (BHT) are used extensively so as to prevent oxidation. However, restrictions on the use of these compounds are commonplace because of their carcinogenicity.[Citation3] Consequently, researchers need to identify alternative natural and safe sources of antioxidants. The search for natural antioxidants, especially those of plant origin, has notably increased in recent years.[Citation4]
Bunium persicum is an important aromatic plant that belongs to the Apiaceae family. Its origin is traced in regions that range from Central Asia to North India.[Citation5] Antioxidant activity (AA) of γ-terpinene has been reported in the literature as the major component being present in the essential oil (EO) of this aromatic plant. In particular, the activity of γ-terpinene is comparable with that of α-tocopherol. Other main components found in this EO are cuminaldehyde, p-cymene, and limonene.[Citation4,Citation6] Rosmarinus officinalis is an aromatic, medicinal, and condiment plant that belongs to the Labiatae family. R. officinalis is widely accepted as a spice, and is renowned for its flamboyant AA. The yield of R. officinalis EO obtained by steam distillation is reported as 1.2%.[Citation7] The main components of R. officinalis EO are 1,8-cineolel, α-pinene, camphor, and α-terpineol.[Citation8]
Recently, several studies have focused on the oxidative stability of vegetable oils enriched with herbs and spices. For instance, a report claims that B. persicum EO is able to reduce the oxidation rate of the soybean oil.[Citation9] Other reports provide evidence that extracts of rosemary and blackcurrant seeds are highly efficient in delaying primary and secondary oxidative changes.[Citation10] Furthermore, considerable evidence has been gathered on the synergistic effects between the various compounds in food products. Assimopoulou et al.[Citation11] reported that combining the resin of Pistacia lentiscus with citric acid (CA) can lead to synergistic effects in sunflower and corn oil. The objective of this study was to investigate the effect of B. persicum and R. officinalis EOs on the oxidation of VOO. The antioxidant activities of EOs were compared with those of β-carotene and BHT. Here, we hypothesize and test whether the addition of CA to the VOO can offer synergy to the natural and synthetic antioxidants. CA is known as a chelating agent.
MATERIALS AND METHODS
Materials
The dried seeds of B. persicum were purchased from a local market in Shiraz, Iran. Aerial parts of R. officinalis were collected from Fars province in March 2014, which were then dried on a large screened tray under ambient temperature (30–40°C) for 3 days. The genus and species of both plants were certified by experts from the Herbarium of Biology Department at Shiraz University, Shiraz, Iran. The VOO was purchased from the Etka Oil Company (Rudbar, Iran). All experimental chemicals were of analytical grade and were purchased from Merck (Darmstad, Germany) and Sigma–Aldrich (St. Louis, MO, USA).
Extraction of EO
Initially, 50 g of plant sample was mixed with 500 mL of distilled water. They were hydrodistillated for 3 h by using a Clevenger-type apparatus. When the condensed material cooled down, the water and EO were separated and the oil was decanted to be used as EO. The ultimate yields of B. persicum and R. officinalis EOs were 1.97 ± 0.28 and 1.40 ± 0.10%, respectively. EO samples were dried over anhydrous sodium sulphate and were stored in sealed vials at –18°C for further use.[Citation12]
Gas Chromatography (GC) Analysis of EOs
The identification of EO constituents was carried out by using a GC (7890A, Agilent Technologies, Santa Clara, CA) which was coupled with a mass spectrometer (5975C, Agilent Technologies, Santa Clara, CA), operating at 70 eV ionization energy, 0.5 s/scan, and a mass range of 35–400 atomic mass units (amu). It was equipped with a HP-5MS capillary column (5% Phenyl Polysilphenylene-siloxane; 30 m length; 0.25 mm internal diameter; 0.25 μm film thickness). One microliter of the EO sample was injected into the gas chromatography/mass spectrometry (GC/MS) in split mode (split ratio: 1/100). Helium was used as the carrier gas with a flow rate of 0.9 mL/min. The injector and detector temperatures were set at 280°C. The oven temperature was programmed to start at 60°C and gradually heated up to a temperature of 210°C at a rate of 3°C/min. Thereafter, the rate of temperature elevation became 20°C/min up to 240°C, whereupon the temperature was held constant for 8.5 min. The MSD ChemStation Software (G1701EA, E.02.01.1177, Agilent Technologies, Santa Clara, CA) was applied to analyze mass spectra and chromatograms. The compounds were identified by comparing their mass spectral fragmentation patterns with those in the data bank (Wiley/NBS). Comparisons were also made with mass spectral data derived from the relevant literature.[Citation4,Citation5,Citation9,Citation12–Citation17] Furthermore, the EO constituents were analyzed qualitatively under the same chromatographic conditions via a GC coupled with a flame ionization detector (FID). Relative percentage data were obtained from electronic integration of chromatogram peak areas, as a modified method taken from a study by Gavahian et al.[Citation18]
AA
Antioxidant activities of EOs were evaluated based on free radical scavenging capacities and their reducing powers. The free radical scavenging capacities of EOs were measured by using DPPHº (2,2-diphenyl-1-picrylhydrazyl radical) as described by Mimica-Dukić et al.[Citation19] The EOs were examined with regard to their radical scavenging activity on DPPHº and, therefore, the radical scavenging capacity was established. At concentrations of 0.01, 0.1, 1, and 10 mg/mL, 3 mL of methanolic solution of the EOs were mixed with 1 mL of DPPHº solution (0.2 mM). The mixtures were left to stand at room temperature for 1 h in the dark. Then, the absorbance values were measured at 517 nm against blank. The same procedure was repeated with BHT as a positive control. Concentrations of samples that were able to cause inhibitions by 50% (IC50) were determined from the equation for plotted curves which correspond to the levels of inhibition.
The cupric ion-reducing power of the EOs and the positive standard (L-ascorbic acid) were determined via a solution containing 1 mL CuCl2 (0.01 M), 1 mL neocuproine methanolic liquid (7.50 × 10−3 M) and 1 ml ammonium acetate aqueous buffer liquid (1.00 M). Then, 0.50 mL of the EO solution and 0.60 mL of H2O were added to the initial mixture so as to make the final volume reach 4.10 mL. The tubes were stoppered and, after 30 min, the absorbance was recorded at 450 nm (A450) against blank.[Citation20] Results were expressed as mg of ascorbic acid equivalents per g of sample.
Initial Characteristics of VOO
Free fatty acid content
Free fatty acid was determined following the American Oil Chemists’ Society (AOCS) official method (Cd 3d-63) and was expressed as the percentage of oleic acid.[Citation21]
Fatty acid composition of VOO
Fatty acid composition of VOO was determined according to the method described by Golmakani et al.[Citation22] with some modifications. Two hundred milligrams of VOO was treated with 10 mL of methanol: acetyl chloride (95:5, v/v) solution. The mixture was sealed in a 20 mL polytetrafluoroethylene (PTFE)-lined vial and heated at 85°C for 1 h. The vial was cooled, whereupon 5.00 mL of double distilled water was added to the vial which was shaken vigorously for 5 min. Thereafter, 1.00 mL of hexane––containing 0.01% tertiary butylhydroquinone (TBHQ)––was added to the mixture and shaken vigorously for 5 min. The TBHQ was added to the mixture in order to prevent the oxidation of double bonds during the isolation procedure and the storage of VOO. Finally, 2.00 mL of hexane, containing 0.01% TBHQ, was added and the mixture was centrifuged at 4000 × g at 25°C for 5 min. The upper phase comprised the hexane layer which contained mixtures of fatty acid methyl esters. The hexane layer was transferred to a clean vial and was stored at 4°C prior to injection.
The fatty acid composition of the VOO sample was analyzed by using a GC system (SP-3420A, Beijing Beifen-Ruili analytical instrument, China). The system was equipped with a split/splitless injector, a FID and a BPX70 capillary column (Bis–cyanopropylsiloxane-silphenylene, 30 m × 0.25 mm internal diameter; 0.25 μm film thickness). The column temperature program started from 140°C, but was held at 140°C for 5 min, and was then increased to 180°C at a rate of 20°C/min. The temperature was held again at 180°C for 9 min and then was increased to 200°C, at a rate of 20°C/min. The temperature was finally held at 200°C for 3 min. The temperatures of the injector and detector were set at 250 and 300°C, respectively. Nitrogen was used as the carrier gas. One microliter of fatty acid methyl esters was injected into the column with a split ratio of 1:10. Fatty acids of the samples were identified according to the retention times of standard fatty acids injected under the same operating conditions. The quantitative determination of fatty acids was carried out by calculating their relative peak areas.
Total Phenolic Content
Phenolic compounds were isolated from a solution of oil in hexane by triple-extraction with water–methanol (60:40 v/v), and were estimated with Folin–Ciocalteu reagent at 725 nm. Results were expressed as mg of gallic acid per gram of VOO.[Citation23]
Chlorophyll and Carotenoid Contents
Chlorophyll content was determined at 670 nm according to the method described by Minguez-Mosquera et al.[Citation24] The total carotenoid content was determined at 470 nm by using the British Standard Methods of Analysis.[Citation25]
Oxidation Indices of VOO
The peroxide value (PV) was determined as described in the AOCS official method (Cd 8-53) and expressed as milliequivalents of active oxygen per kg of VOO.[Citation21] The p-Anisidine value (AV) was determined by using the AOCS official method (Cd 8-53) and was expressed as mg per kg of VOO.[Citation21] The TOTOX (total oxidation value; AV + 2 PV) is used as an empirical measure that quantifies the precursor non-volatile carbonyls which are present in the processed oils. Any further oxidation product that is developed after storage can also be quantified by TOTOX.[Citation26] The K232 and K268 extinction coefficients were determined according to the AOCS official method (Chapters 5–91) by measuring the absorbance of a 1% solution in isooctane at 232 and 268 nm with 1 cm pass length.[Citation21]
Accelerated Storage of VOO
EOs of B. persicum and R. officinalis were prepared at 1000 ppm concentration and were added to the VOO. BHT and β-carotene were prepared at the concentration of 100 ppm and were added to VOO. Furthermore, the mixtures of EOs, BHT, and β-carotene were combined with 100 ppm of CA, then to be added to the VOO. After mixing them altogether, the samples were transferred separately into amber bottles (60 mL). The bottles were completely filled and sealed. No headspace was left in the bottles. Then samples were stored in an incubator at 60 ± 1°C for 16 days. Chemical indicators of lipid oxidation, namely PV, AV, K232, and K268 were measured every 4 days. Chlorophyll and carotenoid contents were measured at the beginning and at the end of storage. The protection factor (PF) was expressed according to Eq. (1).
where IPinh is the induction period (IP) of the VOO sample with any of the inhibitors (BHT, β-carotene, or EOs) and IPc is the IP of the control sample.[Citation27] IP is estimated to be the time required for a sample to reach a PV of 20 meq O2/kg—the value overcoming the maximum permitted limit—which is consequently expected to lose the classification of VOO category.[Citation15,Citation28] AA is a function of an antioxidant’s concentration. AA was calculated according to Eq. (2).
where [AH] is the concentration of the antioxidant in proper units.[Citation29] Antioxidant power (AOP) is expressed as a value between 0 and 100; the greater the AOP, the stronger the antioxidant capacity. AOP was calculated according to Eq. (3).[Citation30]
Percent of synergism was calculated according to Eq. (4).[Citation27]
where IPm is the IP of the sample containing the combination of an inhibitor and CA, IP1 is the IP of the sample containing the inhibitor without CA, and IP2 is the IP of the sample containing CA.
Statistical Analysis
All experiments were performed in triplicate and the data were reported as mean values of the measurements. Standard deviation values were presented in the tables and the standard deviation bars were provided in the figures. A general linear model (GLM) procedure from Statistical Analysis Software (SAS, version 9.1; SAS Institute Inc. Cary, NC) was used for the comparison of mean values. The simple regression equations of the chemical variables, obtained from the storage study of VOO (PV, K232, and K268), were calculated by Microsoft Office Excel 2010.
RESULTS AND DISCUSSION
GC Analysis of EO
The chemical compositions of B. persicum and R. officinalis EOs are presented in . Three main components of B. persicum EO were monoterpene hydrocarbons (γ-terpinene [17.24%] and p-cymene [12.85%] and benzaldehydes including cuminaldehyde [33.13%]). An earlier study by Shahsavari et al.[Citation9] claims that the main components of Iranian B. persicum EO are γ-terpinene, cuminaldehyde, p-cymene, and limonene. Also, the major constituents of B. persicum EO from Tajikistan are reported as p-mentha-1,4-dien-7-al, γ-terpinene, β-pinene, and cuminaldehyde.[Citation31] Variations in chemical compositions of EOs is influenced by climatic conditions, thereby altering their biological activities.
TABLE 1 Chemical composition of Bunium persicum and Rosmarinus officinalis essential oil
The major components of R. officinalis EO were monoterpene hydrocarbons such as α-pinene (16.10%), camphene (6.26%), limonene (10.36%), and oxygenated monoterpenes including ketones such as camphor (4.14%) and verbenone (10.80%), Esters such as bornyl acetate (14.65%), alcohol of borneol (11.72%), and the ether 1,8-cineol (7.50%). The major hydrocarbon sesquiterpene of R. officinalis EO is (E)-caryophyllene (2.72%). Sacchetti et al.[Citation17] reported that the R. officinalis of Sardinian ecotype is rich in verbenone, camphor, bornyl acetate, and borneol. The Sardinian ecotype of R. officinalis shows better AA than those reported by Baratta et al.[Citation8] who worked on samples of other R. officinalis ecotypes with regard to their α-pinene/1,8-cineole/camphor chemotype.
AA of EOs
The DPPHº assay is considered a simple and very fast method for determining AA. In contrast, DPPHº can only be dissolved in organic media, and this makes it a notable limitation when interpreting the role of hydrophilic antioxidants.[Citation31] B. persicum and R. officinalis EOs reduced the concentration of DPPHº substantially, allowing IC50 values to reach 2.71 ± 0.19 and 3.67 ± 0.33 mg/mL in the mentioned EOs, respectively. These findings are in agreement with earlier published data on the profound antiradical activity of both EOs.[Citation4,Citation17] The B. persicum EO shows stronger radical scavenging capacity than the EO of R. officinalis. EOs with higher amounts of monoterpene hydrocarbons were reported to be almost ineffective.[Citation17] This result is in accordance with the poor performance exhibited by EOs with similar characteristics and by single monoterpene hydrocarbons.[Citation6]
The cupric ion reducing assay can simultaneously measure hydrophilic and lipophilic antioxidants.[Citation20] The cupric ion reducing power of B. persicum and R. officinalis EOs were 516.96 ± 8.84 and 453.57 ± 7.58 mg of ascorbic acid equivalents per gram of the sample, respectively. Thus, both EOs show relevant degrees of hydrogen-donating capacity, but the capacities were, as expected, less than the capacity of ascorbic acid. In agreement with the DPPHº scavenging capacity results, the B. persicum EO shows stronger reducing power than R. officinalis EO.
Initial Characteristics of VOO
The free acidity of VOO was 1.71 ± 0.22% at the beginning of the assay, which was below the upper legal limit established by the International Olive Council (IOC) for VOO (free acidity ≤ 2.0%).[Citation28] The indices referring to primary and secondary oxidation products (PV = 4.45 ± 0.68 meq O2/kg, K232 = 1.67 ± 0.25 and K268 = 0.16 ± 0.10) were well below the upper legal limit established by IOC (PV [≤20], K232 [≤2.6] and K268 [≤0.25]) for VOO, indicating a low initial oxidation status. The VOO used in this study contained high amounts of phenolic compounds (294.5 ± 1.1 mg of gallic acid per g of VOO). These phenolic compounds mainly act by chain-breaking via donating a radical hydrogen to alkyl-peroxy-radicals formed during the propagation step of lipid oxidation, but which subsequently form a stable radical.[Citation2] Chlorophyll and carotenoid contents of VOO were 4.70 ± 0.07 and 2.05 ± 0.06 mg/kg, respectively. In addition to polyphenols, the chlorophyll and carotenoids play important roles in the oxidative stability of VOO and are mainly responsible for the color quality of VOO. The color can vary from yellow-green to greenish-gold.[Citation33] The fatty acid composition of VOO is presented in . Fatty acids in the VOO were namely palmitic (18.71 ± 2.68%), stearic (1.57 ± 0.14%), oleic (68.85 ± 1.70%), linoleic (9.4 ± 2.2%), and linolenic acid (1.22 ± 0.63%). The ratio of mono-unsaturated to poly-unsaturated fatty acids in the VOO is 6.48. High contents of natural antioxidants and low levels of poly-unsaturated fatty acids signify that the VOO is resistant to oxidation. However, the presence of linoleic acids and small amounts of linolenic acids make VOO susceptible to oxidation.
TABLE 2 Fatty acid composition of virgin olive oil
Oxidative Stability of VOO Samples
PV, AV, and TOTOX value
Primary oxidation products, i.e., hydroperoxides, were determined by PV measurement. The PVs of VOO samples during storage at 60°C are presented in and . The PV of the control sample rose faster than those containing natural and synthetic antioxidants. The PV of the control reached 19.59 ± 0.08 meq O2/kg after the VOO was stored for 8 days at 60°C. By increasing the rate at which hydroperoxides decompose into secondary products, the PV began to decline after the VOO was stored for 12 days.
FIGURE 1 Changes in (a,b): peroxide values, (c,d): p-anisidine values, and (e,f): TOTOX values of virgin olive oil samples during storage at 60°C with (b,d,f) and without (a,c,e) citric acid.
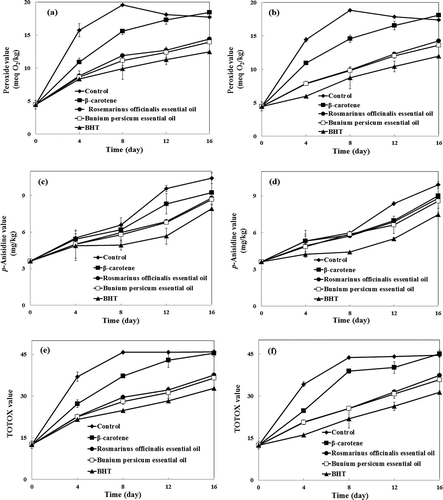
The samples containing B. persicum and R. officinalis EOs had PVs which exhibited almost identical increasing trends during the storage of VOO for 16 days at 60°C. The effect of B. persicum and R. officinalis EOs on retarding the formation of hydroperoxides was slightly less than that of BHT. These results are partially in agreement with the findings of Shahsavari et al.[Citation9] on soybean oil. They reported that the AA of B. persicum EO at 0.06% is similar to that of BHA at 0.02%.
VOO samples containing β-carotene showed higher PVs than those containing EOs and BHT during storage at 60°C. Lee et al.[Citation34] showed that β-carotene does not work as an antioxidant in soybean oil when stored in the dark. Also, Haila and Heinonen[Citation35] reported that during the storage of purified rapeseed oil in open bottles and in the presence of light, β-carotene acted as a pro-oxidant. A strong relationship has been reported between the anti-oxidative effect of β-carotene as a chain-breaking agent and oxygen tensions in the lipid environment. At higher oxygen tensions, β-carotene loses its activity and plays a role as pro-oxidant.[Citation36] However, we did not find any pro-oxidant effect of β-carotene mixed with VOO in closed amber bottles during their storage at 60°C. The PVs of samples containing natural or synthetic antioxidants combined with CA were lower than those containing natural or synthetic antioxidants without CA. Similarly, Assimopoulou et al.[Citation11] reported that the combination of P. lentiscus resin with CA can significantly reduce the oxidation of sunflower oil.
The anisidine test is designed to measure high molecular weights of saturated and unsaturated carbonyl compounds in triacylglycerols.[Citation26] The results of AVs concerning VOO samples during their storage at 60°C are presented in and . AV of the control sample increased throughout the storage time, which increased more rapidly after the VOO was stored for 12 days. In particular, this can be linked to the rate of hydroperoxide decomposition which exceeded the rate of their formation at that time. All VOO samples exhibited significantly lower AVs than the control during their storage at 60°C. After storing the VOO for 16 days, however, the AVs of samples containing BHT, β-carotene, B. persicum EO, and R. officinalis EO reached 7.92 ± 0.25, 9.23 ± 1.03, 8.68 ± 0.40, and 8.81 ± 0.16 mg/kg, respectively. Thus, B. persicum and R. officinalis EOs were slightly weaker than BHT in retarding the formation of secondary oxidation products, whereas both EOs acted more efficiently than β-carotene. Combinations of natural and synthetic antioxidants with CA had a synergistic effect on preventing the formation of secondary oxidation products.
The TOTOX value measures primary and secondary products through the oxidation process. Results of the TOTOX values of VOO samples during their storage at 60°C are presented in and . The addition of BHT, β-carotene, B. persicum EO, and R. officinalis EO caused significant reductions in TOTOX values of VOO samples that were stored for 16 days at 60°C. There was no significant difference between the TOTOX values of samples containing B. persicum EO and those containing R. officinalis EO (p < 0.05). The effects of B. persicum and R. officinalis EOs on delaying the primary and secondary oxidation of VOO were comparable to that of BHT. These results indicate that B. persicum and R. officinalis EOs are good sources of active compounds which can retard the primary and secondary oxidation changes in VOO. The main components of B. persicum EO were monoterpene hydrocarbons (γ-terpinene and p-cymene) and cuminaldehyde. Ruberto and Baratta[Citation6] reported that γ-terpinene has significant protective action against lipid oxidation. The presence of strongly activated methylene groups in γ-terpinene is probably the reason for this behavior, which may compete with the activated methylene in C-11 of linoleic acid. Moreover, p-cymene is found to be less effective than γ-terpinene. They also found that, among the main components in the EO of R. officinalis, limonene, 1,8-cineol, and bornyl acetate were more effective than the other components. Also, borneol and camphene exhibited lowest AA.
β-carotene was less effective than BHT and EOs in delaying the primary and secondary oxidation of VOO. Karabulut[Citation37] reported that a pro-oxidant effect is observed when β-carotene alone is added to butter oil triacylglycerols, at certain concentrations. However, it behaves as an antioxidant for inhibiting the lipid peroxidation, when used along with α-tocopherol. The synergism between β-carotene and α-tocopherol is attributed to the rapid consumption of α-tocopherol in comparison with β-carotene. Via this mechanism, α-tocopherol is supposed to protect β-carotene from oxidation.[Citation38] It seems that the AA of β-carotene in VOO may be due to the synergistic effect between β-carotene and natural tocopherols of VOO.
IP, PF, AA, and AOP for all tested antioxidants are presented in . IP values of all samples treated with natural and synthetic antioxidants were significantly higher than that of the control. The IP of the sample containing B. persicum EO is found here to be slightly higher than that of R. officinalis EO (p < 0.05). IP values of VOO samples treated with EOs were lower than those treated with BHT. Generally, EOs were more effective than β-carotene. These findings are consistent with results by Yagci et al.[Citation39] who found that the IP values of corn and palm oils significantly increased in the presence of Thymbra spicata EO. Also, T. spicata EO was more effective than BHT.
The effectiveness of the two antioxidants was compared with each other according to their PF. The following scale is proposed for the PF values: 1.0–1.5 (very low), 1.5–2.0 (low), 2.0–2.5 (medium), 2.5–3.0 (high), and >3.0 (very high).[Citation40] The PF values for BHT, B. persicum and R. officinalis EOs and also their combinations with CA measured to be more than three. Thus, these antioxidants exhibited a “very high” antioxidant capacity in VOO. Similarly, Samotyja and Malecka[Citation10] reported that the IP values of samples containing rosemary and blackcurrant seed extracts were 3.5-fold higher than that of the control. The β-carotene and its combination with CA exhibited a “medium” antioxidant capacity (PF of 2.0–2.5).
AA is a function of antioxidant concentration. AA would not increase if it is directly proportional to the concentration of the antioxidant. Also, regarding the VOO sample containing the antioxidant, AA would be zero if the time taken to reach the predetermined level of oxidation comes to equal the time for the control sample to reach the same level of oxidation. Also, AA would become larger if reaching the predetermined level of oxidation takes a longer time by VOO samples containing the antioxidant. Furthermore, a negative result would denote a pro-oxidative action.[Citation29]
The AA of all evaluated antioxidants is discovered to be more than zero in this study. Therefore, none of the samples here indicate a pro-oxidative action. In this study, since EOs retarded the oxidation of VOO at higher concentrations, the AA values of EOs were significantly lower than those of the BHT and β-carotene. Similarly, Hashemi et al.[Citation15] reported that the AA of Carum copticum EO is significantly lower than the AA of BHT and BHA. Nonetheless, there is a legal level for the maximum amount of synthetic food additives that can be used. This is established based on various toxicological analyses. However, the B. persicum and R. officinalis EOs could be used at higher levels than their synthetic counterparts because they originate from natural sources. Aside from these argumentations, no significant difference was observed between the AA of VOO samples containing EOs that were combined with CA and those containing EOs without CA (p < 0.05).
According to AOP results, BHT, B. persicum and R. officinalis EOs and their combinations with CA exhibited higher AOP values than β-carotene in VOO that were stored at 60°C. AOP of B. persicum EO was slightly higher than that of R. officinalis EO (p < 0.05). Ruberto and Baratta[Citation6] showed that the antioxidant capability of the main compounds in the B. persicum EO, i.e., γ-terpinene and p-cymene, is significantly higher than the AA of compounds in the R. officinalis EO, namely α-pinene, camphor, bornyl acetate, and 1,8-cineol. Therefore, the main components do not always determine the antioxidant capability of the examined EO. The antioxidant capability of the main compounds can be modulated by other components in the EO.[Citation14]
Percentages of synergisms relating to tested antioxidants that were used in combination with CA are illustrated in . The percentages of synergisms were 2.42, 2.83, 2.42, and 2.03%, respectively, for BHT, B. persicum EO, R. officinalis EO, and β-carotene. This clearly indicates the synergism efficiency brought by CA. It is also worthy of note that CA exhibited similar synergism with BHT, B. persicum EO, R. officinalis EO, and β-carotene in VOO. Parallel to these arguments, Banias et al.[Citation41] reported that CA shows a high synergistic efficiency when it is in combination with marjoram extract. However, they showed that CA exhibits a minor synergy with thyme extract, whereas it produces negative effects when combined with oregano, dittany, rosemary, and sage extracts in lard.
TABLE 3 Oxidative stability and synergism percentage of VOO samples during storage at 60°C
Determination of K232 and K268
The formation of conjugated dienes in fats or oils gives rise to an absorption peak at 232 nm in the ultraviolet region.[Citation29] The K232 values of VOO samples during their storage at 60°C are illustrated in and . The absorption at 232 nm increased gradually by increasing the storage time. In agreement with the PV results, VOO samples containing B. persicum and R. officinalis EOs occur to have lower levels of conjugated oxidative products than VOO samples containing β-carotene and the control. The effects of B. persicum and R. officinalis EOs on preventing the formation of conjugated dienes are comparable to BHT.
FIGURE 2 Changes in (a,b): K232 and (c,d): K268 of virgin olive oil samples during storage at 60°C with (b,d) and without (a,c) citric acid.
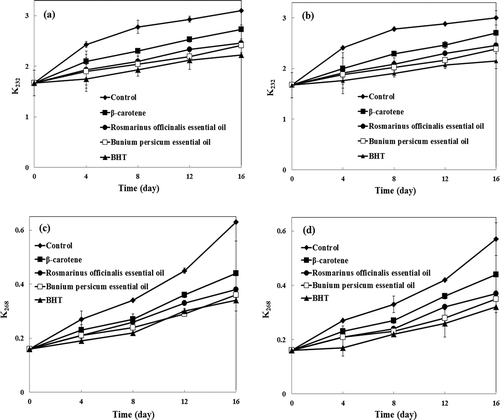
Changes in K268, which are due to the formation of conjugated trienes as well as unsaturated ketones and aldehydes, are presented in and . When VOO samples were stored for 16 days at 60°C, the levels of conjugated trienes were lowest in samples containing BHT and B. persicum EO, followed by those of R. officinalis EO and β-carotene, while the highest levels were found in the control sample. CA showed a minor synergistic effect in combination with natural and synthetic antioxidants in preventing the formation of conjugated dienes and trienes.
The duration required to reach the upper legal limits of K232 and K268 for VOO samples during their storage period are presented in . As expected, the durations required to reach the upper legal limit of K232 and the IP value correlated directly (R2 = 0.933; y = 0.817x + 0.804 and R2 = 0.975; y = 0.763x + 1.514 for VOO samples with and without CA, respectively). Also, there was a direct correlation between the durations required to reach the upper legal limit of K268 and the IP value (R2 = 0.995; y = 0.265x + 1.553 and R2 = 0.943; y = 0.216x + 2.085 for VOO samples with and without CA, respectively). Moreover, the durations required to reach the upper legal limit of K232 correlated directly with the duration to reach the legal limit of K268 (R2 = 0.995; y = 0.322x + 1.340 and R2 = 0.940; y = 0.280x + 1.718 for VOO samples with and without CA, respectively).
TABLE 4 Times required to reach the upper legal limits of K232 and K268 for virgin olive oil samples during storage at 60°C
In most of the VOO samples, K232 and PV reached their upper legal limits almost at the same time. IP values of VOO samples were about 0.95–1.33 times higher than the durations required for K232 to reach its upper legal limit. K268 was the first index that exceeded the upper legal limit established by the IOC for VOO (0.25). IP values of VOO samples were about 2.05–3.93 times higher than the durations required for K268 to reach its upper legal limit. In fact, as we already know, linolenic acid is the fatty acid in VOO which is most susceptible to auto-oxidation and its hydroperoxides undergo rapid decomposition. This decomposition yields a number of compounds, some of which absorb ultraviolet radiation at 268 nm.[Citation42] Also, Gomez-Alonso et al.[Citation42] reported that the linolenic acid decreased more than linoleic acid in VOO during 21 months of storage at room temperature. The decrease in linolenic acid was 2.5–2.8 times greater than that of linoleic acid. Oxidative stability ranking order of K232 which were derived by using the durations required to reach its upper legal limit were identical to that of K268 (BHT > B. persicum EO > R. officinalis EO > β-carotene > control).
Chlorophyll and Carotenoid Contents
Chlorophyll compounds play an important role in the oxidative stability of VOO due to their antioxidant nature in the dark and their pro-oxidative activity in the light.[Citation33] Carotenoids can act as primary antioxidants by trapping free radicals or they can act as secondary antioxidants by quenching singlet oxygen.[Citation43] Changes in chlorophyll and carotenoid contents of VOO samples at the beginning and at the end of storage period are presented in . Chlorophyll and carotenoid contents in all VOO treatments decreased during storage. In all VOO samples, carotenoid contents decreased more than chlorophyll contents during the storage of VOO at 60°C. Similarly, Kiralan and Bayrak[Citation44] reported that the carotenoid fraction decreases faster than the chlorophyll fraction of the VOO when the VOO is stored at 60°C. Samples treated with BHT and B. persicum EO had the highest chlorophyll content after being stored for 16 days at 60°C. The decrease in the chlorophyll percentage of VOO samples containing R. officinalis EO was significantly higher than that of B. persicum EO at the end of storage, whereas there was no significant difference between the B. persicum EO and R. officinalis EO regarding the decrease in carotenoid percentage at the end of storage. This phenomenon can be explained by the fact that the activity of antioxidants differs depending on the oxidizing substrate.[Citation42] β-carotene was less effective than BHT, B. persicum EO and R. officinalis EO in protecting chlorophyll and carotenoid contents of VOO samples. The decrease in the carotenoid content of samples containing β-carotene was higher than that of the control, which may be due to the fact that β-carotene is consumed in chemical reactions when added to the VOO. With regard to the decrease in chlorophyll and carotenoid contents, there was no significant difference between the VOO samples containing mixtures of natural and synthetic antioxidants with CA and those without CA (p < 0.05).
TABLE 5 Changes in chlorophyll and carotenoid contents (mg/kg) of virgin olive oil samples at the beginning and end of storage
CONCLUSION
The results of the present study show that B. persicum and R. officinalis EOs can improve the oxidative stability of VOO during its storage. The effect of B. persicum and R. officinalis EOs on retarding the process of oxidation in VOO is comparable to that of BHT. Since BHT cannot be legally used in VOO, the EOs still compete fairly well in regard to efficiency. Both EOs improved the stability of VOO to a greater extent than β-carotene. Furthermore, the combinations of natural/synthetic antioxidants and CA led to the higher oxidative stability of VOO samples. Therefore, in general, B. persicum and R. officinalis EOs can be recommended as natural antioxidants to be used for improving the VOO shelf life.
NOMENCLATURE
AA: | = | Antioxidant activity |
AOP: | = | Antioxidant power |
AV: | = | p-Anisidine value |
BHA: | = | Butylatedhydroxyanisole |
BHT: | = | Butylatedhydroxytoluene |
CA: | = | Citric acid |
EO: | = | Essential oil |
IP: | = | Induction period |
PV: | = | Peroxide value |
PF: | = | Protection factor |
TBHQ: | = | Tertiary butylhydroquinone |
VOO: | = | Virgin olive oil |
ACKNOWLEDGMENTS
We would like to thank the Edible Oil Industries Group of Etka Organization for providing the VOO. We also thank Mohsen Hamedpour-Darabi for his native English editing service.
FUNDING
This research project was financially supported by Shiraz University.
Additional information
Funding
REFERENCES
- Nogala-Kalucka, M.; Korczak, J.; Dratwia, M.; Lampart-Szczapa, E.; Siger, A.; Buchowski, M. Changes in Antioxidant Activity and Free Radical Scavenging Potential of Rosemary Extract and Tocopherols in Isolated Rapeseed Oil Triacylglycerols During Accelerated Tests. Food Chemistry 2005, 93, 227–235.
- Velasco, J.; Dobarganes, C. Oxidative Stability of Virgin Olive Oil. European Journal of Food Science and Technology 2002, 104, 661–676.
- Madhav, D.L; Salunkhe, D.K. Toxicological Aspects of Food Antioxidants. In Food Antioxidants; Madhavi, D.L., Deshpande, S.S., Salunkhe, D.K., Eds.; Marcel Dekker: New York, NY, 1995; 267–360.
- Sharififar, F.; Yassa, N.; Mozaffarian, V. Bioactivity of Major Components from the Seeds of Bunium Persicum (Boiss.) Fedtch. Pakistan Journal of Pharmaceutical Sciences 2010, 23, 300–304.
- Azizi, M.; Davareenejad, G.; Bos, R.; Woerdenbag, H.J.; Kayser, O. Essential Oil Content and Constituents of Black Zira (Bunium Persicum [Boiss.] B. Fedtsch.) from Iran During Field Cultivation (Domestication). Journal of Essential Oil Research 2009, 21, 78–82.
- Ruberto, G.; Baratta, M.T. Antioxidant Activity of Selected Essential Oil Components in Two Lipid Model Systems. Food Chemistry 2000, 69, 167–174.
- Boutekedjiret, C.; Bentahar, F.; Belabbes, R.; Bessiere, J. Extraction of Rosemary Essential Oil by Steam Distillation and Hydrodistillation. Flavour and Fragrance Journal 2003, 18, 481–484.
- Baratta, M.T.; Dorman, H.D.; Deans, S.G.; Biondi, D.M.; Ruberto, G. Chemical Composition, Antimicrobial, and Antioxidative Activity of Laurel, Sage, Rosemary, Oregano, and Coriander Essential Oils. Journal of Essential Oil Research 1998, 10, 618–627.
- Shahsavari, N.; Barzegar, M.; Sahari, M.A.; Naghdibadi, H. Antioxidant Activity and Chemical Characterization of Essential Oil of Bunium Persicum. Plant Foods for Human Nutrition 2008, 63, 183–188.
- Samotyja, U.; Małecka, M. Antioxidant Activity of Blackcurrant Seeds Extract and Rosemary Extracts in Soybean Oil. European Journal of Lipid Science and Technology 2010, 112, 1331–1336.
- Assimopoulou, A.; Zlatanos, S.; Papageorgiou, V. Antioxidant Activity of Natural Resins and Bioactive Triterpenes in Oil Substrates. Food Chemistry 2005, 92, 721–727.
- Đorđević, A.; Palić, I.; Stojanović, G.; Ristić, N.; Palić, R. Chemical Profile of Satureja Kitaibelii Wierzb. ex Heuff. Essential Oils: Composition of Satureja Kitaibelii Essential Oils. International Journal of Food Properties 2014, 17, 2157–2165.
- Adams, R.P. Identification of Essential Oil Components by Gas Chromatography/Mass Spectrometry; Allured Publishing Corporation: Carol Stream, IL, 2007; 804.
- Dawidowicz, A.L.; Olszowy, M. Does Antioxidant Properties of the Main Component of Essential Oil Reflect Its Antioxidant Properties? The Comparison of Antioxidant Properties of Essential Oils and Their Main Components. Natural Product Research 2014, 28, 1952–1963.
- Hashemi, M.B.; Niakousari, M.; Saharkhiz, M.J.; Eskandari, M.H. Stabilization of Sunflower Oil with Carum Copticum Benth and Hook Essential Oil. Journal of Food Science and Technology 2014, 51, 142–147.
- Mazidi, S.; Rezaei, K.; Golmakani, M.-T.; Sharifan, A.; Rezazadeh, Sh. Antioxidant Activity of Essential Oil from Black Zira (Bunium Persicum Boiss.) Obtained by Microwave-Assisted Hydrodistillation. Journal of Agricultural Science and Technology 2012, 14, 1013–1022.
- Sacchetti, G.; Maietti, S.; Muzzoli, M.; Scaglianti, M.; Manfredini, S.; Radice, M.; Bruni, R. Comparative Evaluation of 11 Essential Oils of Different Origin As Functional Antioxidants, Antiradicals, and Antimicrobials in Foods. Food Chemistry 2005, 91, 621–632.
- Gavahian, M.; Farahnaky, A.; Javidnia, K.; Majzoobi, M. Comparison of Ohmic-Assisted Hydrodistillation with Traditional Hydrodistillation for the Extraction of Essential Oils from Thymus Vulgaris L. Innovative Food Science and Emerging Technologies 2012, 14, 85–91.
- Mimica-Dukić, N.; Bozin, B.; Soković, M.; Mihajlović, B.; Matavulj, M. Antimicrobial and Antioxidant Activities of Three Mentha Species Essential Oils. Planta Medica 2003, 69, 413–419.
- Apak, R.; Güçlü, K.; Özyürek, M.; Çelik, S.E. Mechanism of Antioxidant Capacity Assays and the CUPRAC (Cupric Ion Reducing Antioxidant Capacity) Assay. Microchimica Acta 2008, 160, 413–419.
- AOCS. Official Methods and Recommended Practices of the American Oil Chemists’ Society; AOCS Press: Champaign, IL, 2000.
- Golmakani, M.-T.; Mendiola, J.A.; Rezaei, K.; Ibáñez, E. Expanded Ethanol with CO2 and Pressurized Ethyl Lactate to Obtain Fractions Enriched in γ-Linolenic Acid from Arthrospira Platensis (Spirulina). The Journal of Supercritical Fluids 2012, 62, 109–115.
- Casal, S.; Malheiro, R.; Sendas, A.; Oliveira, B.P.; Pereira, J.A. Olive Oil Stability Under Deep-Frying Conditions. Food and Chemical Toxicology 2010, 48, 2972–2979.
- Minguez-Mosquera, M.I.; Gandul-Rojas, B.; Garrido-Fernandez, J.; Gallardo-Guerrero, L. Pigments Present in Virgin Olive Oil. Journal of the American Oil Chemists’ Society 1990, 67, 192–196.
- British Standard Methods of Analysis. Determination of carotene in vegetable oils, B.S684, section 2.20. Oxford, 1993.
- Frankel, E. N. Lipid Oxidation; Woodhead Publishing Limited: Cambridge, UK, 2012; 470.
- Hraš, A.R.; Hadolin, M.; Knez, Ž.; Bauman, D. Comparison of Antioxidative and Synergistic Effects of Rosemary Extract with α-Tocopherol, Ascorbyl Palmitate and Citric Acid in Sunflower Oil. Food Chemistry 2000, 71, 229–233.
- IOC. Trade Standard Applying to Olive Oils and Olive-Pomace Oils. Decision COI/T.15/NC No 3/Rev. 8. International Olive Council, Madrid, Spain, 2015.
- Antolovich, M.; Prenzler, P.D.; Patsalides, E.; McDonald, S.; Robards, K. Methods for Testing Antioxidant Activity. Analyst 2002, 127, 183–198.
- Silva, F.A.; Borges, F.; Ferreira, M.A. Effects of Phenolic Propyl Esters on the Oxidative Stability of Refined Sunflower Oil. Journal of Agricultural and Food Chemistry 2001, 49, 3936–3941.
- Baser, K.; Oezek, T.; Abduganiev, B.; Abdullaev, U.; Aripov, K.N. Composition of the Essential Oil of Bunium Persicum (Boiss.) B. Fedtsch. from Tajikistan. Journal of Essential Oil Research 1997, 9, 597–598.
- Wojdyło, A.; Oszmiański, J.; Czemerys, R. Antioxidant Activity and Phenolic Compounds in 32 Selected Herbs. Food Chemistry 2007, 105, 940–949.
- Criado, M.-N.; Romero, M.-P.; Casanovas, M.; Motilva, M.-J. Pigment Profile and Colour of Monovarietal Virgin Olive Oils from Arbequina Cultivar Obtained During Two Consecutive Crop Seasons. Food Chemistry 2008, 110, 873–880.
- Lee, J.; Ozcelik, B.; Min, D. Electron Donation Mechanisms of β‐Carotene As a Free Radical Scavenger. Journal of Food Science 2003, 68, 861–865.
- Haila, K.; Heinonen, M. Action of β-Carotene on Purified Rapeseed Oil During Light Storage. LWT–Food Science and Technology 1994, 27, 573–577.
- Burton, G.W.; Ingold, K. U. Beta-Carotene: An Unusual Type of Lipid Antioxidant. Science 1984, 224, 569–573.
- Karabulut, I. Effect of α-Tocopherol, β-Carotene and Ascorbyl Palmitate on Oxidative Stability of Butter Oil Triacylglycerols. Food Chemistry 2010, 123, 622–627.
- Palozza, P.; Krinsky, N. I. β-Carotene and α-Tocopherol Are Synergistic Antioxidants. Archives of Biochemistry and Biophysics 1992, 297, 184–187.
- Yagcı, S.; Yagcı, E.; Gogus, F. Antioxidative Effect of Thymbra spicata on Oxidative Stability of Palm and Corn Oils. International Journal of Food Properties 2012, 15, 656–664.
- Ahmad, M.; Al‐Hakim, S.; Shehata, A. The Behaviour of Phenolic Antioxidants, Synergists, and Their Mixtures in Two Vegetable Oils. Fette, Seifen, Anstrichmittel 1983, 85, 479–483.
- Banias, C.; Oreopoulou, V.; Thomopoulos, C. The Effect of Primary Antioxidants and Synergists on the Activity of Plant Extracts in Lard. Journal of the American Oil Chemists’ Society 1992, 69, 520–524.
- Gómez-Alonso, S.; Mancebo-Campos, V.; Salvador, M.D.; Fregapane, G. Evolution of Major and Minor Components and Oxidation Indices of Virgin Olive Oil During 21 Months Storage at Room Temperature. Food Chemistry 2007, 100, 36–42.
- Liebler, D.C. Antioxidant Reactions of Carotenoids. Annals of the New York Academy of Sciences 1993, 691, 20–31.
- Kıralan, M.; Bayrak, A. Oxidative and Antiradical Stabilities of Two Important Virgin Olive Oils from Ayvalik And Memecik Olive Cultivars in Turkey. International Journal of Food Properties 2013, 16, 649–657.