Abstract
The freeze-thaw stability of oil-in-water emulsions prepared with unheated and heated aqueous dispersions of fresh and stored soy protein isolates was evaluated in the absence and presence of glucose or sorbitol (0.75–15.0% w/w). Sample aging had a negative impact of freeze-thaw stability. The cryoprotectant addition enhanced the freeze-thaw stability, but at low concentrations emulsions prepared with unheated soy protein isolates showed better response to freeze-thawing. Nevertheless, at the highest cryoprotectant concentration, a total stabilization was evidenced for all emulsions. The results of this article indicated that the cryoprotectants act on proteins at interfacial level.
INTRODUCTION
Soy protein isolates (SPI) are widely used as ingredients in food products and contain two major proteins, glycinin (11S fraction) and β-conglycinin (7S fraction). These protein samples are generally obtained from isoelectric precipitation by acidifying (pH 4.5–4.8) an aqueous extract of defatted soy flour (DSF) and further solubilization and neutralization of precipitate. Indeed, in the laboratory it is possible to obtain SPI of high protein solubility where the storage globulins are in native state.[Citation1]
Heating is one of the most important and frequently used methods for denaturation of food proteins for processing. Unlike SPI samples obtained in the laboratory, the industrial preparation of SPI requires thermal treatments so that the soy globulins can be partially or totally denatured. Thermal treatments have a great impact on the hydration and surface-functional properties of SPI.[Citation2] Thus, thermal treatments carried out on SPI samples can appreciably affect their emulsifying properties. In addition, important structural changes also take place when oil-in-water (o/w) emulsions prepared with native SPI are heated at temperatures where 7S and/or 11S unfold.[Citation3–Citation6]
At present, freezing is a widely used technology that preserves the flavor and nutritional properties of foods, and minimizes undesirable deterioration processes such as microbial growth.[Citation7] For o/w emulsions, freezing implies the removal of heat, accompanied by phase transitions both in the oil and the aqueous phase.[Citation8] The stability of emulsions to freeze-thawing depends on a variety of different physicochemical mechanisms and the occurrence of phase transitions is associated with the emulsion composition (oil type and concentration, emulsifier type and concentration, aqueous sugars, polyols, and aqueous salts) and storage conditions (cooling rate, subzero storage temperature, and storage time).[Citation9–Citation11] When emulsion droplets are trapped and concentrated between growing ice crystals, they will remain stable only as long as the interfacial film remains intact. Although the interfacial membranes stabilized by proteins are relatively resistant to environmental stresses, these emulsifiers usually exert a limited protection during frozen storage. Therefore, the addition of cryoprotectants is often necessary.[Citation8–Citation13] The cryoprotective effect of sugars and polyols on proteins in food emulsions has been reported previously.[Citation11–Citation13] Notwithstanding this, the environmental stresses induced by freeze-thawing, and the capacity of different ingredient combination to resist them is still poorly understood. Hence, for o/w emulsions prepared with proteins as the sole emulsifier, different trends were observed in previous works.[Citation10]
In a previous article,[Citation14] the effect of protein concentration on freeze-thaw stability of model o/w emulsions prepared with native and denatured SPI in the absence of cryoprotectant was evaluated. However, for emulsions subjected to freeze-thawing, the influence of sample aging has been poorly assessed. The purpose of this study was to evaluate the impact of sample aging of laboratory-made SPI on the freeze-thaw stability of model o/w emulsions in the presence of glucose or sorbitol at various concentrations. Aqueous dispersions of fresh and stored SPI also were previously heated to additionally analyze the effect of thermal denaturation on stability of emulsions to freeze-thawing.
Materials and methods
Materials
DSF was provided by Solae Latin America (Barueri, Brazil). The mean proximate composition (% w/w in dry basis, as given by the producer) was: crude protein (N×6.25), 56.0; ash, 7.0; lipids, 3.5; dietary fiber, 14.0. Refined sunflower oil was purchased in a local supermarket. 1-anilino-8-naphtalene sulfonate, ammonium salt (ANS) and bovine serum albumin were purchased from Sigma Co (MO, USA). Sudan III liposoluble dye (C22H16N4O, C.I. 26,100) was purchased from Chroma Gesselschaft (Schmidt GmbH & Co.; Germany). Sorbitol and anhydrous glucose were purchased from Biopack (Buenos Aires, Argentina). Distilled water was always used, and all other chemical were analytical grade reagents.
Preparation of SPI
SPI was obtained from a fiber-free DSF aqueous extract at pH 8.0 by precipitation of storage soy proteins at pH 4.5, separation of pellet by centrifugation, solubilization, and neutralization of precipitate, freeze-drying, and grinding. The detailed experimental procedure was described elsewhere.[Citation1] SPI was stored as a freeze-dried powder at 4°C and was rapidly utilized to avoid the sample aging (fresh sample, SPIF). SPIF sample was stored at 4°C for 2 years, giving the sample SPIS1. Moreover, the sample SPIS2 was prepared from a stored (1 year, 4ºC) DSF, following the same experimental procedure and then was stored at 4ºC for 2 years. The crude protein content (N×6.25, in dry basis) of freeze-dried SPI powder samples was 92.2 ± 1.2% w/w. The cold storage was carried out by placing 50 g of sample (DSF or SPI) in cylindrical vials of polyvinyl chloride (diameter = 60 mm), which were completely filled before sealing.
Preparation of Protein Aqueous Dispersions
SPI aqueous dispersions (2.0% protein w/w) were prepared by dissolving the powders in sodium phosphate buffer 10 mM, pH 7.0 at room temperature under magnetic stirring for at least 3 h (u-SPIF, u-SPIS1 and u-SPIS2). Sodium azide (0.03% w/w) was added to avoid the microbial spoilage. Thermal denaturation of SPI aqueous dispersions was carried out in sealed flasks at 90.0 ± 1.0ºC under mild stirring. Sample temperature increased from 25 to 90°C (15 min) and was kept at final temperature for 5 min; cooling was performed to room temperature (~25ºC) in a water–ice bath. The aqueous dispersions of heated SPI samples (h-SPIF, h-SPIS1, and h-SPIS2) samples were immediately used after their preparation.
Preparation of O/W Emulsions
Coarse emulsions were prepared by mixing refined sunflower oil with SPI aqueous dispersions (oil mass fraction, ϕm = 0.25) in a high-speed blender (Ultraturrax T-25, S25-20NK-19G dispersing tool; IKA Labortechnik; Staufen, Germany) at 20,000 rpm for 60 s. Pre-emulsions were then passed three times through a twin-stage valve high pressure homogenizer (Panda 2K, GEA Niro Soavi; Parma, Italy) at 40 and 4 MPa in the first and second valves, respectively. Glucose or sorbitol addition (0.75, 2.25, 3.75, 7.5, and 15.0% w/w in emulsion, corresponding to 1.0, 3.0, 5.0, 10.0, and 20.0% w/w in the aqueous phase, respectively), was made after homogenization step. Emulsions without cryoprotectant addition were used as control samples.
Freeze-Thaw Treatment
Emulsion samples or aqueous dispersions (20 g) were transferred to vertical plastic containers (internal diameter = 30 mm with plastic lids) and were isothermally stored in still air for 24 h at—18 ± 2ºC. During storage, the freezer temperature periodically varied from –18 to –20ºC and the variation period was approximately 50 min. The temperature of samples before freezing experiments was set at 20 ± 1ºC. After storage, frozen samples were thawed into a water bath at 20 ± 1ºC, and kept at this temperature before further characterization analyses.
Characterization of Protein Aqueous Dispersions
Protein solubility
Protein solubility of SPI aqueous dispersions (2.0% protein w/w, sodium phosphate buffer 10 mM, pH 7.0) before and after heating, was determined by following the experimental procedure of Mitidieri and Wagner.[Citation4] Dispersions were centrifuged for 30 min at 15,000 × g (Beckman Coulter GS-15R, Fullerton; USA). Appropriate dilutions of initial dispersions (before centrifugation step) and their supernatants were carried out and the protein concentration was determined by the method of Markwell et al.,[Citation15] using bovine serum albumin as standard. Protein solubility index (PSI) was determined from:
CP,S is the protein concentration (% w/w) in the supernatant and CP,I is the protein concentration (% w/w) in the initial dispersion before centrifugation.
Aromatic surface hydrophobicity
Aromatic surface hydrophobicity (H0) was determined by fluorescence using the ANS probe, according to the experimental procedure reported by Mitidieri and Wagner.[Citation4] Initial and freeze-thawed SPI aqueous dispersions (2.0% protein w/w) were prepared in sodium phosphate buffer 10 mM, pH 7.0 with or without glucose or sorbitol (3.0 and 20.0% w/w), stirred for 2 h at 20°C and centrifuged at 15,000 × g for 20 min. Protein concentration in the supernatants was determined by the method of Markwell et al.[Citation15] Each supernatant was serially diluted with the same buffer to obtain protein concentrations ranging from 0.05 to 0.0005% w/w, then 40 mL of ANS (8.0 mM in sodium phosphate buffer 100 mM pH 7.0) were added to 3 mL of each diluted sample. Fluorescence intensity (FI) was measured at 365 nm (excitation wavelength) and 484 nm (emission wavelength) using Perkin-Elmer 2000 spectrofluorometer (Perkin Elmer, Waltham, MA, USA). The FI reading was calibrated by adjusting it to a value of 80 (1×) with 15 mL ANS in 3 ml methyl alcohol (high-performance liquid chromatography [HPLC] grade). The initial slope of the FI versus protein concentration plot was used as an index of surface aromatic hydrophobicity (H0).
Characterization of O/W Emulsions
Particle size distribution (PSD)
The PSD of emulsions was determined by laser diffraction using a Malvern Mastersizer 2000E analyzer (Malvern Instruments Ltd., Worcestershire; UK). Optical parameters were: relative refractive index, 1.10; adsorption coefficient: 0.001.[Citation14] Two aliquots of each emulsion were diluted separately with 10 mM sodium phosphate buffer pH 7.0 without and with 1.0% w/v sodium dodecyl sulfate (SDS). These diluted aliquots were then dispersed in tap water at 2000 rpm (Hydro 2000MU dispersion unit) and PSD was finally obtained and expressed as volume frequency. From PSD, De Brouckere (volume-weighted, D4,3) mean diameters were obtained. Flocculation index (FI%) was calculated both for unfrozen and freeze-thawed emulsions:
D4,3 and D4,3 SDS are the volume-weighted diameters, measured without and with of SDS, respectively. Only the flocs stable to measurement conditions (dilution and stirring) are evaluated so that FI% parameter is an apparent value.[Citation14] Moreover, coalescence index (CI%) was calculated from:
D4,3 SDS, F-T and D4,3 SDS, unf are the D4,3 values of freeze-thawed and unfrozen, emulsions, respectively. Both parameters were obtained from PSD in the presence of SDS (1.0% w/v). [Citation14]
Free oil (FO) determination
The dye-dilution spectrophotometric technique was utilized to quantitatively determine the amount of FO present in the emulsions before or after freeze-thawing. The experimental procedure of Palanuwech et al.[Citation16] was followed with some modifications and was described in detail elsewhere.[Citation14] After mixing with emulsions, the Sudan III dye solution (0.0015 g/100 g sunflower oil) is diluted only if they contain non emulsified oil and hence, the absorbance of dye solution decreases. FO was calculated from:
Mo is the mass (g) of added dye solution, Me is the mass (g) of emulsion, ϕm is the oil mass fraction and A = Ab × Aa–Citation1 is the ratio of the measured absorbances of the dye solution before (Ab) and after (Aa) mixing. The calibration, using emulsions with known amount of FO on top, were performed according to previous works. [Citation16]
Optical microscopy
Micrographs were obtained with an optical microscope operating at 400× magnification and using an adapted digital camera (Canon Power Shot A570 IS; Canon Inc., Malaysia) at 4× optical zoom.
Determination of freezable water (FW)
The amount of FW (% w/w) was determined by differential scanning calorimetry (DSC, Q200 calorimeter, TA Instruments, LLC Waters; USA). Unfrozen emulsions (4–6 mg), without and with cryoprotectant, were placed in aluminum pans and rapidly transferred to calorimeter alongside an empty reference pan. Samples were kept at 25°C for 5 min, then cooled down to –30°C at 5°C/min, kept at –30°C for 5 min and finally heated up at the same rate to 25°C. The enthalpy was measured for a known mass of emulsion and for a known mass of pure water. For each emulsion, FW was calculated as FW = (ΔHE /ΔHW) × 100 where ΔHE and ΔHW were the enthalpy changes per unit of mass of water for emulsions and pure water, respectively.[Citation13]
Statistical Analysis
For each experiment, at least two independent replicates were measured and the results were expressed as mean ± standard deviation. The statistical analysis was performed by analysis of variance (ANOVA) and test of least significant differences (LSD) using the statistical program Statgraphics Plus V5.1 (Statgraphics Corporation; USA, 2000). Significance was considered at p < 0.05.
Results and discussion
Characterization of Aqueous Dispersions
PSI values of SPI aqueous dispersions are shown in . For u-SPIS1 sample it can be observed that the sample aging promoted the protein aggregation, which reflects in a significant decrease of PSI (p < 0.05). Besides, the previous storage (4°C, 1 year) of DSF induces an additional loss of PSI (u-SPIS2) attributed mainly to increase of protein aggregation, protein denaturation degree and mineral content. These results are in agreement with those previously reported by Sobral et al.[Citation17] It was also evident that the thermal treatment (90ºC, 5 min) of all SPI aqueous dispersions induced a PSI increase (p < 0.05). According to Mitidieri and Wagner[Citation4] this heating condition ensures a total denaturation of glycinin and β-conglycinin. Thermal treatment at low protein concentration also induces partial dissociation of storage globulins and the formation of soluble aggregates, as was also observed in a previous work.[Citation14] Moreover, the PSI increase is consistent with the almost total dissociation of insoluble aggregates for h-SPIF and h-SPIS1 samples. The lower PSI for sample h-SPIS2 would be attributed to the existence of stable insoluble aggregates in unheated aqueous dispersions, which are only partially dissociated after heating. Indeed, these aggregates were formed during the chilled long storage of DSF.
Table 1 Protein solubility index (PSI) of unheated (u) and heated (h) aqueous dispersions (2.0% protein w/w in sodium phosphate buffer 10 mM, pH 7.0) prepared with fresh (SPIF) and stored (SPIS1 and SPIS2) soy protein isolates
Characterization of Initial Emulsions
The characterization of initial, unfrozen emulsions prepared with fresh and stored SPI samples was performed. In the absence of SDS, for those prepared with u-SPIF and u-SPIS1, PSD were monomodal, whereas in the presence of surfactant, the peak modes were shifted toward lower particle sizes ( and ). Conversely, a clear bimodal behavior was observed for u-SPIS2 emulsion () without SDS. In the presence of the surfactant, the disappearance of peak in the range 2–20 μm and a concomitant increase of particle population at particle sizes lower than 1 μm were evidenced. These results were consistent with the presence of stable flocs in the measurement conditions.[Citation13,Citation14] Undoubtedly, droplet aggregation was more pronounced for u-SPIS2 emulsions (). In the absence of SDS, a unique and broad peak was observed for emulsions prepared with h-SPIF and h-SPIS2. In contrast, for h-SPIS1 emulsions, a large span of particle sizes (ranging from 0.1 to 10 µm) was observed, presumably as consequence of the overlapping of two particle populations. In the presence of SDS, all PSD were monomodal with peak modes lower than 1 µm; for h-SPIF emulsions a single particle population in the range 0.2–3 μm was evidenced, whereas for those prepared with h-SPIS1 and h-SPIS2, PSD was extended up to 4 and 5 µm, respectively ().
FIGURE 1 Particle size distributions of unfrozen o/w emulsions prepared with fresh (a: SPIF) and stored (b: SPIS1; c: SPIS2) soy protein samples.
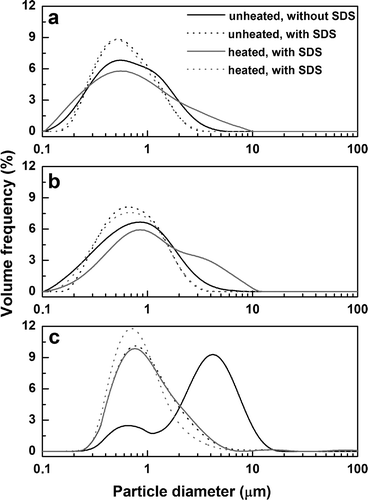
From PSD, De Brouckere (D4,3) mean values were obtained (). In the absence of SDS, for SPIF and SPIS1 emulsions, significant higher D4,3 values were observed respect to those prepared with heated aqueous dispersions (p < 0.05). However, the opposite trend was observed for SPIS2 emulsions. When the measurements were performed with SDS, only the effect of storage of SPI samples was evident: heated and unheated samples showed similar D4,3 values (p > 0.05). However, this parameter increased in the order (SPIF < SPIS1 < SPIS2) whatever the previous heating of dispersions (p < 0.05). According to the differences observed in D4,3 values without and with SDS, FI% was always higher for h-SPIF and h-SPIS1 emulsions (). In contrast, for h-SPIS2 emulsions, a significant lower FI% value respect to that of u-SPIS2 sample was effectively obtained (p < 0.05).
Table 2 De Brouckere (D4,3, volume-weighted) moment mean diameters and flocculation index (FI%) of o/w emulsions prepared with unheated (u) and heated (h) aqueous dispersions of fresh (SPIF) and stored (SPIS1, SPIS2) soy protein isolates. D4,3 values were obtained from particle size distributions measured in the absence (–) and presence (+) of SDS
According to previous works,[Citation3,Citation14,Citation18] emulsions prepared with thermally denatured SPI samples exhibited higher values of adsorbed protein on oil droplets, mainly due to the interfacial adsorption of soluble aggregates. Although there is sufficient emulsifier to cover all of the droplet surfaces,[Citation14] the slow conformational changes of soluble aggregates of h-SPIF and h-SPIS1 at the oil/water interface can occur so that emulsions are flocculated by a bridging mechanism (). Conversely, the lower FI% values for u-SPIF and u-SPIS1 emulsions can be attributed to the absence of these soluble aggregates and the presence of partially dissociated storage globulins, which can better adsorb at the oil/water interface. Interestingly, an opposite tendency was observed for SPIS2 emulsions, where D4,3 and FI% values were always higher for those prepared with heated sample (p < 0.05, ). The fairly low PSI of u-SPIS2 aqueous dispersion, as a consequence of the presence of insoluble aggregates of poor interfacial activity, would explain this result. In the same way, the enhanced emulsifying behavior of h-SPIS2 sample would be attributed to the partial dissociation of insoluble aggregates, which reflects in a significant increase of PSI ().
Characterization of Freeze-Thawed Emulsions
Freeze-thaw stability of o/w emulsions in the absence of cryoprotectants
First, the freeze-thaw stability of o/w emulsions in the absence of cryoprotectant was evaluated. After freeze-thaw treatment, all emulsions exhibited gravitational separation, with the formation of a droplet-rich creamy layer and a droplet-depleted turbid layer, as was also observed in a previous article.[Citation14] According to and , emulsions prepared with u-SPIF were destabilized mainly by coalescence, although the oil phase remained in an emulsified state (FO < 2% w/w, ). CI% values were extremely high (>104) as a consequence to high D4,3 values for freeze-thawed emulsions in the presence of SDS. At the same time, these emulsions exhibited relatively low FI% values (). Undoubtedly, the flocs formed during frozen storage did not resist the stress imposed by the formation of ice.[Citation14] Similar behavior was observed for u-SPIS1 emulsions, with a increase of D4,3 values and a low, but significantly higher value of FO (p < 0.05). Upon freeze-thawing, the cold aggregation of native soy globulins at the oil-water interface promoted the disruption and collapse of interfacial film.[Citation14] Conversely, o/w emulsions prepared with h-SPIF and h-SPIS1 samples were destabilized mainly by flocculation, according to noticeable high FI% values. The formation of flocs for h-SPIF emulsion after freeze-thawing is clearly evidenced in and . The sample aging enhanced the destabilization through an increase of D4,3, CI% and FI% values (). Presumably, the presence of soluble aggregates of denatured globulins at the oil/water interface ensures a short-range steric repulsion, which avoids the extensive coalescence during frozen storage but flocculation cannot be controlled.
Table 3 De Brouckere (D4,3, volume-weighted) moment mean values, flocculation index (FI%), coalescence index (CI%) and the amount of free oil (FO) for freeze-thawed o/w emulsions prepared with unheated (u) and heated (h) aqueous dispersions of fresh (SPIF) and stored (SPIS1, SPIS2) soy protein isolates. D4,3 values were obtained from particle size distributions measured in the absence (–) and presence (+) of SDS
FIGURE 2 Microstructure of o/w emulsions prepared with unheated (u) and heated (h) fresh soy protein isolates (SPIF) before and after freeze-thawing (F-T) in the absence or presence of sorbitol (S) at different concentrations. For u-SPIF emulsions: a: unfrozen, b: F-T without cryoprotectant, c: F-T with S (0.75% w/w), d: F-T with S (15.0% w/w); for h-SPIF emulsions, e: unfrozen, f: F-T without cryoprotectant, g: F-T with S (0.75% w/w), h: F-T with S (15.0% w/w). Bar = 10 μm.
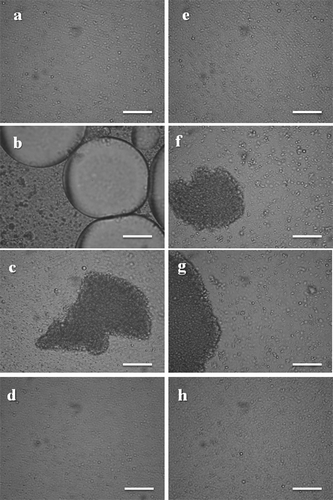
It is worth noting that, for u-SPIS2 and h-SPIS2 emulsions, FO was visually observed on the top of the container, in agreement with noticeable high FO values (>30% w/w, ). Unlike the SPIF and SPIS1 samples, previous heating of u-SPIS2 aqueous dispersions did not improve the response of emulsions to freeze-thawing. Evidently, the previous chilled storage of soy flour has an important influence on the structural characteristics of adsorbed proteins at the oil/water interface, which reflects as a decrease of stability of emulsions to freeze-thawing. For these highly destabilized emulsions, the comparative analysis of D4,3, FI% and CI% values respect to those of other samples should be performed with caution. For example, the lower CI% value of u-SPIS2 emulsions respect to those of u-SPIF and u-SPIS1 was not consistent with a higher stability to freeze-thawing (). A comparative analysis between CI% parameters would be valid only if oil phase remains almost totally in an emulsified state (SPIF and SPIS1 emulsions).
Influence of cryoprotectant addition on freeze-thaw stability of o/w emulsions
In this section, the effect of glucose or sorbitol addition (0.75–15.0% w/w in the emulsion) on the freeze-thaw stability of o/w emulsions was assessed. It is worth noting that the glucose or sorbitol addition at different concentrations did not alter the PSD of all unfrozen emulsions, due to these cryoprotectants were added after homogenization (data not shown). For SPIF and SPIS2 emulsions, both cryoprotectants showed similar behavior after freeze-thawing. Hence, D4,3 values and destabilization parameters were presented only in the presence of sorbitol. For fresh SPI samples (u-SPIF and h-SPIF), D4,3 values measured without and with SDS are shown in . In the presence of SDS, D4,3 values for freeze-thawed u-SPIF and h-SPIF emulsions were similar to those of unfrozen emulsions. This result is consistent with high coalescence stability after freeze-thawing. Indeed, no oil was extracted from these emulsions by dye-dilution technique (FO ≈ 0). Moreover, for freeze-thawed u-SPIF and h-SPIF emulsions, in the absence of SDS, D4,3 values progressively decreased with increasing sorbitol concentration. This fact is in agreement with the inhibition of the formation of new flocs at high cryoprotectant concentration. The presence of flocs were effectively observed at the lowest cryoprotectant concentration (0.75% w/w) for both emulsions ( and ). Nevertheless, it can be observed that in the range 0.75–3.75% w/w sorbitol, D4,3 values without SDS and FI% values were noticeable higher for h-SPIF emulsions respect to those prepared with u-SPIF sample. Conversely, at the highest cryoprotectant concentration, both freeze-thawed u-SPIF and h-SPIF emulsions were totally stabilized, showing D4,3 values similar to those of unfrozen emulsions (, , and ).
FIGURE 3 De Brouckere (D4,3, volume-weighted) moment mean diameters of freeze-thawed o/w emulsions prepared from unheated a: and heated b: fresh soy protein isolates (SPIF) in the presence of sorbitol (S) at different concentrations (0.75-15.0% w/w in the emulsion). Values are mean of two (n = 2) independent replicates and error bars indicate standard deviation. For comparative purposes, D4,3 values of initial, unfrozen emulsions were also included. For u-SPIF or h-SPIF emulsions measured without SDS, mean values with different lowercase letters indicate significant differences between samples (p < 0.05). For u-SPIF or h-SPIF emulsions measured with SDS, mean values with different uppercase letters indicate significant differences between samples (p < 0.05).
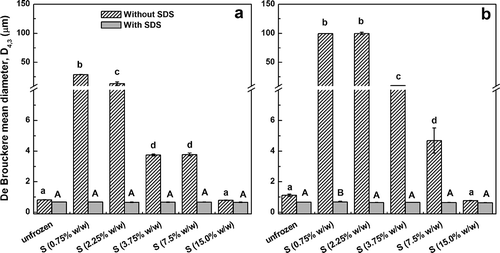
The effect of sample aging was assessed through a comparative analysis between CI% and FI% parameters for unheated and heated SPIF and SPIS1 emulsions (). The sample aging had a negative influence on freeze-thaw stability. For u-SPIF and h-SPIF emulsions, coalescence was totally inhibited; only at the lowest cryoprotectant concentration (0.75% w/w), low CI% values were effectively observed. It is worth noting that, throughout the entire range of sorbitol concentration, CI% values of h-SPIS emulsions were higher respect to those of u-SPIS. Coalescence was not completely inhibited, although CI% values were fairly low at the highest cryoprotectant concentration (15% w/w, ). With regard to FI% values, the tendency was similar for fresh and stored SPI emulsions: the formation of new flocs in the range 0.75–7.5% w/w was not avoided. Besides, FI% values were always higher for emulsions prepared with heated SPI samples and this parameter decreased with increasing sorbitol concentration ().
Table 4 Flocculation index (FI%) and coalescence index (CI%) values of freeze-thawed o/w emulsions prepared with unheated and heated fresh and stored (SPIF and SPIS1, respectively) soy protein aqueous dispersions with addition of sorbitol as cryoprotectant at different concentrations (0.75–15.0% w/w in the emulsion)
Total denaturation of storage globulins caused by thermal treatment at pH 7.0 of dilute aqueous dispersions led to unfolding of molecules, exposing hydrophobic zones.[Citation19,Citation20] Aromatic surface hydrophobicity (H0) is noticeably increased upon heating, as was observed in for SPIS1 aqueous dispersions in the absence of sorbitol (control). After freeze-thawing, H0 values were similar whatever the heating. As was mentioned above, h-SPISI emulsions exhibited high FI% values but a relatively high stability to coalescence (). For h-SPIS1 sample, its high H0 value would promote the adsorption of soluble aggregates at oil/water interface, due to an increase of hydrophobic interactions. Thus, the coalescence stability is increased although the flocculation cannot be controlled.
FIGURE 4 a: Aromatic surface hydrophobicity (H0) of unheated and heated aqueous dispersions prepared with stored soy protein isolates (SPIS1) before (U) and after freeze-thawing (F-T) in the absence (control) and presence of sorbitol (S, 3.0 and 20.0% w/w); b: Freezable water content (FW% w/w) of o/w emulsions prepared with unheated and heated stored soy protein isolates (SPIS1) in the absence (control) and presence of sorbitol (S) as cryoprotectant (0.75–15.0% w/w in the emulsion). H0 and FW values are the mean of two (n = 2) independent replicates ± standard deviation. For dispersions or emulsions prepared with unheated and heated SPIS1 samples, mean values with different lowercase letters are significantly different (p < 0.05).
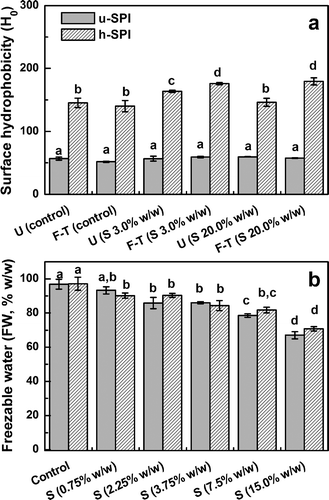
An additional increase of H0 values was observed for h-SPIS1 dispersion with sorbitol addition after freeze-thawing (). According to previous articles,[Citation10,Citation21] the presence of sugars or polyols, increased the attraction between emulsion droplets once the protein molecules had unfolded. Although, both u-SPIS1 and h-SPIS1 emulsions with sorbitol, were more stable to freeze-thawing than those without cryoprotectant ( and ), emulsions prepared with more hydrophobic h-SPIS1 sample exhibit higher FI% and CI% values. Even though the cryoprotectants are added at low concentration, the ability of cold denaturation-aggregation of native soy globulins at the oil/water interface would be inhibited. Thus, interfacial membrane would be less prone to collapse during freeze-thawing. Conversely, the attraction between protein molecules in the presence of cryoprotectant once they have unfolded, would promote the droplet aggregation during frozen storage. At low and high levels of sorbitol, the amount of FW was similar for u-SPIS1 and h-SPIS1 emulsions (). This data is consistent with a similar volume of unfrozen aqueous phase. Hence, the influence of cryoprotectants is exerted at interfacial level, where they affect the conformation of protein at the oil/water interface. At high cryoprotectant concentration (15.0% w/w), the differential effect of cryoprotectants on the stability of emulsions prepared with unheated and heated SPI samples, would be masked by the significant decrease in the FW content (). The increase of viscosity and the confinement of droplets in a higher volume of unfrozen aqueous phase enhance the stability to freeze-thawing.
Interestingly, the differential effect of cryoprotectant at low concentration (0.75–3.75% w/w) was dramatically increased for emulsions prepared with SPIS2 samples (). In the absence of cryoprotectant, these emulsions were highly unstable with an extensive oiling off (). With the addition of glucose or sorbitol almost no oil was extracted after freeze-thawing (FO ≈ 0), but h-SPIS2 emulsions exhibited noticeable higher CI% values than those of u-SPIS2 samples. Unlike the other freeze-thawed emulsions (SPIF and SPIS1), glucose seems to have a better response than sorbitol for h-SPIS2 emulsions (). Conversely, the polyol was more effective as cryoprotectant for those prepared with u-SPIS2. It is evident that the explanation of this result requires a more rigorous approach related with the interaction between individual sugars or polyols and adsorbed proteins on oil droplets. Nevertheless, this fact is clearly consistent with the influence that cryoprotectants exert on proteins at interfacial level during freeze-thawing.
FIGURE 5 Coalescence index (CI%) values of freeze-thawed o/w emulsions prepared with unheated and heated stored soy protein isolates (SPIS2) in the presence of sorbitol a: and glucose b: at different concentrations (0.75–3.75% w/w in the emulsion). CI% values are the mean of two independent (n = 2) replicates ± standard deviation.
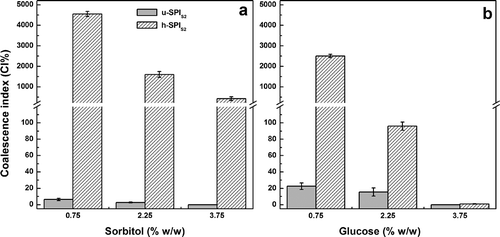
Conclusions
The freeze-thaw stability of o/w emulsions depend on a complex variety of physicochemical processes that can occur in the aqueous and oil phase. In this article, it is clear that the previous chilled storage of soy flour and SPI, can noticeably influence the protein aggregation in unheated and heated aqueous dispersions and hence, the freeze-thaw stability of emulsions both in the absence and presence of cryoprotectant. Sample aging, mainly in those emulsions prepared with SPI where the soy flour was previously stored, exerted a negative effect on freeze-thaw stability, which mainly reflects in high CI% values and extensive oiling off when no cryoprotectant is present in the aqueous phase. At low cryoprotectant concentration, a differential effect of sorbitol on stability of emulsions prepared with unheated and heated samples was effectively observed. Moreover, freeze-thaw stability was increased with increasing glucose or sorbitol concentration and the differential effect disappeared at high cryoprotectant concentration. In this case, the volume of unfrozen aqueous phase increases, so the oil droplets can better reorganize to resist the stress imposed by the expanding ice. The results of this paper can be interpreted in terms of the effect that the cryoprotectants exert on proteins at interfacial level. In studying the freeze-thaw stability of o/w emulsions prepared with proteins as the sole emulsifier, the effect of sample aging should be always empathized. In the same way, during the preparation of SPI, it is expected that all experimental modifications that affect the protein aggregation degree have a noticeable influence on stability of o/w emulsions to freeze-thawing.
Acknowledgment
Authors wish to thank the CINDEFI (Universidad Nacional de La Plata) by the availability of Perkin-Elmer spectrofluorometer.
Funding
The authors are grateful for the financial support of CONICET (PIP 2012-2014 Nº 11220110100398) and Universidad Nacional de Quilmes (53/1007 I+D grant).
Additional information
Funding
References
- Sorgentini, D.A.; Wagner, J.R. Comparative Study of Structural Characteristics and Thermal Behavior of Whey and Isolate Soybean Protein. Journal of Food Biochemistry 1999, 23, 489–507.
- Sorgentini, D.A.; Añón, M.C.; Wagner, J.R. Enhancement of Hydration and Surface Properties of Soy Isolates by Thermal and Acid Treatments. In Food Emulsions and Dispersions; Anton, M.; Ed.; Research Signpost: Kerala, India, 2002; 29–39.
- Keerati-u-rai, M.; Corredig, M. Heat-Induced Changes in Oil-in-Water Emulsions Stabilized with Soy Protein Isolates. Food Hydrocolloids 2009, 23, 2141–2148.
- Mitidieri, F.E.; Wagner, J.R. Coalescence of O/W Emulsions Stabilized by Whey and Isolated Soybean Proteins. Influence of Thermal Denaturation, Salt Addition, and Competitive Interfacial Adsorption. Food Research International 2002, 35, 547–557.
- Palazolo, G.G.; Sorgentini, D.A.; Wagner, J.R. Emulsifying Properties and Surface Behavior of Native and Denatured Whey Soy Proteins in Comparison with Other Proteins. Creaming Stability of O/W Emulsions. Journal of the American Oil Chemists’ Society 2004, 81, 625–632.
- Perrechil, F.A.; Ramos, V.A.; Cunha, R.L. Synergistic Functionality of Soybean 7S and 11S Fractions in Oil-in-Water Emulsions. Effect of Protein Heat Treatment. International Journal of Food Properties 2015, 18, 2593–2602.
- Xiong, Y.L. Protein Denaturation and Functionality Losses. In Quality of Frozen Foods; Erickson, M.C.; Hung, Y.C.; Eds.; Chapman & Hall: New York, NY, 1997; 111–139.
- Cornacchia, L.; Roos, Y.H. Lipid and Water Crystallization in Protein Stabilised Emulsions. Food Hydrocolloids 2011, 25, 1726–1736.
- Cramp, G.L.; Docking, A.M.; Ghosh, S.; Coupland, J.N. On the stability of Oil-in-Water Emulsions to Freezing. Food Hydrocolloids 2004, 18, 899–905.
- Ghosh, S.; Coupland, J.N. Factors Affecting the Freeze-Thaw Stability of Emulsions. Food Hydrocolloids 2008, 22, 105–111.
- McClements, D.J. Protein-Stabilized Emulsions. Current Opinion in Colloid and Interface Science 2004, 9, 305–313.
- Ghosh, S.; Cramp, G.; Coupland, J.N. Effect of Aqueous Composition on the Freeze-Thaw Stability of Emulsions. Colloids and Surfaces A: Physicochemical and Engineering Aspects 2006, 272, 82–88.
- Thanasukarn, P.; Pongsawatmanit, R.; McClements, D.J. Impact of Fat and Water Crystallization on the Stability of Hydrogenated Palm Oil-in-Water Emulsions Stabilized by Whey Protein Isolate. Colloids and Surfaces A: Physicochemical and Engineering Aspects 2004, 246, 49–59.
- Palazolo, G.G.; Sobral, P.A.; Wagner, J.R. Freeze-Thaw Stability of Oil-in-Water Emulsions Prepared with Native and Thermally Denatured Soybean Isolates. Food Hydrocolloids 2011, 25, 398–409.
- Markwell, M.A.K.; Haas, S.; Bieber, L.L.; Tolbert, N.E. A Modification of the Lowry Procedure to Simplify Protein Determination in Membrane and Lipoprotein Samples. Analytical Biochemistry 1978, 87, 206–210.
- Palanuwech, J.; Poniteni, R.; Roberts, R.F.; Coupland, J.N. A Method to Determine Free Fat in Emulsions. Food Hydrocolloids 2003, 17, 55–62.
- Sobral, P.A.; Palazolo, G.G.; Wagner, J.R. Thermal Behavior of Soy Protein Fractions Depending of Their Preparation Methods, Individual Interactions and Storage Conditions. Journal of Agricultural and Food Chemistry 2010, 58, 10092–10100.
- Li, F.; Kong, X.; Zhang, C.; Hua, Y. Effect of Heat Treatment on the Properties of Soy-Protein Stabilized Emulsions. International Journal of Food Science and Technology 2011, 46, 1554–1560.
- Yamauchi, F.; Yamagishi, T.; Iwabuchi, S. Molecular Understanding of Heat-Induced Phenomena of Soybean Protein. Food Reviews International 1991, 7(3), 283–322.
- He, Z.; Li, W.; Guo, F.; Li, W.; Zeng, M., Chen, L. Foaming Characteristics of Commercial Soy Protein Isolate As Influenced by Heat-Induced Aggregation. International Journal of Food Properties 2015, 18, 1817–1828.
- Kulmizraev, A.; Bryant, C.; McClements, D.J. Influence of Sucrose on the Thermal Denaturation, Gelation, and Emulsion Stabilization of Whey Proteins. Journal of Agricultural and Food Chemistry 2000, 48, 1593–1597.