Abstract
A chemically modified electrode was constructed by incorporating manganese (III) tetraphenyl porphyrine into a carbon paste matrix. The modified electrode was used as a sensitive electrochemical sensor for measuring of riboflavin. The constructed electrode exhibited catalytic properties for the electro-oxidation of riboflavin and lowered the over potential for the oxidation of this compound; consequently, the corresponding peak currents of riboflavin increased significantly. The modified electrode showed a near-Nernstian behavior for electro-oxidation of riboflavin hence, it could be a suitable voltammetric sensor for the fast and easy determination of riboflavin. A linear response in concentration range 1.0 × 10−8 – 1.0 × 10−5 M was obtained with a detection limit of 8.0 × 10−9 M (S /n = 3) for the determination of riboflavin. The electrode showed long-term stability and the standard deviation of the slope obtained after repeated calibration during a period of 3 months was 3.5% (n = 10). The modified electrode was used for differential pulse voltammetric determination of riboflavin in pharmaceutical and food samples.
Introduction
Riboflavin (RF; vitamin B2) is a part of the vitamin B group. It is the major component of the cofactors flavin adenine dinucleotide (FAD) and flavin mononucleotide (FMN), and it is required for a variety of flavoprotein enzyme reactions including activation of other vitamins. Like all the B vitamins, vitamin B2 plays a key role in energy production. RF can be found in certain foods such as milk, meat, eggs, nuts, enriched flour, and green vegetables. RF is frequently used in combination with other members of B vitamins group in B-complex products. The importance of flavins in enzymatic catalysis and biochemical reactions have motivated studies of the electrochemical behavior and determination of flavins.[Citation1] So far several analytical techniques such as Electrogenerated chemiluminesence,[Citation2] ultra high-performance liquid chromatography/liquid chromatography-mass spectrometry (UHPLC/LC–MS),[Citation3] high-performance liquid chromatography (HPLC),[Citation4] fluorescence,[Citation5] and ultraviolet visible (UV-Vis) spectroscopy[Citation6] have been developed for the determination of RF. However, these methods have their own advantages and disadvantages. These methods are usually time consuming, expensive instruments, and involve complicated sample processing and procedures. Due to the high selectivity, sensitivity, and simplicity of electrochemical methods, several electrochemical sensors have been developed for the determination of RF[Citation7–Citation18] and other inorganic, organic, and pharmaceuticals samples.[Citation19–Citation21] Wang and co-workers exploited 32-mer homoadenine ssDNA oligonucleotides and graphene as an effective anchoring block for attachment to the layered molybdenum disulfide and the Au electrode. They electrochemically reduced the molybdenum disulfide binding with the Au electrode and used this sensor for determination of RF with differential pulse voltammetry.[Citation22] Square-wave adsorptive stripping voltammetric method was also used for the determination of RF in oral, syrup, and tablet samples by using an ex situ plated bismuth-film electrode.[Citation23] Surface modification of glassy carbon electrode has been achieved by electropolymerized film of 3-amino-5-mercapto-1, 2, 4-triazole. The modified glassy carbon electrode has been used for the simultaneous determination of RF, ascorbic acid, and folic acid at physiological pH by electrochemical method.[Citation15] Nezamzadeh and Pouladsaz investigated the immobilization of Co2+-Y zeolite into carbon paste and studied its electrocatalytic activity for oxidation of RF.[Citation24] A critical assessment of voltammetric methods for determination of RF is presented in . All of these electrochemical sensors and methods have their own advantages and disadvantages. Some of these methods have the narrow linear dynamic range,[Citation7,Citation14,Citation15,Citation23,Citation24] or require tedious and complex electrode pretreatment or construction.[Citation12,Citation18] The toxicity of mercury has caused a decline in the application of some methods.[Citation13,Citation14] in routine laboratories. The calibration curve of some methods shows two or three different slopes[Citation17,Citation23] which creates practical difficulties in measurement of RF. In the continuing development and introduction of new electrochemical sensors, we describe incorporation of manganese (III) tetraphenyl porphyrine (MnTPP) as a modifier into carbon paste matrix to make a sensitive and selective sensor for the determination of RF. The electrochemical behavior of RF on the surface of modified carbon paste electrode was studied by using cyclic voltammetry and differential pulse voltammetry. The catalytic behavior of MnTPP allowed the development of a sensitive electrochemical sensor for the determination of RF in different matrices. The electrode does not need preconcentration required for carbon ionic liquid electrode,[Citation7] or pretreatment which is performed for pencil graphite electrode.[Citation12] Long-term stability, excellent catalytic activity, high reproducibility, and simplicity of preparation are some of the remarkable properties of the developed sensor. The electrode could be readily applied for the determination of RF in real samples such as milk, beverages, and pharmaceutical products.
TABLE 1 List of some of voltammetric methods have been reported for determination of RF
Materials and method
Reagents
Vitamins C, B5, B2, B3, B6, B1, B12, B9, and Nujol were obtained from Merck (Darmstadt, Germany). Graphite powder (mesh size < 100 µm) was supplied by Fluka. All chemicals used were of reagent grade. Stock solution (1 mM) of RF was prepared before using and being protected against light. RF is only slightly soluble in water but it is soluble in 0.1 M sodium hydroxide. First, RF was dissolved in the minimum amount of 0.1 M sodium hydroxide and then distilled deionized water was added to bring the volume up to the final 10 mL. Distilled deionized water was obtained by using a Millipore water system and was used in all tests. Tetraphenylporphyrin was prepared and metallated according to the reported procedures.[Citation25,Citation26]
Apparatus
Electrochemical experiments were performed with a potentiostat-galvanostat (Computrace 797 VA) electrochemical system in conjunction with a 663VA Stand Metrohm (Swiss) electrochemical cell. A three-electrode cell was employed incorporating carbon paste modified working electrode, KCl-saturated Ag/AgCl reference electrode and platinum counter electrode. Cyclic voltammograms were recorded on the modified and unmodified electrodes from 0−0.7 V. Solutions were deaerated by bubbling nitrogen prior to the experiments and the electrochemical cell was kept under nitrogen atmosphere throughout the experiments.
Preparation of Modified Electrode
To prepare Manganese tetraphenylporphyrin (MnTPP) modified carbon paste electrode (MnTPP/CPE), a 50.0 mg portion of MnTPP was throughly mixed with 450.0 mg graphite powder and then the mixture dispersed in 2.0 mL dimethylformamide (DMF) and homogenized in ultrasonic bath for 20 min. DMF was evaporated at 100°C in an oven overnight. Then, graphite powder consisting of 10% w/w MnTPP was mixed with mineral oil, Nujol, in 30% weight ratio. After thorough hand mixing them in a mortar and pestle to obtain a very fine paste, a portion of the composite mixture was packed into the end of a Pyrex glass tube (ca. 3 mm i.d. and 20 cm long). Electrical contact was established by forcing a copper wire down the glass tube and into the back of the mixture. Unmodified carbon paste electrode (UMCPE) was prepared accordingly without adding MnTPP. The working surface of the electrodes was polished by using a soft polishing tissue to have a shiny surface. Then, the electrodes were rinsed with distilled water.
Preparation and Analysis of Real Samples
A kind of B-complex tablet (B-COMPLEX produced by Amin pharmaceutical Co., Isfahan, Iran) containing vitamins B1 (1.5 mg), B2 (2 mg), B6 (2 mg), and B3 (10 mg); and a kind of multivitamin tablet (produced by Amin pharmaceutical Co., Isfahan, Iran) containing vitamins B1 (1.5 mg), B2 (1.7 mg), B6 (2 mg), B12 (12 μg), B9 (400 μg), and C (60 mg) were obtained from a local drugstore. Nine tablets from three different sheets were weighed, and then ground to a fine powder with a pestle and mortar. Then, the powder was mixed with 50 mL of deionized water and was shaken for 10 min on a shaker. After this stage, the samples were centrifuged for 5 min to remove traces of any undissolved matter, then the clear solution obtained was diluted with acetate buffer solution to the required concentration and differential pulse voltammetry was performed by using standard addition method. Delester (a non-alcoholic beer, Iran Behnoush Co.) was purchased from a local supermarket. Samples were prepared by dilution in acetate buffer (1:5) and the determination was made by standard addition method. Five milliliters of cow milk (Pak, Iran Dairy Industries Co.) was mixed well with 1 mL glacial acetic acid to precipitate out proteins. The solution was then digested on a water bath for 20 min and centrifuged for 10 min at 4000 rpm. The 1 mL of diluted filtrate was mixed with acetate buffer pH 5.0 and the standard addition method was used for determination.
Results and discussion
Voltammetric Studies of Riboflavin
Cyclic voltammograms of 50 µM RF in 0.1 M acetate buffer (pH 5.0) at the surface of unmodified carbon paste electrode (UMCPE, curve B), and carbon paste electrode modified with MnTPP (MnTPP/CPE, curve A) in the potential range of 0.0–0.7 V vs. Ag–AgCl reference electrode have been shown in . It is observed that RF undergoes reversible reaction with the difference in peaks potential (ΔEp) of 36 mV at a scan rate of 100 mVs−1 at the surface of UMCPE with the very small peaks current. This behavior shows that RF undergoes two electron exchange reactions. The remarkable current enhancement in the electrochemical reaction of RF occurs when the electrode is modified with MnTPP. On the other hand, there is no measurable wave for the modified electrode in the potential range studied in plain supporting electrolyte. This behavior, which was observed at several potential scan rates and different pH of supporting electrolyte, clearly demonstrates that the MnTPP acts as electrocatalyst in electrochemical response of RF on the surface of modified electrode. In these conditions, the peak separation of RF on the MnTPP/CPE is 80 mV and the ratio of cathodic to anodic peak current is 1.7, indicating that the reversibility decreases on the surface of modified electrode.
FIGURE 1 Cyclic voltammograms A: MnTPP/CPE in the presence of 50 µM RF; B: UMCPE in the presence of 50 µM RF; and C: MnTPP/CPE in 0.1 M acetate buffer pH 5.0 and scan rate 100 mVs−1.
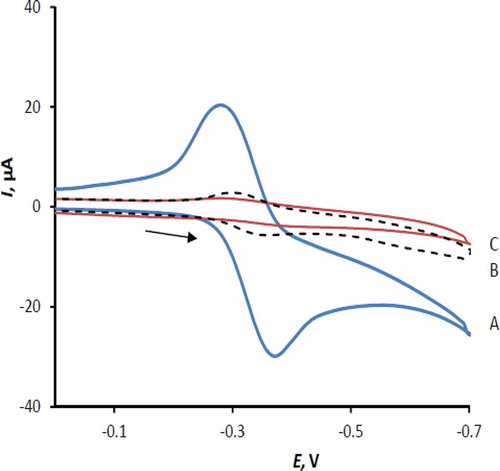
shows the effect of solution pH on the cyclic voltammograms of 50 µM RF in the range of 3.0–7.0. The results show that the ΔIp values were affected by pH and enhance by increasing pH from 3.0 to 5.0. However, at higher pH the ΔIp values decrease extremely and the peaks almost disappear at pH 7.0. Such a behavior can be related to degradation of RF at higher pH values in ambient light.[Citation24] As it can be seen, the peak separation of RF in acetate buffer with pH 3, 4, 5, and 6 are 125, 166, 80, and 90 mV, respectively. Therefore, pH 5 was selected for further study. In addition, the effect of various type of electrolyte such as borate, Briton-Robinson, phosphate and acetate buffers on the peak current and shape were studied (figure not shown). The results show that 0.1 M of acetate buffer is the best supporting electrolyte for determination of RF due to the fact that borate buffer almost make it disappear both cathodic and anodic waves. On the other hand, phosphate and Briton-Robinson buffers decrease the peak current and broaden the peak. Therefore, acetate buffer was selected as a suitable electrolyte for more study.
FIGURE 2 Cyclic voltammograms of 50 µM RF on the surface of MnTPP/CPE at different pH: A: pH 3; B: pH 4; C: pH 5; D: pH 6; and E: pH 7.
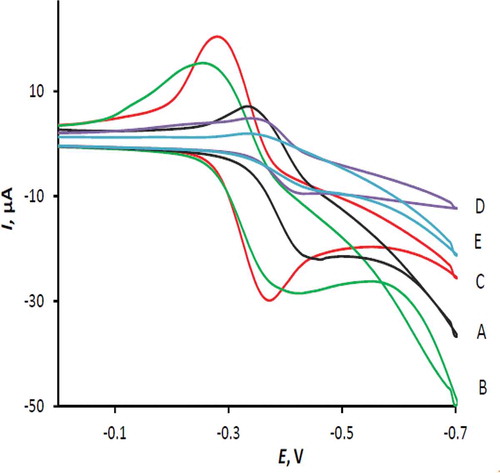
The effect of scan rate on the cyclic voltammetric response of 50 µM RF at the modified electrode surface is shown in . The relation between the peak current, ΔIp, and the square root of scan rate, Ѵ1/2 is linear () for both cathodic and anodic waves in the range of 25–400 mVs–1 with the following equations: ΔIpa = 81.3 ʋ½ – 11.3, R2 = 0.9929; ΔIpc = –135.7 ʋ½ – 5.2, R2 = 0.9909. This behavior, suggests that the reduction and reverse oxidation of RF at the MnTPP/CPE be a diffusion controlled process.[Citation27] On the other hand, the intercepts of the above equations indicate that the electrode processes are not simple. The current function plots (insets of ) illustrate the characteristic shape of EC mechanism for the RF reduction.[Citation28]
FIGURE 3 Cyclic voltammograms of 50 µM RF on the surface of MnTPP/CPE at different scan rates: A: 25; B: 50; C: 100; D: 200; E: 300; and F: 400 mVs−1.
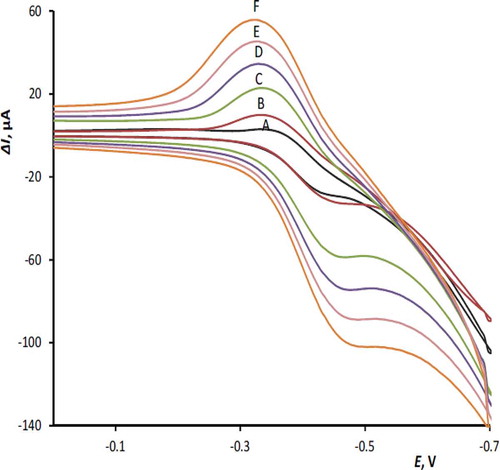
FIGURE 4 The peak currents versus scan rate ranges from 25–400 mVs−1 (derived from ). Inset: The current function plot for reduction of 50 µM RF, pH 5.
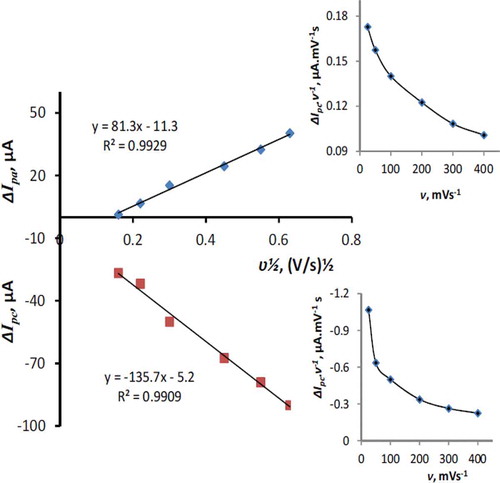
A Tafel plot was drawn from points of the Tafel region of the cyclic voltammogram as it has been shown in , to obtain charge transfer coefficient and information about the rate determination step. According to the cathodic Tafel equation (Eq. 1) the slope of the Tafel plot is equal to.
where I0 is the exchange current, αc stands for the charge transfer coefficient and n represents the number of electrons transferred in the rate determination step. The value of αc equal to 0.41 and 0.34 was obtained for scan rate 25 and 400 mVs–1, respectively, which indicates that the activation free energy curve is not symmetrical in such a semi-reversible oxidation process.
A plot of peak potential vs. log ν was obtained (figure is not shown). The relationship between the reduction peak potentials and logarithm of the scan rates is also linear with the corresponding Eq (2):
By comparing Eq. (2) to the equation obtained by Zhang[Citation29] for an irreversible electrochemical processes:
where αc nc, F, R, and T are charge transfer coefficient, number of electrons involved in the rate-determining step, Faraday constant, gas constant, and absolute temperature, respectively. The value of αc was obtained as 0.32 which is in agreement with that acquired from Tafel equation (0.34).
Differential Pulse Voltammetric Measurements
Differential pulse voltammetry (DPV) was used for calibration curve studies and measurement of RF on the MnTPP/CPE. It was observed from the differential pulse voltammogram that RF on the UMCPE exhibits a well-defined peak at –0.36 V. However, considerable enhancement in the peak current was observed on the modified electrode. Optimum conditions for the DPV response were established by measuring the dependency of peak current on several instrumental parameters, including pulse amplitude, measuring time, pulse duration, and potential scan rate. These parameters were optimized to obtain maximum signal-to-noise ratio using 5 µM RF in acetate buffer pH 5.0 by the one factor at a time method. Optimum values for the investigated parameters were obtained as 100 mV pulse amplitude, 1 ms measuring time, 5 ms pulse duration, and 10 mVs–1 potential scan rate. shows a typical differential pulse voltammograms of 5 µM RF (pH 5.0) at the surface of the modified electrode at the scan rate 10 mVs–1 and different puls amplitude in the range 10–120 mV. After optimization of the parameters, calibration plot, i.e., peak current versus RF concentration, was constructed under the optimal conditions. shows differential pulse voltammograms of different concentration of RF on the surface of MnTPP/CPE. The calibration graph of RF is linear in the concentration range 1.0 × 10−8 – 1.0 × 10−5 M with the following equation (Eq. 4). A detection limit (3Ϭ) of 8.0 × 10−9 M (8.0 nM) was obtained for determination of RF.
Effect of Modifier Percent
Differential pulse voltammograms of RF under optimum conditions were recorded on the surface of the modified electrodes with 5, 10, and 15% (w/w) MnTPP. Calibration curves of RF were examined using the same instrumental parameters for all three electrodes. Following equations were obtained: Y = –7.28X – 8.80, R2 = 0.9963; Y = –13.42X – 4.17, R2 = 0.9958; Y = –10.52X – 5.21, R2 = 0.9943 for the modified electrodes with 5, 10, and 15% (w/w) MnTPP, respectively. The results disclosed that the optimum percent of MnTPP was 10%. Lower and higher amounts of the modifier decrease the sensitivity of the electrode response. Hence, a modified electrode containing 10% MnTPP was successfully applied for the electrocatalytic oxidation of RF.
Repeatability, Reproducibility, and Stability
The stability and repeatability of the sensor response are two important parameters for the evaluation of the viability of a sensor. Over a period of 3 months, the electrode retained 97.8% of its initial peak current response for a concentration of 1.0 µM of RF. This shows the long-term stability of the modified electrode in determination of RF. Repeated voltammograms (n = 8) showed that relative standard deviation for 0.05, 0.1, and 5.0 µM of RF was 3.9, 2.3, and 2.1%, respectively. This indicates the good repeatability of the response of the modified electrode. Reproducibility in electrode construction was evaluated by the determination of RF concentration with three independent electrodes. The relative standard deviation for 1.0 µM of RF on these three electrodes was 3.8% which shows the good reproducibility in preparation of the modified electrode. All results indicated that the modified electrode has good reproducibility and repeatability both in the voltammetric determinations and the preparation procedure with excellent long-term stability.
Interference Study
In order to investigate the selectivity of the proposed method for the determination of RF in real sample matrix, various potentially interference species on the determination of 1.0 µM RF was investigated under optimum conditions. The tolerance limit was defined as the concentration which gave a relative error of ±5.0% in the determination. Some anions, cations, and organic compounds that may exist in real food samples were selected. The results are summarized in . The results show that the adding of some inorganic ions such as Mg2+, Fe3+, Ca2+, Cu2+, K+, and Na+, do not interfere with RF determination. Some organic substances such as 100-fold of glycine, glucose, glutamic acid ascorbic acid, carbonate, and bicarbonate; 10-fold of B1, B6, B12, H, and B9 Vitamins and 6.0 fold of niacin and uric acid do not interfere the determination of 1.0 µM RF.
TABLE 2 Interference study for determination of 1.0 µM RF under the optimized conditions
Analytical Application
In order to study the applicability of the proposed method in a practical analytical situation, the method was used for the determination of RF in real samples such as cow milk, non-alcoholic beer, B-complex, and multivitamin tablets. The standard addition method was utilized for the evaluation of the recovery of the spiked samples at three different levels (0.5 and 1.0 µM). The results of RF determination, summarized in , show an average recovery of 98.0–101.40%, indicating that the constituents of the samples do not significantly interfere with the determination of RF. The accuracy of the method was calculated for B-complex and multi vitamin tablets according to the label of pharmaceutical samples. The results of t-test () show that tcal < ttable and this means the null hypothesis is accepted (with the probability 95% and degree of freedom 3) and there is no difference between true value and test results. On other words, there is no any bias or systematic error for the proposed method. Therefore, the method seems to be promising for the determination of RF in food samples.
TABLE 3 Results of measurement and the recovery analysis of RF in food and pharmacutical samples
Conclusion
In this research, we used manganese tetraphenylporphyrin to construct a highly sensitive sensor for determination of RF based on carbon paste matrix. Remarkable improvement in the electron transfer kinetics of RF reduction was observed on the surface of MnTPP/CPE, by increasing sharpness and the peak current of the cathodic wave. Differential pulse voltammetry using the modified electrode was proposed a very selective and sensitive method with a nanomolar detection limit for the determination of RF. Wide linear dynamic ranges (3 order of magnitude), low detection limit, very good reproducibility and repeatability, high sensitivity, and stability can be presented as the advantages of the prepared sensor. The proposed method was successfully utilized for the determination of RF content in milk, Delester, B-complex, and multivitamin tablets.
FUNDING
The partial support of this survey by Payame Noor, University of Tehran, is acknowledged.
References
- Mateljan, G. The World’s Healthiest Foods/Vitamin B2: 2015. http://www.whfoods.com/genpage.php?tname=nutrient&dbid=93 (accessed May 23, 2015).
- Qi, H.; Cao, Z.; Hou, L. Electrogenerated Chemiluminesence Method for the Determination of Riboflavin at An Ionic Liquid Modified Gold Electrode. Spectrochimica Acta Part A: Molecular and Biomolecular Spectroscopy 2011, 78, 211–215.
- Zand, N.; Chowdhry, B.Z.; Pullen, F.S.; Snowden, M.J.; Tetteh, J. Simultaneous Determination of Riboflavin and Pyridoxine vy UHPLC/LC–MS in UK Commercial Infant Meal Food Products. Food Chemistry 2012, 135, 2743–2749.
- Petteys, B.J.; Frank, E.L. Rapid Determination of Vitamin B2 (Riboflavin) in Plasma by HPLC. Clinica Chimica Acta 2011, 412, 38–43.
- Žiak, L.U.; Májek, P.; Hroboňová, K.; Čacho, F.; Sádecká, J. Simultaneous Determination of Caffeine, Caramel, and Riboflavin in Cola-Type and Energy Drinks by Synchronous Fluorescence Technique Coupled with Partial Least Squares. Food Chemistry 2014, 159, 282–286.
- Koop, J.; Monschein, S.; Pauline Macheroux, E.; Knaus, T.; Macheroux, P. Determination of Free and Bound Riboflavin in Cow’s Milk Using a Novel Flavin-Binding Protein. Food Chemistry 2014, 146, 94–97.
- Safavi, A.; Maleki, N.; Ershadifar, H.; Tajabadi, F. Development of a Sensitive and Selective Riboflavin Sensor Based on Carbon Ionic Liquid Electrode. Analytica Chimica Acta 2010, 674, 176–181.
- Sá, É.S.; da Silva, P.S.; Jost, C.L.; Spinelli, A. Electrochemical Sensor Based on Bismuth-Film Electrode for Voltammetric Studies on Vitamin B2 (Riboflavin). Sensors and Actuators B: Chemical 2015, 209, 423–430.
- Nezamzadeh-Ejhieh, A.; Pouladsaz, P. Voltammetric Determination of Riboflavin Based on Electrocatalytic Oxidation at Zeolite-Modified Carbon Paste Electrodes. Journal of Industrial and Engineering Chemistry 2014, 20, 2146–2152.
- Li, Y.; Hsu, P.-C.; Chen, S.-M. Multi-Functionalized Biosensor At WO3–Tio2 Modified Electrode for Photoelectrocatalysis of Norepinephrine and Riboflavin. Sensors and Actuators B: Chemical 2012, 174, 427–435.
- Hajian, A.; Rafati, A.A.; Afraz, A.; Najafi, M. Electrosynthesis of High-Density Polythiophene Nanotube Arrays and Their Application for Sensing of Riboflavin. Journal of Molecular Liquids 2014, 199, 150–155.
- Ensafi, A.A.; Heydari-Bafrooei, E.; Amini, M. DNA-Functionalized Biosensor for Riboflavin Based Electrochemical Interaction on Pretreated Pencil Graphite Electrode. Biosensors and Bioelectronics 2012, 31, 376–381.
- Bandžuchová, L.; Šelešovská, R.; Navrátil, T.; Chýlková, J.; Novotný, L. Voltammetric Monitoring of Electrochemical Reduction of Riboflavin Using Silver Solid Amalgam Electrodes. Electrochimica Acta 2012, 75, 316–324.
- Baś, B.; Jakubowska, M.; Górski, Ł. Application of Renewable Silver Amalgam Annular Band Electrode to Voltammetric Determination of Vitamins C, B1, and B2. Talanta 2011, 84, 1032–1037.
- Revin, S.B.; John, S.A. Simultaneous Determination of Vitamins B2, B9, and C Using a Heterocyclic Conducting Polymer Modified Electrode. Electrochimica Acta 2012, 75, 35–41.
- Roushani, M.; Shahdost-fard, F. A Novel Ultrasensitive Aptasensor Based on Silver Nanoparticles Measured Via Enhanced Voltammetric Response of Electrochemical Reduction of Riboflavin As Redox Probe for Cocaine Detection. Sensors and Actuators B: Chemical 2015, 207(Part A), 764–771.
- Ly, S.Y.; Yoo, H.S.; Ahn, J.Y.; Nam, K.H. Pico Molar Assay of Riboflavin in Human Urine Using Voltammetry. Food Chemistry 2011, 127, 270–274.
- Wang, Y.; Zhuang, Q.; Ni, Y. Fabrication of Riboflavin Electrochemical Sensor Based on Homoadenine Single-Stranded DNA/Molybdenum Disulfide–Graphene Nanocomposite Modified Gold Electrode. Journal of Electroanalytical Chemistry 2015, 736, 47–54.
- Ahmadpour-Mobarakeh, L.; Nezamzadeh-Ejhieh, A. A Zeolite Modified Carbon Paste Electrode As Useful Sensor for Voltammetric Determination of Acetaminophen. Materials Science and Engineering 2015, 49, 493–439.
- Hashemi, H.S.; Nezamzadeh-Ejhieh, A.; Karimi-Shamsabadi, M. A Novel Cysteine Sensor Based on Modification of Carbon Paste Electrode by Fe(II)-Exchanged Zeolite X Nanoparticles. Materials Science and Engineering 2016, 58, 286–293.
- Khaloo, S.S.; Mozaffari, S.; Barekat, A.; Karimi, F. Fabrication of a Modified Electrode Based on Multi-Walled Carbon Nanotubes Decorated with Iron Oxide Nanoparticles for the Determination of Enrofloxacin. Micro and Nano Letters 2015, 10, 561–566.
- Wang, Y.; Zhuang, Q.; Ni, Y. Fabrication of Riboflavin Electrochemical Sensor Based on Homoadenine Single-Stranded DNA/Molybdenum Disulfide–Graphene Nanocomposite Modified Gold Electrode. Journal of Electroanalytical Chemistry 2015, 736, 47–54.
- Sá, É.S.; da Silva, P.S.; Jost, C.L.; Spinelli, A. Electrochemical Sensor Based on Bismuth-Film Electrode for Voltammetric Studies on Vitamin B2 (Riboflavin). Sensors and Actuators B: Chemical 2015, 209, 423–430.
- Nezamzadeh-Ejhieh, A.; Pouladsaz, P. Voltammetric Determination of Riboflavin Based on Electrocatalytic Oxidation at Zeolite-Modified Carbon Paste Electrodes. Journal of Industrial and Engineering Chemistry 2014, 20, 2146–2152.
- Safari, N.; Notash, B.; Mohammad Nezhad, J.; Chiniforoshan, H.; Hadadzadeh, H.; Rezvani, A.R. Synthesis and Characterization of Tetraphenylporphyrin Manganese(III) Complexes of Phenylcyanamide Ligands. Inorganica Chimica Acta 2005, 358, 2967–2974.
- Kargar, H.; Afkhami, S.; Alikhani, F. Green Oxidation of 2-Imidazolines with Tert-Butyl Hydroperoxide Catalyzed by Supported Manganese(III) Porphyrin in Water. Journal of Coordination Chemistry 2012, 65, 3502–3510.
- Amini, M.K.; Khorasani, J.H.; Khaloo, S.S.; Tangestaninejad, S. Cobalt(II) Salophen-Modified Carbon-Paste Electrode for Potentiometric and Voltammetric Determination of Cysteine. Analytical Biochemistry 2003, 320, 32–38.
- Khaloo, S.S.; Amini, M.K.; Tangestaninejad, S.; Shahrokhian, S.; Kia, R. Voltammetric and Potentiometric Study of Cysteine at Cobalt(II) Phthalocyanine Modified Carbon-Paste Electrode. Journal of the Iranian Chemical Society 2004, 1, 128–135.
- Zhang, Z.; Wang, E. Electrochemical Principles and Methods; Science Press: Beijing, 2000.
- Kotkar, R.M.; Desai, P.B.; Srivastava, A.K. Behavior of Riboflavin on Plain Carbon Paste and Aza Macrocycles Based Chemically Modified Electrodes. Sensors and Actuators B: Chemical 2007, 124, 90–98.