Abstract
Solubility and quality of actomyosin play crucial roles during storage and processing of a particular muscle type. We report here the effect of low intensity (20 kHz) ultrasonication on the solubility and some other biochemical properties of the actomyosin isolated from chicken breast muscle. Although there was an overall enhancement in the solubility of actomyosin during sonication, the major increase of ~61.55% occurred at 0.2M NaCl after 10–12 min of exposure. The distinctive feature of sodium dodecyl sulphate-polyacrylamide gel electrophoretic (SDS-PAGE) profiles of this fraction was the presence of free or non-interacting actin in considerable amount. Even at higher salt concentrations (0.4 and 0.5M NaCl), the protein solubility was almost twice as high as that of the corresponding controls. At low salt concentrations (<0.3 M NaCl or KCl) where the protein solubility was high, Ca2+-, K+(EDTA)- and Mg2+-ATPase activities were low. However, a remarkable increase in each of the enzymatic activities occurred at the salt concentrations higher than 0.3 M. More importantly, the high level of Mg2+-ATPase strongly indicated that actomyosin was reconstituted. The ultraviolet spectra also supported the reconstitution of actomyosin at high salt concentrations. These data suggested that ultrasonication caused conformational changes which modified properties of myosin and actin affecting the intermolecular relationships within actomyosin complex. The shift in the solubility and functional modifications of sonicated actomyosin provide some basis to explain the processing behavior of sonicated poultry meat. In addition, the low frequency sonication may be suggested as a non-destructive method to compare actomyosin extracted from various sources.
INTRODUCTION
The changes in biochemical properties of actomyosin (AM) have been extensively used to explain storage and processing behavior of poultry and other types of meats.[Citation1–Citation5] AM constitutes bulk of the myofibrillar protein mass while retaining functional characteristics of myofibrillar contractile apparatus. For biochemical investigations, skeletal AM has been routinely extracted and dissolved in high salt molarity solutions (~0.6 M NaCl or KCl).[Citation6,Citation7] Attempts to solubilize myosin and associated proteins of the contractile apparatus in millimolar salt solutions have also been successful.[Citation8,Citation9] To achieve maximum solubility, low frequency (20 kHz) ultrasonic disruption of myofibrillar structure was considered essential by some investigators.[Citation10] However, low ionic strength or water soluble myofibrillar protein extracts are suitable as protein supplements only, because they lack functionality.
In vitro studies have established that it was isolated AM that simulated heat gelation behavior of a particular muscle.[Citation1–Citation6] The available literature also revealed that quantity as well as the quality of AM were crucial in forming gels of desirable viscoelastic properties.[Citation6,Citation11] Thus, high salt contents and the solubilization of AM are obligatory for converting comminuted meat to heat processed products.[Citation12–Citation15] Ultrasonication has been reported to improve processing properties of meat such as the tenderness, salt diffusion and binding strength of thermal gel matrices.[Citation16–Citation18]
These observations underlined the importance of basic information on the sonication-solubilized proteins of AM complex. So far, only some preliminary observations have been published which dealt with low intensity sonication of unpartitioned AM.[Citation6] Therefore, we have investigated the biochemical changes which accompanied the low frequency (20 kHz, 50 W) sonication of AM isolated from chicken breast muscle. We have also monitored variations of the investigated properties in low salt dilutions of the sonicated AM. Compared to myofibrillar suspension, isolated AM complex represents a soluble and cell free system which permits ultraviolet (UV) spectroscopy.[Citation14]
MATERIALS AND METHODS
Chemicals
Acrylamide, bis-acrylamide, phenyl methane sulfonylfluoride (PMSF), adenosine 5ʹ-triphosphate disodium salt (ATP), ammonium per-sulfate and tetramethylethylene diamine (TEMED) were procured from authorized dealers of Sigma-Aldrich chemicals Pvt. Ltd. Potassium chloride, bovine serum albumin, tris buffer, and 1-amino-2-naphthol-4-sulphonic acid and chemicals or reagents of analytical grade were purchased from SRL, India.
Preparation of AM
Birds (3 months old broiler chicken) were sacrificed with standard procedures of exsanguinations.[19] There was no pooling of AM preparations. Excised breast (pectoralis major) muscles were immediately immersed in crushed ice bath. AM was prepared as previously described[Citation20,Citation21] with some modifications: prior to overnight extraction of muscle mince in the extraction buffer (0.6 M KCl in 25 mM Tris-maleate buffer of pH 7.0, containing 2 mM PMSF), it was washed thrice with 50 mM phosphate buffer (pH 7.2). The thick viscous solution of natural AM was recovered after centrifugation at 10 K rpm (4°C) for 15 min. AM was precipitated by 10-fold dilution with chilled distilled water and collected by centrifugation at 5 K rpm for 15 min (4°C). The precipitate was saved and dissolved in 0.6 M NaCl with 20 mM Tris-maleate buffer, pH 7.2 (dissolving buffer). Traces of free myosin were removed by two cycles of precipitation of 0.6 M AM solution at 0.2 M NaCl. Then, AM solution in 0.6 M NaCl was passed through one more cycle of 10× dilution before dissolving finally in 0.6 M NaCl buffer. Overnight dialysis against the dissolving buffer followed which contained no PMSF.
Protein Estimation
Protein concentrations of all the samples were determined by the method of Lowry et al.,[Citation22] using bovine serum albumin as standard. Optical density was recorded at 660 nm on BioSyn UV-Visible spectrophotometer. All the values are average of three different dilutions of each protein sample.
Ultrasonic Treatment
Solutions of 2.5–3 mg/mL AM in 10 mL of dissolving buffer were exposed to low frequency (20 kHz, 50 W) ultrasonic waves for 30 min.[Citation7] Immersion-probe type ultrasonicator (Ralco, India Ltd.) was used throughout the study. The probe of the sonicator was immersed in the middle of AM container and held in a central position. During ultrasonication, sample tubes were kept under ice bath and each sonication burst of 15 s was followed by 10 s of cooling lag to offset heating. Five aliquots of equal volume (2.0 mL) were collected at intervals of 5, 10, 15, 20, and 30 min.
Preparation of Salt Soluble Fractions of Sonicated AM at Various NaCl Dilutions
Sonicated aliquots of AM dissolved in 0.6 M NaCl (20 mM tris-maleate) and collected at specific time intervals were diluted to 0.1, 0.2, 0.3, 0.4, and 0.5 M NaCl. The diluted samples were fractionated into supernatants (soluble fraction) and pellets (insoluble fraction) following centrifugation at 10 K rpm for 15 min. The soluble fractions were saved for studying various biochemical parameters. The solubility at a given dilution was expressed as the ratio of protein content in supernatant to total protein and was calculated using the following formula:
Assay of ATPases
ATPase activities of the control as well as sonicated AM were assayed following the standard protocol.[Citation20,Citation21] Ca2+ or Mg2+ ion activated ATPase was assayed at 20ºC in final concentrations of 50 mM KCl, 20 mM Tris-maleate of pH 7.0, and 5 mM CaCl2 (or Mg2+). Exclusively for K+(EDTA)-ATPase assay, NaCl was removed by two cycles of precipitation and AM samples were then dissolved in 0.6 M KCl. The final concentration of KCl in K+(EDTA)-ATPase assay mixture was maintained at 0.6 M KCl by adding the required amount of KCl stock solution to dilutions of AM where KCl concentration was below 0.6 M.[Citation23] In all the assays, the reaction was started with the addition of ATP (1.0 mM) and stopped by perchloric acid (15%) after incubating for 2 and 4 min. To quantitate inorganic phosphate (Pi) liberated during ATP hydrolysis, we followed the method of Fiske and Subbarow.[Citation24] The Pi data was plotted using the average of 2 and 4 min readings.
UV Absorption Spectra
UV absorption spectra of various AM samples were taken at a constant protein concentration of 0.2 mg/mL on GENESYS 10 UV scanning spectrophotometer. NaCl solutions of corresponding strength were used as blanks.
Sodium Dodecyl Sulphate-Polyacrylamide Gel Electrophoresis (SDS-PAGE) Profiling of Sonicated AM Dilutions and Documentation
We followed the sodium dodecyl sulphate-polyacrylamide gel electrophoresis (SDS-PAGE) protocol of Laemmli[Citation25] to monitor polypeptide abundance, relative intensities, and stoichiometry of individual polypeptides in the soluble fractions of ultrasonicated AM, with a few minor modifications reported previously.[Citation20,Citation21] Gels (10%) were run initially at 5 mA until samples entered separating gel, and 15 mA thereafter. Gels were fixed using a mixture of methanol (40%) and acetic acid (10%) for 2 h at 25°C that was also used as washing solution to remove SDS. Subsequently, gels were stained with Coomassie brilliant blue R-250 (CBBR-250; 0.25% w/v) in 45% methanol and 10% acetic acid. Excess stain was removed by incubating gels overnight in 7% acetic acid. Finally, the gels were photographed or directly scanned on document scanner (HP Deskjet F370).
Densitometry and Quantitative Assessment of SDS-PAGE Profiles
Data from digital photographs and direct gel-scans were compared and various lanes were selected for densitometric analysis through Scion Imaging (Scion Corporation; Beta release: 4.0) software. Gel Pro software (Cybernetics, USA) was used for quantitative analysis of various lanes. Each value of the data obtained by Scion imaging software is expressed as integrated pixel density value (IDV). Based on these values expected actin was calculated using the formula:
Statistical Analysis
Up to 25 birds were sacrificed to investigate one or the other biochemical property. However, the data discussed here is the average of duplicate determinations made on different AM preparations, which were also used for UV spectroscopy. The differences in the obtained values of control and sonicated samples of every category (n = 5) were tested through student’s t-test and the values were considered significant at p < 0.05.
RESULTS AND DISCUSSION
Time-Dependent Solubility of Sonicated-AM
The time-dependent solubility profiles followed the biphasic kinetics. A rapid enhancement in protein solubility occurred during the first 10–12 min of sonication (). Subsequent enhancement in the solubility was modest until the 30th min. The deceleration in protein solubility during this (second) phase suggested that >12 min of low frequency sonication cause some denaturation of soluble AM at >0.3 M NaCl.
FIGURE 1 (a) Time course of changes in protein solubility of sonicated AM as 0.6M NaCl solution after post-exposure dilutions to different NaCl molarities. Starting point (zero) of each curve is the protein concentration of control supernatant at each NaCl dilution. (n = 5; p < 0.05; SE ± 2–5); (b) Salt concentration-dependence of sonicated-AM solubility (continuous line) showing shift to lower NaCl molarity. Opposite behavior of solubility displayed by unsonicated AM at different dilutions of NaCl is displayed as dotted line. (n = 5; p < 0.05; SE ± 2–5).
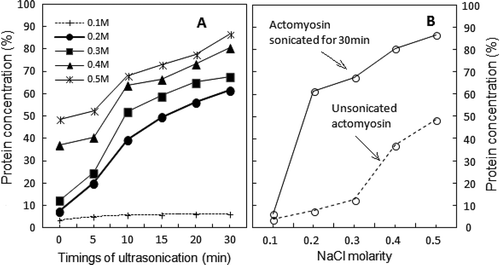
Salt Concentration-Dependent Solubility of Sonicated-AM
When AM was subjected to 30 min long sonication, barely 6.35% of the protein was soluble at 10 mM NaCl dilution. As shown in , the dilution to 0.1 M NaCl precipitated most of the sonicated AM (~94%). In precipitated AM, actin and myosin are in a strongly bound crystallized state which is morphologically different from the arrowhead structure of actin-myosin filaments in situ.[Citation26] We have recently shown that low frequency (20 kHz) sonication of precipitated AM for 30 min solubilizes only 13% of protein.[Citation27] The least solubility of AM at 0.1 M NaCl is also supported by SDS-PAGE profiles of soluble polypeptides of sonicated AM at 0.1 M NaCl dilution.
The solubility gradients of sonicated AM and the corresponding unsonicated controls were just opposite to each other (). Whereas only 6.35% of sonicated AM was soluble at 0.1 M NaCl, a sharp enhancement of 61.68% was observed at 0.2 M NaCl (). In comparison, corresponding values at these NaCl molarities were 3.75 and 7.54%, respectively. Since the difference between solubility of sonicated AM and its corresponding control was minimal at 0.2 M NaCl, it can be taken as the lowest concentration at which sonication exclusively solubilized the highest amount of AM. At further high concentrations of NaCl, the enhancement in solubility of sonicated AM was modest, as evident from the values of 67.68 and 86.66% at 0.3 and 0.5 M NaCl, respectively. In contrast, the corresponding values for AM controls were 37.2 and 48.54%, respectively. In any case, the solubility of sonicated AM is almost twice that of the controls. As indicated by the low levels of Mg2+-ATPase activities at low salt concentrations (explained below under suitable subhead), the maximum solubility of sonicated AM at 0.2 M NaCl was due to “weak actin-myosin interaction.”
Stoichiometry of Myosin Heavy Chain (MyHC) and Actin Binding
According to IDV, the two remarkable features of the dynamics of polypeptide abundance in 0.2 M NaCl solution of sonicated AM were: A sudden rise in MyHC contents after 15 min of sonication; and the presence of free actin (non-binding to MyHC). The standard stoichiometric binding ratio of actin: MyHC as calculated from the IDV of respective bands in SDS-PAGE profiles of Srf is ~0.62 (lane Srf in ). This ratio is in agreement with the reported values of vertebrate skeletal muscle AM.[Citation28,Citation29] According to standard stoichiometry of actin-MyHC binding, the IDV of actin band after 15 min of sonication had to ascended parallel with the IDV of MyHC (). However, the observed IDV of actin band at 0.2 M NaCl deviated from this “expected trend of stoichiometry,” as free or non-interacting actin was present in excess of MyHC-binding requirement (). In comparison, the IDV of actin band was proportionate to actin-MyHC binding at 0.3 M (). According to the IDV data, free actin was again detected at 0.4 M and 0.5 M NaCl, though in rather low amounts (). Dissociation of actin-myosin at 0.2 M NaCl, partially explains excess of non-interacting actin. One of the strong possibilities is a more rapid denaturation of myosin, although actin molecule also fragments and repolymerize under influence of ultrasonication.[Citation30,Citation31]
FIGURE 2 SDS-PAGE profiles of proteins in sonication solubilised AM at various NaCl dilutions (Srf). Equal volume (10 µL) of each sample was loaded in individual pocket. Notation sequence below the lanes 1-2 shows: Srf = Standard reference (0.6 M NAM); C = Supernatant of unsonicated control at corresponding dilution. Subsequent notations (5, 10, 15, 20, and 30) indicate time in minutes at which supernatants were obtained. MyHC = Myosin heavy chain (200 kDa); Actin (46 kDa); Tm (Tropomyosin) = 37; Tn T (Troponin T) = 35; Tn I (Troponin I) = 24; Tn C (Troponin C) = 20; MLC I (Myosin light chain I) = 26; MLC II = 18; MLC III = 16.
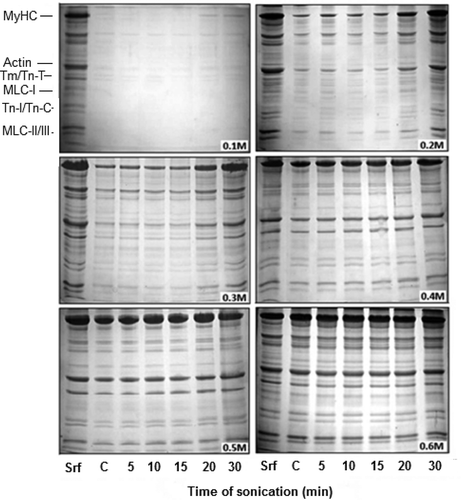
FIGURE 3 Densitometric analysis of selected SDS-PAGE profiles of supernatants from 0.2 to 0.5 M dilutions of sonicated-AM, showing change in of MyHC and Actin with the increasing time of sonication (x-axis). Out of each set of bars, 1st, 2nd, and 3rd bar represent IDV of MyHC, expected IDV of actin (calculated according to formula given under “Materials and Methods”) and actually observed IDV of actin, respectively (±1–4%).
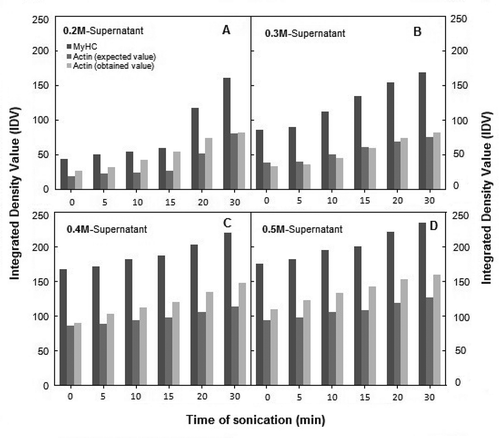
Changes in ATPase Activities
During exposure to high frequency ultrasonication, Ca2+-ATPase activity has been reported to decline more rapidly than the rate of AM reconstitution.[Citation32] Our preliminary study also showed that with the increasing time of low frequency sonication, Ca2+-ATPase activity of unpartitioned and undiluted AM declined accordingly.[Citation7] To date, no data on K+(EDTA)-ATPase activity, the other index of the status or integrity of myosin/MyHC,[Citation33,Citation34] has been reported. However, sonic treatment exceeding 10 min in duration positively modulated Ca2+- and K+(EDTA)-ATPase activities (of soluble fraction) at >0.3 M NaCl/KCl in a time-dependent manner (). The profiles of both of the enzymatic activities myosin/MyHC in reconstituted sonicated AM appeared lower than those of the Srf.
FIGURE 5 Exposure time dependence of ultrasonication-induced changes in Ca2+-ATPase and K+ (EDTA)-ATPase activities recorded for soluble fractions of actomyosin at various NaCl dilutions (frames a and b). Changes in Mg2+-ATPase activity are compared in frames c–d. Symbols for individual ATPase activity are given with notations in each frame. To facilitate comparison, ATPase activities were converted to percent of respective controls of the same dilutions of chicken AM (±3–10%).
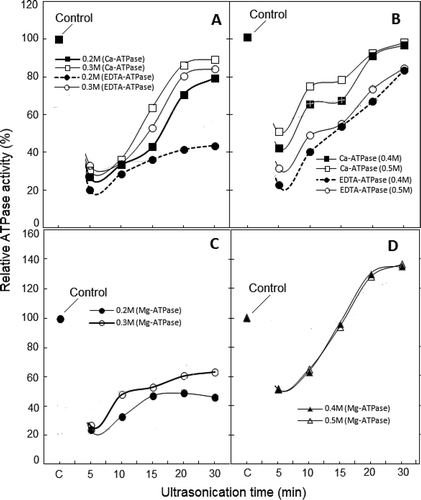
Although, the magnitude of positive modulation of Ca2+- and K+(EDTA)-ATPase activities at 0.4 and 0.5 M NaCl is close to the values of corresponding controls, it is still ~30% lower than the activities of 0.5M NaCl dilution of Srf (unsonicated AM in 0.6 M NaCl). Therefore, the enzymatic characteristics of myosin/MyHC in reconstituted sonicated AM demonstrate partial inactivation of the two ATPases. Similarly, despite the near normal ratio of actin-MyHC bands at 0.3 M NaCl ( and ), Mg2+-ATPase is lower than the activity noted at 0.5 M NaCl dilution of Srf ( and ). Apparently, the observed decline in various ATPase activities is the consequence of sonication-induced conformational changes which may include some aggregation of myosin.
ATP binding site is located at the apex of the cleft which separates the two 50 kDa actin binding domains of myosin subfragment-1.[Citation35,Citation36] Our results suggest that low intensity sonication might have affected actin binding domains as well as the ATP binding site. This is supported by the changes in profiles and magnitudes of Ca2+- and K+(EDTA)-ATPase activities and actin-myosin interaction indicator, Mg2+-ATPase.
Spectral Analyses
Recently, UV spectra of AM at low protein concentrations have been used to obtain information on heat denaturation related changes.[Citation14] Unlike heat treatment which increases turbidity,[Citation14,Citation19,Citation34] ultrasonication has the advantage of increasing transparency of exposed AM solutions.[Citation7] Spectra of chicken AM differed from profile of invertebrate AM isolated from green mussel which exhibits maximum at 270–280 nm.[Citation37]
Changes in UV spectra of sonicated AM are compared in . At each NaCl dilution, perturbation at wavelengths (247–299 nm) was recorded, which suggested involvement of tyrosyl, tryptophanyl, and phenyl-alanyl residues from 5 min of ultrasonication onward ( and ). Thus, sonication affected hydrophobic regions of myosin and actin molecules, the two major proteins of AM which essentially determine the functionality and solubility of AM complex. The maximum impact of sonication was observed at 15 min with >0.3 M NaCl, when sonicated AM is either partially or substantially solubilized (–).
FIGURE 6 UV spectra of soluble fractions obtained at 0.2–0.6 M NaCl dilutions of ultrasonicated 0.6 M NaCl-soluble AM, taken after various exposure timings. Thick continuous line represents 15 min supernatant, where ~286 nm maximum assumes shape of peak beyond 0.3 M NaCl. Spectra in frame d represent unpartitioned sonicated AM soluble in 0.6 M NaCl.
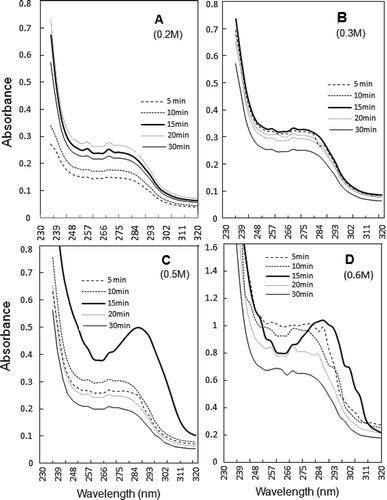
FIGURE 7 Comparison of sonic exposure and salt-dependence of the peak absorbance values at ~257 and ~286 nm of UV spectra, shown in .
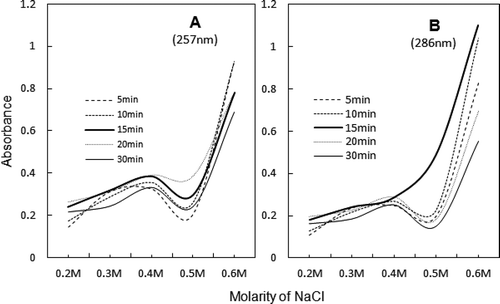
The close resemblance between peak values of aromatic residues at 257 and 286 nm is displayed in . In general, O.D. values are high from 0.3 to 0.4 M NaCl which is also the salt concentration range for AM reconstitution and heat induced gelation ().[Citation1,Citation11] The critical timing and dilution, at which various spectra closely resemble the spectrum of Srf, are 15 min and 0.5 M NaCl, respectively ( and ).
Collectively, the evidence presented here suggested that ultrasonication-induced changes in AM are the mainly expression of “conformational state of the myosin molecule” and “altered actin-myosin interaction.” As already pointed out, the lower Ca2+- and K+(EDTA)-ATPase activities indicate a less active conformation of myosin. A correlation with myosin light chains (LCs) is likely, since SDS-PAGE profiles indicate slight change in the relative intensities of these polypeptides. LC2 has a crucial role in filament assembly and functional states of myosin heads.[Citation36–Citation39] The observation is supported by our study on low salt precipitated AM, which showed a quantitative increase in LCs that corresponded with the increasing duration of sonication.[Citation21]
A number of reviews have consolidated the published information on the applications and consequences of ultrasonic exposure of various meats including that of the chicken.[Citation17,Citation40,Citation42–Citation43] The present investigations provided information on the properties of sonicated but unfragmented myosin/MyHC soluble at NaCl concentrations lower than 0.6 M. We ensured inactivation of endoproteases by adding PMSF during washing the muscle mince and overnight extraction of AM. Therefore, the low frequency sonic waves can be used to defrost meat[Citation44] without significantly fragmenting myofibrillar proteins. More importantly, the data demonstrates that the proteins of sonicated AM complex are more soluble from 0.2–0.3 M NaCl and have a different conformation from unsonicated AM. The sonication-induced changes in the properties of AM observed in this study support the possible reduction in salt solubility requirement of myofibrillar proteins during processing, without affecting thermal gel formability.[Citation18] However, more comprehensive information on sonication of myofibrillar proteins is needed to establish an unambiguous correlation with the qualitative attributes of poultry meat.
CONCLUSIONS
Low frequency (20 kHz) ultrasonication of broiler chicken AM solution enhanced its solubility at NaCl concentrations lesser than 0.6 M. Functionality of sonicated AM was governed by “dissociation” or “reconstitution” kinetics of AM in an exposure-time and salt-dependence manner. Since at this frequency no key proteins of AM fragmented, the low frequency sonication can defrost meat without significantly fragmenting myofibrillar proteins. More than 10 min of sonication caused some denaturation irrespective of reconstitution of AM at >0.3 M NaCl. Relatively low recovery of myosin ATPases and UV spectra supported a relatively less active conformation of myosin/MyHC involving hydrophobic interactions. Mg2+-ATPase indicated that actin-myosin binding is reasonably strong at about 2% (~0.4 M) NaCl. Therefore, sonication can help to reduce salt requirements of processing of comminute meat.
ACKNOWLEDGMENTS
The authors are grateful to the Aligarh Muslim University and the Chairman, Department of Zoology for providing facilities. The authors are thankful for the help provided by Prof. S. M. Abbas Abidi during the experimental work. The authors are thankful for the cooperation of Miss Areeba Ahmad and other laboratory colleagues deserves special mention. We are also thankful to Dr. S. Arif Abdul Rahman and Dr. Thomas McWilliams of MRC, College of Life Sciences at University of Dundee (UK) for useful discussions and language revisions.
FUNDING
The work was carried out of the financial assistance from MRP by the UGC, New Delhi [F. No. 39-581/2010 (SR)] to AH/RA and from UGC-Non-NET Fellowship to RS.
Additional information
Funding
REFERENCES
- Asghar, A.; Samejima, K.; Yasui, T.; Henrickson, R.L. Functionality of Muscle Proteins in Gelation Mechanisms of Structured Meat Products. Critical Reviews in Food Science & Nutrition 1985, 22 (1), 27–106.
- Xiong, Y.L.; Brekke, C.J. Changes in Protein Solubility and Gelation Properties of Chicken Myofibrils During Storage. Journal of Food Science 1989, 5 (5), 1141–1146.
- Samejima, K.; Lee, N.H.; Ishioroshi, M.; Asghar, A. Protein Extractability and Thermal Gel Formability of Myofibrils Isolated from Skeletal and Cardiac Muscles at Different Post‐Mortem Periods. Journal of the Science of Food and Agriculture 1992, 58 (3), 385–393.
- Xiong, Y.L.; Blanchard, S.P. Dynamic Gelling Properties of Myofibrillar Protein from Skeletal Chicken Parts. Journal of Agricultural and Food Chemistry 1994, 42, 670–674.
- Kang, G.H.; Park, G.B.; Joo, S.T.; Lee, M.; Lee, S.K. Effects of Muscle Fiber Types on Gel Property of Surimi‐Like Materials from Chicken, Pork, and Beef. Journal of Muscle Foods 2010, 21 (3), 570–584.
- Yasui, T.; Ishioroshi, M.; Samejima, K. Effect of Actomyosin on Heat Induced Gelation of Myosin. Agricultural and Biological Chemistry 1982, 46, 1049–1059.
- Ahmad, R.; Hasnain, A. Ultrasonication of Chicken Natural Actomyosin: Effect on AtPASE Activity, Turbidity, and SDS-PAGE Profiles at Different Protein Concentrations. American Journal of Biochemistry and Molecular Biology 2013, 3 (2), 240–247.
- Krishnamurthy, G.; Chang, H.S.; Hultin, H.O.; Feng, Y.; Srinivasan, S.; Kelleher, S.D. Solubility of Chicken Breast Muscle Proteins in Solutions of Low Ionic Strength. Journal of Agricultural and Food Chemistry 1996, 44 (2), 408–415.
- Feng, Y.; Hultin, H.O. Solubility of the Proteins of Mackerel Light Muscle at Low Ionic Strength. Journal of Food Biochemistry 1997, 21 (1), 479–496.
- Ito, Y.; Toki, S.; Omori, T.; Ide, H.; Tatsumi, R.; Wakamatsu, J.I.; Nishimura, T.; Hattori, A. Physicochemical Properties of Water-Soluble Myofibrillar Proteins Prepared from Chicken Breast Muscle. Animal Science Journal 2004, 75, 59–65.
- Wang, S.F.; Smith, D.M. Gelation of Chicken Breast Muscle Actomyosin As Influenced by Weight Ratio of Actin to Myosin. Journal of Agricultural Food Chemistry 1995, 43, 331–336.
- Barbut, S.; Mittal, G.S. Effects of Salt Reduction on the Rheological and Gelation Properties of Beef, Pork, and Poultry Meat Batters. Meat Science 1989, 26, 177–191.
- Liu, H.; Gao, L.; Ren, Y.; Zhao, Q. Chemical Interactions and Protein Conformation Changes During Silver Carp (Hypophthalmichthys Molitrix) Surimi Gel Formation. International Journal of Food Properties 2014, 17 (8), 1702–1713.
- Liu, R.; Zhao, S.; Yang, H.; Li, D.; Xiong, S.; Xie, B. Comparative Study on the Stability of Fish Actomyosin and Pork Actomyosin. Meat Science 2011, 88 (2), 234–240.
- You, J.; Pan, J.; Shen, H.; Luo, Y. Changes in Physicochemical Properties of Bighead Carp (Aristichthysmobilis) Actomyosin by Thermal Treatment. International Journal of Food Properties 2012, 15 (6), 1276–1285.
- Stadnik, J.; Dolatowski, Z.J.; Baranowska, H.M. Effect of Ultrasound Treatment on Water Holding Properties and Microstructure of Beef (M. Semimembranosus) During Ageing. LWT-Food Science and Technology 2008, 41, 2151–2158.
- Xiong, G.Y.; Zhang, L.L.; Zhang, W.; Wu, J. Influence of Ultrasound and Proteolytic Enzyme Inhibitors on Muscle Degradation, Tenderness, and Cooking Loss of Hens During Aging. Czech Journal of Food Science 2012, 30, 195–205.
- McDonnel, C.K.; Allen, P; Morin, C.; Lyng, J.G. The Effect of Ultrasonic Salting on Protein and Water-Protein Interactions in Meat. Food Chemistry 2014, 147, 245–251.
- Nawsad, A.A.; Kanoh, S.; Niwa, E. Thermal Gelation Properties of Spent Hen Mince Surimi. Poultry Science 2000, 79, 117–125.
- Ahmad, R.; Hasnain, A. Correlation Between Biochemical Properties and Adaptive Diversity of Skeletal Muscle Myofibrils and Myosin of Some Air-Breathing Teleost. Indian Journal of Biochemistry and Biophysics 2006, 43, 217–225.
- Saleem, R.; Hasnain, A.; Ahmad, R. Changes in Some Biochemical Indices of Stability of Broiler Chicken Actomyosin at Different Levels of Sodium Bicarbonate in Presence and Absence of Sodium Chloride. International Journal of Food Properties 2015, 18 (6), 1373–1384.
- Lowry, O.H.; Rosebrough, N.J.; Farr, A.L.; Randall, R.J. Protein Measurement with the Folin Phenol Reagent. Journal of Biological Chemistry 1951, 193, 265–275.
- Hasnain, A.; Ahmad, R. A Study on Some Biochemical Properties of Active Subfragment-1 (S1) Prepared from Actomyosin of Air-Breathing Freshwater Teleost, Channa Gachua. Biomedical Research 2006, 17, 27–31.
- Fiske, C.H.; Subbarow, Y. The Colorimetric Determination of Phosphorus. Journal of Biological Chemistry 1925, 66 (2), 375–400.
- Laemmli, U.K. Cleavage of Structural Proteins During the Assembly of the Head of Bacteriophage T4. Nature 1970, 227, 680–685.
- Thomas, D.; Pollard, R.; Weihing, R.; Adelman, M.R. Actin and Myosin and Cell Movement. Critical Reviews in Biochemistry 1974, 2 (1), 1–65.
- Saleem, R.; Hasnain, A.; Ahmad, R. Solubilization of Muscle Proteins from Chicken Breast Muscles by Ultrasonic Radiation in Physiological Ionic Medium. Cogent Food & Agriculture 2015, 1 (1),1046716. DOI:10.1080/23311932.2015.1046716
- Jakubiec-Puka, A.; Szczepanowska, J.; Wieczorek, U. Myosin Heavy Chains in Striated Muscle Immobilized in a Shortened Position. Basic and Applied Myology 1995, 5 (2), 147–153.
- Hrynets, Y.; Ndagijimana, M.; Betti, M. Non-Enzymatic Glycation of Natural Actomyosin (NAM) with Glucosamine in a Liquid System at Moderate Temperatures. Food Chemistry 2013, 139 (1), 1062–1072.
- Asakura, S.; Taniguch, M; Osawa, F. Mechanochemical Behavior of F-Actin. Journal of Molecular Biololgy 1963, 7, 55–69.
- Carlier, M.F.; Pantaloni, D.; Korn, E.D. Polymerization of ADP-Actin and ATP-Actin Under Sonication and Characteristics of the ATP-Actin Equilibrium Polymer. Journal of Biological Chemistry 1985, 260, 6565–6571.
- Bárány, M.; Bárány, K.; Oppenheimer, H. Effect of Ultrasonics on the Adenosine Triphosphatase Activity and Actin-Binding Ability of L-Myosin and Heavy Meromyosin. Nature 1963, 199, 694–695.
- Iwamoto, H. Influence of Ionic Strength on the Actomyosin Reaction Steps in Contracting Skeletal Muscle Fibers. Biophysical Journal 2000, 78 (6), 3138–3149.
- Kanaya, H.; Hara, K.; Okabe, H.; Matsushige, K.; Nishimuta, S.; Muguruma, M.; Fukazawa, T. Observation of Transmitted Light Spectra During Gelation Process of Actomyosin. Journal of the Physical Society of Japan 1992, 61 (3), 1113–1118.
- Ahmad, R.; Hasnain, A. Peptide Mapping of Polymorphic Myosin Heavy Chain Isoforms in Different Muscle Types of Some Freshwater Teleosts. Fish Physiology and Biochemistry 2013, 39, 721–731.
- Wick, M.A. Filament Assembly Properties of the Sarcomeric Myosin Heavy Chain. Poultry Science 1999, 78 (5), 735–742.
- Geeves, M.A.; Holmes, K.C. The Molecular Mechanism of Muscle Contraction. Advances in Protein Chemistry 2005, 71, 161–194.
- Ryder, J.W.; Lau, K.S.; Kamm, K.E.; Stull, J.T. Enhanced Skeletal Muscle Contraction with Myosin Light Chain Phosphorylation by a Calmodulin-Sensing Kinase. Journal of Biological Chemistry 2007, 282 (28), 20447–20454.
- Shamasundar, B.A.; Dileep, A.O. Some Physico-Chemical, Functional, and Rheological Properties of Actomyosin from Green Mussel (Pernaviridis). Food Research International 2006, 39, 992–1001.
- Jayasooriya, S.D.; Bhandari, B.R.; Torley, P.; D’Arcy, B.R. Effect of High Power Ultrasound Waves on Properties of Meat: A Review. International Journal of Food Properties 2004, 7 (2), 301–319.
- Dolatowski, Z.J.; Stadnik, J.; Stasiak, D. Applications of Ultrasound in Food Technology. Acta Scientiarum Polonorum Technologia Alimentaria 2007, 6 (3), 89−99.
- Chemat, F.; Zill-e-Huma; Khan, M.K. Applications of Ultrasound in Food Technology: Processing, Preservation and Extraction. Ultrasonication and Sonochemistry 2011, 18, 813–835.
- Alaa El-Din, A.B.; Carne, A.; Ha, M.; Franks, P. Physical Interventions to Manipulate Texture and Tenderness of Fresh Meat: A Review. International Journal of Food Properties 2014, 17, 433–453.
- Malainine, M.O.; Faiz, B.; Moudden, A.; Izbaim, D.; Maze, G.; Aboudaoud, I. Beginning of Fish Defrosting by Using Non-Destructive Ultrasonic Technique. In IOP Conference Series: Materials Science and Engineering 2012, 42 (1), DOI:10.1088/1757-899X/42/1/012036.